Geochronology/Stratigraphy

Stratigraphy is concerned with the order and relative position of strata and their relationship to the geological time scale.
The image at the right shows rock strata in Cafayate, Argentina, the subject of stratigraphy.
Theoretical stratigraphy
[edit | edit source]

Def. the "study of rock layers and the layering process"[1] is called stratigraphy.
Smith's first law, the law of superposition states: in an undeformed stratigraphic sequence, the oldest strata occur at the base of the sequence, an object cannot be older than the materials of which it is composed.[2]
Newer rock beds lie on top of older rock beds unless the succession has been overturned.
Since there is no overturning, the rock at the bottom in the image on the right is older than the rock on the top by the Law of Superposition.
Smith's second law, the Law of Strata identified by fossils states that each stratum in the succession contains a distinctive set of fossils, which allows beds to be identified as belonging to the same stratum even when the horizon between them is not continuous.[3]
The Principle of Original Horizontality states that layers of sediment are originally deposited horizontally under the action of gravity.[4]
In the second image down on the right, the sediments composing these rocks were formed in an ocean and deposited in horizontal layers.
In the image on the left, these strata make up much of the famous prominent rock formations in widely spaced protected areas such as Capitol Reef National Park and Canyonlands National Park. From top to bottom: Rounded tan domes of the Navajo Sandstone, layered red Kayenta Formation, cliff-forming, vertically jointed, red Wingate Sandstone, slope-forming, purplish Chinle Formation, layered, lighter-red Moenkopi Formation, and white, layered Cutler Formation sandstone. Picture from Glen Canyon National Recreation Area, Utah.
The principle of lateral continuity states that layers of sediment initially extend laterally in all directions; in other words, they are laterally continuous.[5] As a result, rocks that are otherwise similar, but are now separated by a valley or other erosional feature, can be assumed to be originally continuous.
Layers of sediment do not extend indefinitely; rather, the limits can be recognized and are controlled by the amount and type of sediment available and the size and shape of the sedimentary basin. As long as sediment is transported to an area, it will eventually be deposited. However, as the amount of material lessens away from the source, the layer of that material will become thinner.
Often, coarser-grained material can no longer be transported to an area because the transporting medium has insufficient energy to carry it to that location. In its place, the particles that settle from the transporting medium will be finer-grained, and there will be a lateral transition from coarser- to finer-grained material. The lateral variation in sediment within a stratum is known as sedimentary facies.
Cross-cutting relationships is a principle of geology that states that the geologic feature which cuts another is the younger of the two features:
- Structural relationships may be faults or fractures cutting through an older rock.
- Intrusional relationships occur when an igneous pluton or dike is intruded into pre-existing rocks.
- Stratigraphic relationships may be an erosional surface (or unconformity) cuts across older rock layers, geological structures, or other geological features.
- Sedimentological relationships occur where currents have eroded or scoured older sediment in a local area to produce, for example, a channel filled with sand.
- Paleontological relationships occur where animal activity or plant growth produces truncation. This happens, for example, where animal burrows penetrate into pre-existing sedimentary deposits.
- Geomorphological relationships may occur where a surficial feature, such as a river, flows through a gap in a ridge of rock. In a similar example, an impact crater excavates into a subsurface layer of rock.
Second down on the left, an igneous intrusion is cut by a pegmatite dyke, which in turn is cut by a dolerite dyke. These rocks are of Precambrian (Proterozoic) age and they are located in Kosterhavet National Park on Yttre Ursholmen island in the Koster Islands in Sweden. The oldest igneous rocks in this photo show features caused by magma mingling or magma mixing.
The law of included fragments states that clasts in a rock are older than the rock itself.[6] A xenolith, a fragment of country rock that fell into passing magma as a result of stoping, or a derived fossil, a fossil eroded from an older bed and redeposited into a younger one, are included fragments.[7]
Def. a "body of rock with specified characteristics reflecting the way it was formed"[8] is called a facies.
A facies is a body of rock with specified characteristics,[9] which can be any observable attribute of rocks (such as their overall appearance, composition, or condition of formation), and the changes that may occur in those attributes over a geographic area and is the sum total characteristics of a rock including its chemical, physical, and biological features that distinguishes it from adjacent rock.[10]
Law of Facies, or simply Walther's Law, states that the vertical succession of facies reflects lateral changes in environment; conversely, it states that when a depositional environment "migrates" laterally, sediments of one depositional environment come to lie on top of another.[11] In Russia the law is known as Golovkinsky-Walther's Law, "The fundamentals of the facies law, known in the West as Walther's Law and in Russia as Golovkinsky-Walther's Law, were also described in Golovkinsky's work long before Walther drew his conclusions on this subject. The present paper shows that the fundamentals of sequence stratigraphy were first set forth in the work of N. A. Golovkinsky."[12]
Chemostratigraphy
[edit | edit source]The changes in the relative proportions of trace elements and isotopes within and between lithologic units vary with time and can used to map subtle changes that occurred in the paleoenvironment.
Stratigraphy
[edit | edit source]Dates have been assigned to specific geologic stratigraphy frames, columns, or columnar units.
Stratigraphic columns
[edit | edit source]As an example of a stratigraphic column, the image at the right shows one for the Dinosaur National Monument, Utah and Colorado, USA.
Each geographic location on the rocky surface of the Earth has a stratigraphic column. Correlating each stratum that has been shown to be in a geologic time period with others around the world is part of the fun of stratigraphy.
Geography
[edit | edit source]At the right is a small portion of the stratigraphic column between the Hatherton and MacKay glaciers in Antarctica. The top rock layer is a greyish red siltstone. The next downward is a greenish grey siltstone penetrated by sinuous tubes that may be roots or root-like structures. Underlaying this is "a zone of calcareous nodules."[13]
"The Beacon Supergroup (Barrett, 1970) in the Transantarctic Mountains is largely a flat-lying, nonmarine sequence from Devonian or older to Jurassic in age. It consists of the Taylor Group (Devonian or older), a quartzose sandstone sequence, and the Victoria Group (Permian and Triassic), dominantly a coal-bearing sandstone-siltstone sequence (Harrington, 1965)."[13]
"The Taylor Group comprises up to 1,450 m of quartzose sandstone, with smaller conglomerate, arkosic and shaly units [...]. [The] youngest Taylor Group unit [is] the Aztec Siltstone [of which the image at the right exhibits]."[13]
Glacial sediment layers
[edit | edit source]
The cross section of a sandur deposit on the right from Iceland shows strata of various sand-like material atop dead ice from a former surge of the glacier Brúarjökull.
In the second image down on the right: "The pebble beach at Weybourne marks the start of the cliff section of the Norfolk coast that extends in easterly direction. This change in the character of the coastline is due to the properties of the chalk, which is harder to the east. The cliffs resting on this chalk base are composed of layers of glacial sediments of flints and fossils."[14]
Varves
[edit | edit source]
Def. "a pair of sedimentary layers, a couplet, that form in an annual cycle as the result of seasonal weather changes"[15] is called a varve.
"Typically formed in glacial lakes a varve couplet consists of a coarser grained summer layer formed during open-water conditions, and a finer grained winter layer formed from deposition from suspension during a period of winter ice cover. Many varve deposits contain hundreds of couplets."[15]
Lias
[edit | edit source]Def. a "stratigraphic group from the lower Jurassic period, consisting of thin layers of blue limestone [present in parts of southern England]"[16] is called a lias.
Technology
[edit | edit source]"A VIMS field crew [assembled in the image on the right] collects sediment cores on the landward side of the Plum Island barrier island in May 2014. These cores were collected with a Geoprobe drill rig [shown] and went as much as 60 feet below the surface of the island. Cores were collected in 4 feet sections and brought back to VIMS for processing."[17]
Sediment cores
[edit | edit source]Sediment cores may be obtained "by drilling or jack-hammering a steel rod or shoving a hand auger or hollow "push core" into a beach or marsh or water bottom, and pulling up sediment samples for analysis."[18]
"You can think of a sediment core as being more or less a tape recorder of time. Within that sediment core, we work with proxies, or environmental proxies, and these can be very simple measures of grain size or composition or some organic geochemical property or maybe pollen."[19]
"There are environments that preserve storm records that are buried in the sea bed, so that you can go down through time and actually develop a record of the intensity and frequency of cyclonic storms. That's something that's pretty high up on the radar for coastal inhabitants. Kind of understanding the pattern of these storms through time helps us to understand what might be coming down the pike."[19]
"You take a sediment core through a barrier island and under that is marsh, bay, marsh, mainland. You have maybe an old forest, roots. Looking back in time at that location, hundreds of thousands of years ago, you get this vertical succession of these different layers."[20]
Cenozoic
[edit | edit source]Quaternary
[edit | edit source]"In the Greenland ice cores, the Pleistocene–Holocene transition is chronologically constrained between two clearly defined tephra horizons: the Saksunarvatn tephra (1409.83 m depth) and the Vedde Ash (1506.14 m depth). These are dated at 10 347 yr b2 k (counting uncertainty 89 yr) and 12 171 yr (counting uncertainty 114 yr) b2 k, respectively."[21]
Meghalayan
[edit | edit source]The Meghalayan Global Boundary Stratotype Section and Point (GSSP) is a Krem Mawmluh Cave formation in Meghalaya, a state in Northeast India.[22] Mawmluh cave is one of the longest and deepest caves in India, and conditions here were suitable for preserving chemical signs of the transition in ages.[23] The global auxiliary stratotype is an ice core from Mount Logan in Canada.[24]
Northgrippian
[edit | edit source]Greenlandian
[edit | edit source]The lower boundary of the Greenlandian Age is the Global Boundary Stratotype Section and Point (GSSP) sample from the North Greenland Ice Core Project in central Greenland (75.1000°N 42.3200°W).[25] The Greenlandian GSSP has been correlated with the end of Younger Dryas (from near-glacial to interglacial) and a "shift in deuterium excess values".[25]
Chibanian
[edit | edit source]Calabrian
[edit | edit source]"The [Calabrian] GSSP occurs at the base of the marine claystone conformably overlying sapropelic bed ‘e’ within Segment B in the Vrica section. This lithological level represents the primary marker for the recognition of the boundary, and is assigned an astronomical age of 1.80 Ma on the basis of sapropel calibration."[26]
"The boundary falls between the highest occurrence of Discoaster brouweri (below) and the lowest common occurrence of left-coiling Neogloboquadrina pachyderma (above), and below the lowest occurrences of medium-sized Gephyrocapsa (including G. oceanica) and Globigerinoides tenellus."[26]
In the image on the right, the Vrica section includes specifically the GSSP of the Calabrian Stage fixed at the top of layer ‘e’.
Gelasian
[edit | edit source]"The base of the Quaternary System [shown in the image on the right] is defined by the Global Stratotype Section and Point (GSSP) of the Gelasian Stage at Monte San Nicola in Sicily, Italy, currently dated at 2.58 Ma."[27]
"The astrochronological age of sapropel MPRS 250 (mid-point), corresponding to precessional cycle 250 from the present, is 2.588 Ma (Lourens et al., 1996), which can be assumed as the age of the boundary."[28]
Piacenzian
[edit | edit source]"The base of the beige marl bed of the small-scale carbonate cycle 77 (sensu Hilgen, 1991b) is the approved base of the Piacenzian Stage (that is the Lower Pliocene-Middle Pliocene boundary). It corresponds to precessional excursion 347 as numbered from the present with an astrochronological age estimate of 3.600 Ma (Lourens et al., 1996a)."[29]
Bidahochi Formation
[edit | edit source]
Miocene–Pliocene 15.5–4.1 Ma.
Underlies: volcanic tuff (locally), or Quaternary alluvium.
The formation is underlain by various Mesozoic formations,[30] such as the Chinle Formation,[31] and is typically the uppermost named unit in its outcrop area.[30]
Overlies: Chinle Formation–(225 Ma), erosion unconformity.
Thickness: 488 feet (149 m) maximum (east of Bidahochi, AZ), variable.
Coordinates: 35.40°N 110.14°W.
Extent: SE perimeter Black Mesa (Arizona), NNW Defiance Plateau–(Uplift), S(E & NE north perimeter foothills White Mountains), NW & W Painted Desert.
The Bidahochi Formation was deposited in a large ephemeral lake basin covering much of northeastern Arizona from 4 to 8 million years ago.
At its reference section near Indian Wells, Arizona, the Bidahochi Formation is divided into three informal members. The lower member is 214 feet (65 m) thick and consists mostly of mudstone and fine sandstone with a basal conglomerate of limestone and siltstone pebbles. The beds show distorted crossbedding and ripple marks, and plant fossils (impressions and petrified wood) are present. The middle volcanic member, which is only 5 feet (1.5 m) thick, consists of volcaniclastics, mostly basalt and quartzite pebbles. The upper member is over 274 feet (84 m) thick, forms ledges and slopes, and is mostly mudrock and sandstone.[32]
The formation is likely middle Miocene to middle Pliocene in age, and the lower part of the formation may correlate with the Fence Lake Formation.[32][30] One of the lava flows within the formation has a radiometric age of 4.1 Ma,[33] and radiometric dating of other associated flows and ash beds gives the formation an age range of 15.5[34] to 4 million years.[30]
The lower member has been interpreted as a lacustrine facies and the upper member as a fluvial facies.[34] The lacustrine deposits suggests widespread lakes in the area, though geologists continue to debate whether there was a single large lake (Hopi Lake) or a series of smaller ephemeral lakes, or whether Hopi Lake was a shallow playa lake or a deep lake.[30][35][34][36] However, the evidence from the maars and scoria cones erupted through the formation favors a shallow, possibly ephemeral, lake.[34]
The formation has been likened to the Ogallala Formation of eastern New Mexico and west Texas in both age and depositional environment.[30]
The Bidahochi is possibly the only large non-volcanic geologic formation of Neogene age on the Colorado Plateau.[37] This has made it of interest to geologists attempting to reconstruct conditions on the Colorado Plateau during the Neogene[38] and for theories of the formation of the Grand Canyon.[30][35][34][36]
Palm Spring Formation
[edit | edit source]Imperial Formation
[edit | edit source]Underlies: Palm Spring Formation (California).
Overlies: Ocotillo Formation (California).
The Imperial Formation occurs in the Colorado Desert, in Imperial County of Southern California, dates to the Zanclean−Lower Pliocene stage of the Pliocene Epoch, during the Neogene Period of the Cenozoic Era.
Ocotillo Formation
[edit | edit source]Trubi Formation
[edit | edit source]
The Scala dei Turchi is formed by marl, a sedimentary rock with a characteristic white color, made from the tests of planktonic foraminifera, specifically a marine sedimentary unit of Lower Pliocene (Zanclean) age, deposited after the Zanclean flood, in which the Mediterranean refilled after having previously evaporated during the Messinian salinity crisis.[39]
"The boundary-stratotype of the stage is located in the Eraclea Minoa section on the southern coast of Sicily (Italy), at the base of the Trubi Formation. The age of the Zanclean and Pliocene GSSP at the base of the stage is 5.33 Ma in the orbitally calibrated time scale, and lies within the lowermost reversed episode of the Gilbert Chron (C3n.4r), below the Thvera normal subchron."[40]
In the chronostratigraphic correlation of the Piacenzian section, the base of the Zanclean is marked as the '0' point.
Messinian
[edit | edit source]"The GSSP of the Messinian Stage, which per definition marks the base of the Messinian and, hence, the boundary between the Tortonian and Messinian Stages of the Upper Miocene Subseries, is Oued Akrech (Morocco) where the Messinian GSSP is now formally designated at the base of the reddish layer of sedimentary cycle no. 15. This point coincides closely with the first regular occurrence (FRO) of the planktonic foraminiferal Globorotalia miotumida group and the first occurrence (FO) of the calcareous nannofossil Amaurolithus delicatus, and falls within the interval of reversed polarity that corresponds to C3Br.1r. The base of the reddish layer and, thus, the Messinian GSSP has been assigned an astronomical age of 7.251 Ma."[41]
"The correlation of characteristic sedimentary cycle patterns to the astronomical record resulted in an astronomical age of 7.24 Ma (Hilgen et al., 1995), in good agreement with the radiometric age estimates of Vai et al. (1993) and Laurenzi et al. (1997)."[41]
The integrated magnetostratigraphy, calcareous plankton biostratigraphy and cyclostratigraphy of section Oued Akrech is diagrammed on the left.
Tortonian
[edit | edit source]Serravallian
[edit | edit source]Langhian
[edit | edit source]Burdigalian
[edit | edit source]Bolderian
[edit | edit source]The Bolderian is named after the Boldenberg Formation of the Miocene (Dumont, 1850). The Opitter Member is part of the Boldenberg Formation.
Stratigraphic members of the Boldenberg Formation:
- Molenbeersel member
- Genk Member
- Houthalen Member
- Elsloo gravel
"UA7 correlates to the Genk Member [BbGe] and UA6 to the Houthalen Member [BbHh] of the Bolderberg Formation [Bb] (Deckers & Louwye, 2017)."[42]
"The unit 35 of Table 3 (UA3) was interpreted in the borehole file as Mol Formation, units 40–49 (UA4) as Kasterlee Formation, UA5 as Diest Formation, UA6 as Genk Member of the Bolderberg Formation, UA7 as the Houthalen Member of the Bolderberg Formation, followed by UA8, the Voort Formation."[42]
The Boldenberg is followed by the Diest and Kasterlee Formations.[42]
"Although all deposits are assigned to known formations, they occur in reduced thicknesses and show deviating sedimentary characteristics so that local members had to be introduced (Sels et al., 2001): (1) is the "Dorperberg member" (Zanden van Dorperberg) of the latest Miocene Kasterlee Formation; (2) is the "Gruitrode Mill member" (Zanden van de Molen van Gruitrode) of the Late Miocene Diest Formation; and (3) is the "Opitter member" (Lid van Opitter) of the Early to Middle Miocene Bolderberg Formation."[42]
"Houthuys & Matthijs (2020, [...]) and Dusar (archives BGD 048E0294) have argued that the Opitter sand, interpreted as part of the Bolderberg Formation in the sand pit, is erroneous."[43]
"The Elsloo gravel is overlain by the marine Houthalen Member: a dark green, often clayey, medium fine-grained sandy unit, micaceous, slightly ligniferous and glauconitic."[43]
"The Neogene depositional history in the eastern part of the [Kempen Basin] CB and on the [Bree Uplift] BU starts with the Early to Middle Miocene Bolderberg Formation on top of the Oligocene Voort Formation. This formation is the local lateral correlate of the Berchem Formation. The base of the Bolderberg Formation is characterized by the presence of the Elsloo gravel which contains flint pebbles, rounded phosphate concretions, coarse quartz grains, shark teeth and reworked fossils, marking an important transgressive surface. It is followed by the thinly developed shallow marine glauconiferous Houthalen Member and the thicker marginal marine Genk Member. The latter consists of yellow to white fine sands which are poor in glauconite and may contain continental elements such as layers of lignite and flint pebbles. The Bolderberg Formation is truncated by a well-developed marine transgressive surface covered by the late Miocene green glauconite-rich sand of the Diest Formation (Houthuys et al., 2020, [...]). This is in turn truncated and covered by fine sand and clayey sand of the latest Miocene to early Pliocene Kasterlee Formation (Vandenberghe et al., 2020, [...]). They are shallow marine to coastal and show to the south a transition to backbarrier marginal marine deposits (Verhaegen et al., 2020, [...]). In NE Belgium, they are separated by a transgressive surface from the Pliocene Mol Formation. These white fine to medium-coarse quartz sands were also deposited in a marginal marine area, though more exposed to waves than the backbarrier facies of the Kasterlee Formation. The Mol Formation contains lignitic horizons and has dispersed small lumps or drapes of white, kaolinitic clay. The lower part of the formation may have a slight admixture of often clay-sized glauconite (Vandenberghe et al., 2020, [...]). The overall palaeogeographic evolution is from shallow marine to marginal marine."[42]
"A new member, the Molenbeersel member, is proposed for the glauconite-bearing silts and fine sands in the upper part of the Bolderberg Formation in the Roer Valley Graben.Rhine during the late Tortonian."[43]
"The North Sea was a semi-enclosed basin during the Neogene. The marine connection between the southern North Sea Basin and the Channel was prevented by the Weald-Artois ridge, while in the north an open marine connection with the Norwegian-Greenland Sea existed (Ziegler, 1990; Rasmussen et al., 2008; Rasmussen et al., 2010)."[43]
"Tectonic uplift of northern Belgium during late Oligocene time pushed the southern coastline of the North Sea northwards, and late Oligocene sedimentation in Belgium was limited to a thin cover of glauconitic sand in northern Belgium; it is only well developed in the subsiding Roer Valley Graben (RVG) in the very northeast (Dusar et al., 2020, [...]). At the beginning of the Miocene, during the global sea-level rise after the Mi1 glacial maximum (Miller et al., 1991), sea levels rose and northern Belgium was invaded by a marine transgression from a northern to northwestern direction (Louwye, 2005). Furthermore, Munsterman & Deckers (2020, [...]) recorded in two wells in the northeastern part of the Campine latest Oligocene – earliest Miocene (Aquitanian – early Burdigalian) deposits and proposed a transgressive phase from the Roer Valley Graben towards the Campine area."[43]
"The Berchem Formation holds the Edegem Member, the Kiel Member, the Antwerpen Member and the Zonderschot Member, while the Bolderberg Formation is divided into the Houthalen Member and the Genk Member, including the white quartz sand Opgrimbie facies [...]. The complete thickness of the Berchem Formation increases from about 30 m in the west to over 100 m in the east. The Bolderberg Formation has a maximum thickness of circa 160 m in the Roer Valley Graben in northeast Belgium (Molenbeersel borehole (BGD 049w0225, DOV kb18d49w-B225) according to Broothaers et al. (2012), truncates locally the subjacent formations and wedges out in a westward direction."[43]
"The lower boundary of the Bolderberg Formation with the Oligocene Voort or Eigenbilzen Formations is not easy to delineate on geophysical log data, but regularly coincides with an upwards increase in gamma ray values and decrease in resistivity values (Deckers et al., 2019) (Fig. 9). The lower part of the Bolderberg Formation consists of high gamma ray and relatively low resistivity values of the glauconite-rich, clayey, fine-grained Houthalen Member. The upper—generally thicker—part consists of low to very low gamma ray and relatively high resistivity values of the glauconite-poor, coarser-grained Genk Member. The transition between the Houthalen and Genk Members is consequently expressed by a gradual upward decrease in gamma ray values and increase in resistivity. This gradual change continues in the lower part of the Genk Member, and is interpreted as representing a coarsening upwards trend (Deckers & Louwye, 2017)."[43]
"The Bolderberg Formation is topped by the basal gravel layer of the Diest Formation. On wireline logs, the boundary between the Bolderberg Formation and Diest Formation coincides with a subtle increase in gamma ray values caused by an increase in glauconite content (Deckers & Louwye, 2017)."[43]
"Wouters (1978) recognized in the Houthalen Member the ostracod Pterygocytheris continens – Kuiperiana wanneri Zone, which is correlatable to the lower Miocene U2 zone of Gramann (1988)."[43]
"The base of the Edegem Member is formed by the Burcht Gravel consisting of dark rounded flint pebbles, shell fragments, shark teeth and bone fragments. Reworked foraminifers, septaria and glauconite provide evidence for substantial reworking of sediment from the underlying Oligocene Boom Formation (Vandenberghe et al., 1998)."[43]
"The well-developed, transgressive Elsloo gravel at the base of the Bolderberg Formation consists of reworked Oligocene components, dark blue, egg-shaped, indented phosphate pebbles and shark teeth (Vandenberghe et al., 1998). Tavernier (1954) already stressed the importance of the Elsloo gravel as a reference level. These characteristic pebbles occur under a thin glauconitic sand between Leuven and Tienen (Vandenberghe & Gullentops, 2001), while Houthuys (2014) reports similar pebbles in the Flemish Hill sand base gravel near Ronse. The Elsloo gravel is overlain by the marine Houthalen Member: a dark green, often clayey, medium fine-grained sandy unit, micaceous, slightly ligniferous and glauconitic. Similar to the Berchem Formation, dispersed and concentrated mollusks occur which are also reworked in the basal gravel of the superjacent Diest Formation (De Meuter & Laga, 1976)."[43]
The Elsloo gravel dates from 23.03 Ma to 19 Ma of the Houthalen Member based on the presence of reworked fossils and the Houthalen Member above. "As is the case for the Edegem Member, the Kiel Member contains glauconite which, based on radiometric dating and grain-size distribution curves, are presumed to be reworked (Odin et al., 1974; Vandenberghe et al., 2014; Adriaens, 2015). The radiometric datings further show divergence between K-Ar ages (23 to 25.3 Ma; Chattian) and Rb-Sr ages (30 Ma; Rupelian) (Odin & Kreuzer, 1988)."[43]
Aquitanian
[edit | edit source]Oligocene
[edit | edit source]

The Oligocene dates from 33.9 ± 0.1 x 106 to 23.03 x 106 b2k.
The Oligocene Epoch covers 34 - 23 Mya.
During the Oligocene Epoch, the continents continued to drift toward their present positions.[45][46]
A deep 400,000-year glaciated Oligocene-Miocene boundary event is recorded at McMurdo Sound and King King George Island.[47]
Oligocene climate following the Eocene-Oligocene event is poorly known.[48] There were several pulses of glaciation in middle Oligocene, about the time of the Oi2 oxygen isotope shift. This led to the largest drop of sea level in past 100 million years, by about 75 meters (246 ft). This is reflected in a mid-Oligocene incision of continental shelves and unconformities in marine rocks around the world.[49]
Some evidence suggests that the climate remained warm at high latitudes[50][51] even as ice sheets experienced cyclical growth and retreat in response to orbital forcing and other climate drivers.[52] Other evidence indicates significant cooling at high latitudes.[53][54] Part of the difficulty may be that there were strong regional variations in the response to climate shifts. Evidence of a relatively warm Oligocene suggests an enigmatic climate state, neither hothouse nor icehouse.[55]
The timing of the formation of the Drake Passage between South America and Australia is also uncertain, with estimates ranging from 49 to 17 mya (early Eocene to Miocene),[56] but oceanic circulation through the Drake Passage may also have been in place by the end of the early Oligocene.[57][58] This may have been interrupted by a temporary constriction of the Drake Passage from sometime in the middle to late Oligocene (29 to 22 mya) to the middle Miocene (15 mya).[59]
The Drake Passage is located between South America and Antarctica. Once the Tasmanian Gateway between Australia and Antarctica opened, all that kept Antarctica from being completely isolated by the Southern Ocean was its connection to South America. As the South American continent moved north, the Drake Passage opened and enabled the formation of the Antarctic Circumpolar Current (ACC), which would have kept the cold waters of Antarctica circulating around that continent and strengthened the formation of Antarctic Bottom Water (ABW).[60][61] With the cold water concentrated around Antarctica, sea surface temperatures and, consequently, continental temperatures would have dropped. The onset of Antarctic glaciation occurred during the early Oligocene,[62] and the effect of the Drake Passage opening on this glaciation has been the subject of much research. However, some controversy still exists as to the exact timing of the passage opening, whether it occurred at the start of the Oligocene or nearer the end. Even so, many theories agree that at the Eocene/Oligocene (E/O) boundary, a yet shallow flow existed between South America and Antarctica, permitting the start of an Antarctic Circumpolar Current.[63]
Stemming from the issue of when the opening of the Drake Passage took place, is the dispute over how great of an influence the opening of the Drake Passage had on the global climate. While early researchers concluded that the advent of the ACC was highly important, perhaps even the trigger, for Antarctic glaciation[60] and subsequent global cooling, other studies have suggested that the δ18O signature is too strong for glaciation to be the main trigger for cooling.[63] Through study of Pacific Ocean sediments, other researchers have shown that the transition from warm Eocene ocean temperatures to cool Oligocene ocean temperatures took only 300,000 years,[64] which strongly implies that feedbacks and factors other than the ACC were integral to the rapid cooling.[64]
The latest hypothesized time for the opening of the Drake Passage is during the early Miocene.[64] Despite the shallow flow between South America and Antarctica, there was not enough of a deep water opening to allow for significant flow to create a true Antarctic Circumpolar Current. If the opening occurred as late as hypothesized, then the Antarctic Circumpolar Current could not have had much of an effect on early Oligocene cooling, as it would not have existed.
The earliest hypothesized time for the opening of the Drake Passage is around 30 Ma.[64] One of the possible issues with this timing was the continental debris cluttering up the seaway between the two plates in question. This debris, along with what is known as the Shackleton Fracture Zone, has been shown in a recent study to be fairly young, only about 8 million years old.[61] The study concludes that the Drake Passage would be free to allow significant deep water flow by around 31 Ma. This would have facilitated an earlier onset of the Antarctic Circumpolar Current.
Currently, an opening of the Drake Passage during the early Oligocene is favored.
The reorganization of the oceanic tectonic plates of the northeastern Pacific, which had begun in the Paleocene, culminated with the arrival of the Murray and Mendocino Fracture Zones at the North American subduction zone in the Oligocene. This initiated strike-slip movement along the San Andreas Fault and extensional tectonics in the Basin and Range province,[65] ended volcanism south of the Cascades, and produced clockwise rotation of many western North American terranes. The Rocky Mountains were at their peak. A new volcanic arc was established in western North America, far inland from the coast, reaching from central Mexico through the Mogollon-Datil volcanic field to the San Juan volcanic field, then through Utah and Nevada to the ancestral Northern Cascades. Huge ash deposits from these volcanoes created the White River and Arikaree Groups of the High Plains, with their excellent fossil beds.[66]
Between 31 and 26 mya, the Ethiopia-Yemen Continental Flood Basalts were emplaced by the East African large igneous province, which also initiated rifting along the Red Sea and Gulf of Aden.[67]
The Alps were rapidly rising in Europe as the African plate continued to push north into the Eurasian plate, isolating the remnants of the Tethys Sea.[68][69] Sea levels were lower in the Oligocene than in the early Eocene, exposing large coastal plains in Europe and the Gulf Coast and Atlantic Coast of North America.
The Obik Sea, which had separated Europe from Asia, retreated early in the Oligocene, creating a persistent land connection between the continents.[70]
One recent hypothesis is that a separate microcontinent collided with south Asia in the early Eocene, and India itself did not collide with south Asia until the end of the Oligocene.[71][72] The Tibetan Plateau may have reached nearly its present elevation by the late Oligocene.[73]
The Andes first became a major mountain chain in the Oligocene, as subduction became more direct into the coastline.[74][75]
Climate during the Oligocene reflected a general cooling trend following the Early Eocene Climatic Optimum. This transformed the Earth's climate from a greenhouse to an icehouse climate.[76]
The best terrestrial record of Oligocene climate comes from North America, where temperatures dropped by 7 to 11 °C (13 to 20 °F) in the earliest Oligocene. This change is seen from Alaska to the Gulf Coast. Upper Eocene paleosols reflect annual precipitation of over a meter of rain, but early Oligocene precipitation was less than half this.[77][78] In central North America, the cooling was by 8.2 ± 3.1 °C over a period of 400,000 years, though there is little indication of significant increase in aridity during this interval.[79] Ice-rafted debris in the Norwegian-Greenland Sea indicated that glaciers had appeared in Greenland by the start of the Oligocene.[80]
Continental ice sheets in Antarctica reached sea level during the transition.[81][82][83] Glacially rafted debris of early Oligocene age in the Weddell Sea and Kerguelen Plateau, in combination with Oi1 isotope shift, provides unambigous evidence of a continental ice sheet on Antarctica by the early Oligocene.[84]
The causes of the Eocene-Oligocene transition are not yet fully understood.[85] The timing is wrong for this to be caused either by known impact events or by the volcanic activity on the Ethiopean Plateau.[86] Two other possible drivers of climate change, not mutually exclusive, have been proposed.[87] The first is thermal isolation of the continent of Antarctica by development of the Antarctic Circumpolar Current.[88][89][46] Deep sea cores from south of New Zealand suggest that cold deep-sea currents were present by the early Oligocene.[90] However, the timing of this event remains controversial.[91] The other possibility, for which there is considerable evidence, is a drop in atmospheric carbon dioxide levels (pCO2) during the transition.[92][93] The pCO2 is estimated to have dropped just before the transition, to 760 ppm at the peak of ice sheet growth, then rebounded slightly before resuming a more gradual fall.[94] Climate modeling suggests that glaciation of Antarctica took placed only when pCO2 dropped below a critical threshold value.[95]
The Oligocene sees the beginnings of modern ocean circulation, with tectonic shifts causing the opening and closing of ocean gateways. Cooling of the oceans had already commenced by the Eocene/Oligocene boundary.[64]
The opening and closing of ocean gateways: the opening of the Drake Passage; the opening of the Tasmanian Passage (Tasmanian Gateway) and the closing of the Tethys seaway; along with the final formation of the Greenland–Iceland–Faroes Ridge; played vital parts in reshaping oceanic currents during the Oligocene. As the continents shifted to a more modern configuration, so too did ocean circulation.[60]
The other major oceanic gateway opening during this time was the Tasman, or Tasmanian, depending on the paper, gateway between Australia and Antarctica. The time frame for this opening is less disputed than the Drake Passage and is largely considered to have occurred around 34 Ma. As the gateway widened, the Antarctic Circumpolar Current strengthened.
The Tethys Seaway was not a gateway, but rather a sea in its own right. Its closing during the Oligocene had significant impact on both ocean circulation and climate. The collisions of the African plate with the European plate and of the Indian subcontinent with the Asian plate, cut off the Tethys Seaway that had provided a low-latitude ocean circulation.[96] The closure of Tethys built some new mountains (the Zagros range) and drew down more carbon dioxide from the atmosphere, contributing to global cooling.[97]
The gradual separation of the clump of continental crust and the deepening of the tectonic ridge in the North Atlantic that would become Greenland, Iceland, and the Faroe Islands helped to increase the deep water flow in that area.[62] More information about the evolution of North Atlantic Deep Water will be given a few sections down.
Isotopic evidence suggests that during the early Oligocene, the main source of deep water was the North Pacific and the Southern Ocean. As the Greenland-Iceland-Faroe Ridge sank and thereby connected the Norwegian–Greenland sea with the Atlantic Ocean, the deep water of the North Atlantic began to come into play as well. Computer models suggest that once this occurred, a more modern in appearance thermo-haline circulation started.[96]
Evidence for the early Oligocene onset of chilled North Atlantic deep water lies in the beginnings of sediment drift deposition in the North Atlantic, such as the Feni and Southeast Faroe drifts.[62]
The chilling of the South Ocean deep water began in earnest once the Tasmanian Gateway and the Drake Passage opened fully.[61] Regardless of the time at which the opening of the Drake Passage occurred, the effect on the cooling of the Southern Ocean would have been the same.
Recorded extraterrestrial impacts:
- Haughton impact crater, Nunavut, Canada (23 Ma, crater 24 kilometres (15 mi) diameter) (now considered questionable as an Oligocene event; later analyses have concluded the crater dates to 39 Ma, placing the event in the Eocene.)[98][99]
La Garita Caldera (28 through 26 million years ago)[100]
Chattian
[edit | edit source]Rupelian
[edit | edit source]Priabonian
[edit | edit source]Bartonian
[edit | edit source]Messel formation
[edit | edit source]
The Messel Formation is a geologic formation in Hesse, central Germany, dating back to the Eocene epoch (about 47 Ma[101]). Its geographic range is restricted to the Messel pit. There it unconformably overlies crystalline Variscan basement and its Permian cover (Rotliegend) as well as Eocene volcanic breccias derived from the basement rocks. The formation mainly comprises lacustrine laminated bituminous shale ('oil shale') renowned for its content of fossils in exceptional preservation, particularly plants, arthropods and vertebrates (e.g. Darwinius masillae).
The Messel Formation forms an isolated Eocene deposit in the middle of the Rotliegend sediments.[102]
So far, representatives of all vertebrate groups as well as insects and plants have been found. The best-known representatives of the Messel fauna are probably the two early equines Propalaeotherium and Eurohippus, of which over 70 individuals have been excavated so far. Other important finds are the crane Messelornis cristata and an early primate Darwinius masillae ("Ida").
The rocks on which the sediments of the Eocene Messel Formation rests were formed over 300 Ma. Some of these are granitoid plutons, which arose in the late phase of the Variscan orogeny in the Upper Carboniferous, and some of them are even older, mostly originally igneous rocks that were sunk deep into the earth's crust during the orogeny and are there as a result of high pressure and temperature (e.g. amphibolite).
From the erosion of the Variscan high mountains, sedimentary layers of erosion debris, so-called molasse, were deposited at the end of the Carboniferous and in the course of the Permian in basins in the interior of the mountains and in the foothills. In Central Europe the variscid molasse is generally called the Rotliegend. In the Messel area these are the Moret layers of the upper Rotliegend.[103]
Molasse is the name for sediments and sedimentary rocks that are created when a mountain range (from an orogeny) is eroded down to its basement.
In the Mesozoic, the Rotliegend Molasse was overlain by other sediments, including the sandstones and claystones of the red sandstone (Lower Triassic), which can be found today southeast and east of the Darmstadt area, in the Odenwald sandstone and in the Spessart sandstone.
The history of the Messel oil shale begins about 48 million years ago in the Eocene.
A research borehole that was sunk in autumn 2001 revealed that a volcanic center was located in the Messel area. Basaltic magma rose towards the surface of the earth and hit groundwater, causing a huge steam explosion. This explosion occurred less than 100 meters below the surface of the earth at that time and not only blasted a deep crater into the landscape, but also disrupted the surrounding rock. This enabled water to penetrate in the direction of the volcanic center and the next steam explosion then took place at a correspondingly greater depth. By repeating this process several times, a more than 700 meters deep explosion funnel was created in the basement. These explosions occurred 48.49 to 47.89 million years ago.[104] While the lower part of the funnel contains rock debris (breccia) and tuff, the top 200–300 meters filled with water after the volcanism subsided and a maar lake formed.[102] Various sediments were then deposited in this lake, mainly the bituminous claystone, which is known today as "Messel oil shale".
Lutetian
[edit | edit source]Ypresian
[edit | edit source]Thanetian
[edit | edit source]Selandian
[edit | edit source]Ojo Alamo Sandstone
[edit | edit source]
"A hiatus of about 8 m.y. separates Late Cretaceous from Tertiary rocks in the [San Juan] Basin. Most of the missing strata are from the Maastrichtian Stage. The unconformity is overlain by the Ojo Alamo Sandstone in the south and underlain by the Kirtland or Fruitland Formation at most other places in the basin."[105]
North Horn Formation
[edit | edit source]

The North Horn Formation is a widespread non-marine sedimentary unit with extensive outcrops exposed in central and eastern Utah. The formation locally exceeds 3,600 feet (1,100 m) in thickness and is characterized by fluvial, lacustrine, and floodplain dominated systems, representing a terrestrial, high energy, depositional environment.[106][107][108] The sediments date from Late Cretaceous (Maastrichtian) to early Paleocene in age and include the K-Pg extinction event boundary; however, this boundary is extremely difficult to locate and there is no strong stratigraphic evidence available that indicates a specific marker bed such as an iridium rich clay layer.[109][110] Thus far, the only visible evidence is represented in the form of faunal turnover from dinosaur to mammal-dominated fossil assemblages. Taxa from the Cretaceous part of the formation include squamates, testudines, choristoderes, crocodyliforms, sharks, bony fishes, amphibians, mammals, dinosaurs, eggshell fragments, trace fossils, mollusks, plant macrofossils, such as wood fragments, and palynomorphs.[111][112][113][114]
The North Horn Formation is a non-marine, stratigraphic unit located in east and central Utah which unconformably overlies the Late Cretaceous Price River Formation west of the San Rafael Swell, and the Tuscher Formation east of the swell.[107] The formation is overlain by the late Paleocene-early Eocene Flagstaff Formation, also called the Flagstaff Limestone.[106][107] The North Horn type section is located on North Horn Mountain in Emery County, Utah.[115] Laterally, the North Horn Formation nearly spans an 87-mile (140 km) long east–west transect that extends from the Wasatch Plateau on the west and the Book Cliffs, near Green River, on the east, separated in the middle by the San Rafael Swell.[108] The North Horn Formation varies greatly in thickness and lithology, representing a time transgressive stratigraphic sequence, which means that the age of the base and top of the formation changes as one moves laterally. West of the San Rafael Swell, near Price Canyon, Utah, the basal contact for the North Horn Formation is Maastrichtian (latest Cretaceous) in age,[106][107][108] whereas its base is Paleocene in age on the eastern side of the swell.[107] Some of the most complete sections of the North Horn Formation are exposed west of the San Rafael Swell at North Horn Mountain in which the local stratigraphy sometimes exceeds 3,600 ft (1,100 m) in thickness. Further to the east, towards Green River, Utah, stratigraphic sections are significantly thinner compared to sections in the west with thicknesses varying between as little as 50 to 130 ft (15 to 40 m).[107][109]
The formation is divided into three informal units based on broad but distinct lithological characteristics. These units include the lower variegated unit at the base, a middle coal unit (in which the K-Pg boundary is located), and the upper variegated unit at the top. The units themselves are further divided into a total of eight sub-units, or lithofacies, based on facies scale lithological features.[106][108][109] A detailed sedimentary study of the North Horn Formation was conducted by Lawton et al.[107] These units, from oldest to youngest, include a 330 ft (100 m) thick basal conglomerate unit that consists of an upward fining conglomerates and sandstone and lacks any interbedded shale, followed by the lower redbed unit, a 790 ft (240 m) thick sequence dominated by red sandy siltstone, conglomerate, and pebbly sandstone. The third unit is the sheet sandstone unit and is composed of sheetlike sandstone beds that are interbedded with gray and carbonaceous siltstones. Some limestone beds are present but are uncommon. The fourth unit is characterized by 131 to 374 ft (40 to 114 m) thick coal beds and coal-streaked siltstone deposits and are referred to as the coal-bearing unit. This unit is overlain by a 833 ft (254 m) thick sequence of primarily calcareous siltstone beds, called the calcareous siltstone unit. The big mountain unit consists of nearly 750 feet (230 m) of interbedded conglomerates and trough cross bedded sandstones, followed by a 200 ft (60 m) thick set of strata composed of sandstone, pebbly sandstone, and conglomerates which make up the coal canyon unit. The uppermost unit is the upper redbed unit, which is nearly a 390 ft (120 m) thick and is composed of red brown mottled siltstone and sandy siltstone.
Characteristic dinosaur taxa include the ceratopsian Torosaurus utahensis, the titanosaurid sauropod Alamosaurus sanjuanensis, and the theropod Tyrannosaurus;[116][117][118] however, the most frequently occurring taxon in the Cretaceous strata of the North Horn Formation is the Polyglyphanodont squamate Polyglyphanodon.[111] Fauna recorded from Paleocene strata within the formation appear to be far more diverse and over 70 different taxa have been identified, including frogs, numerous multituberculate, protoeutheria (protoeutherians), periptychidae (periptychids), arctocyonids and phenacodontid mammals, crocodyliforms, choristoderes, trace fossils, and palynomorphs.[109][112][113][111] Marsupial remains similar to Alphadon and Eodelphis have also been found here as well.[119]
Pterodactyloid tracks present at an unnamed site. The specimens are kept at the Dinosaur Tracks Museum, of the University of Colorado at Denver]].[120] Bird and dinosaur eggs have also been found at the site, along with unidentified hadrosaur fossils (possibly from Edmontosaurus or Kritosaurus).[111]
Genus | Species | Location | Stratigraphic position | Abundance | Notes | Image |
---|---|---|---|---|---|---|
Adocus[111] | A. sp<[111] | ![]() | ||||
Alamosaurus[121] | A. sanjuanensis[121] | ![]() | ||||
Basilemys[111] | B. sp[111] | ![]() | ||||
Champsosaurus[111] | C. sp[111] | ![]() | ||||
Lepisosteus[111] | L. sp.[111] | ![]() | ||||
Polyglyphanodon[111] | P. sternbergi[111] | |||||
Pteraichnus[120] | Found at an unnamed site.[120] | Specimens kept at the Dinosaur Tracks Museum, of the University of Colorado at Denver.[120] | ||||
Torosaurus[121] | T. utahensis[121] | ![]() | ||||
Tyrannosaurus[118][121] | T. rex[118][121] | ![]() |
Basque Coast Geopark
[edit | edit source]The Basque Coast Geopark comprises 89 square kilometers of countryside with a 23 km long cliffed coast fronting the Bay of Biscay. The coast is characterised by steep cliffs of flysch of late Cretaceous to Palaeogene age. The flysch cliffs arranged along the coast record 60 million years of unbroken deposition of marine sediments from the Cretaceous period through into the Palaeocene and Eocene stages of the succeeding Palaeogene period. Alternating layers of sandstone and mudstone deposited as turbidite flows were originally laid down flat but have been tilted at angles up to the vertical by tectonic forces associated with the Alpine orogeny. An extensive wave-cut platform is exposed at low tide. These coastal sections include outcrops which record the significant Cretaceous-Paleogene boundary (K-T) (or K-Pg) boundary defined by the Cretaceous–Paleogene extinction event which caused the demise of the dinosaurs some 66.1 million years ago.[122]
The oldest bedrock exposed within the area dates from the late Triassic period and comprises the gypsums and clays found in the Mutriku area. The youngest are sandstones, limestones and shales of the Eocene part of the Palaeogene, found around Zumaia.[123]
The "Zumaia cliffs (Basque Country) [...] have an exceptional section of strata that reveals the geological history of the Earth in the period of 115-50 million years ago (Ma)."[124]
The "climate changes that occurred just before and after the massive extinction marked by the K/Pg boundary, as well as its potential relation to this large biological crisis [were analyzed]. For the first time, [...] whether this climate change coincides on the time scale with its potential causes: the Deccan massive volcanism (India) ─one of the most violent volcanic episodes in the geological history of the planet─ and the orbital variations of the Earth [was examined]."[124]
"The particularity of the Zumaia outcrops lies in that two types of sediments accumulated there ─some richer in clay and others richer in carbonate─ that we can now identify as strata or marl and limestone that alternate with each other to form rhythms. This strong rhythmicity in sedimentation is related to cyclical variations in the orientation and inclination of the Earth axis in the rotation movement, as well as in the translational movement around the Sun."[124]
"These astronomic configurations ─the known Milankovitch cycles, which repeat every 405,000, 100,000, 41,000 and 21,000 years─, regulate the amount of solar radiation they receive, modulate the global temperature of our planet and condition the type of sediment that reaches the oceans."[124]
Three "intense climatic warming events ─known as hyperthermal events─ that are not related to the Chicxulub impact [were revealed]. The first, known as LMWE and prior to the K/Pg boundary, has been dated to between 66.25 and 66.10 Ma. The other two events, after the mass extinction, are called Dan-C2 (between 65.8 and 65.7 Ma) and LC29n (between 65.48 and 65.41 Ma)."[124]
All "these events are in sync with extreme orbital configurations of the Earth known as eccentricity maxima. Only the LMWE, which produced an estimated global warming of 2-5°C, appears to be temporally related to a Deccan eruptive episode, suggesting that it was caused by a combination of the effects of volcanism and the latest Cretaceous eccentricity maximum.”[125]
"The global climate changes that occurred in the late Cretaceous and early Palaeogene ─between 250,000 years before and 200,000 years after the K/Pg boundary─ were due to eccentricity maxima of the Earth’s orbit around the Sun."[124]
"However, the orbital eccentricity that influenced climate changes before and after the K/Pg boundary is not related to the late Cretaceous mass extinction of species. The climatic changes caused by the eccentricity maxima and augmented by the Deccan volcanism occurred gradually at a scale of hundreds of thousands of years."[124]
"These data would confirm that the extinction was caused by something completely external to the Earth system: the impact of an asteroid that occurred 100,000 years after this late Cretaceous climate change (the LMWE). Furthermore, the last 100,000 years before the K/Pg boundary are characterized by high environmental stability with no obvious perturbations, and the large mass extinction of species occurred instantaneously on the geological timescale."[124]
Danian
[edit | edit source]

"The GSSP section near El Kef contains one main feature that allows for a direct correlation of this marine section with continental sections: the Ir anomaly at the base of the Boundary Clay."[126] In the image on the right, the finger is pointing to the K/Pg boundary clay in the Geulhemmergroeve tunnels near Geulhem, The Netherlands.
The image on the top left shows the K-Pg boundary in the Badlands near Drumheller, Alberta, where glacial and post-glacial erosion have exposed the boundary.
The K-Pg boundary at Trinidad Lake State Park, Colorado, USA, in the second image down on the right, occurs at the color change from dark gray or black to the Cenozoic light tans and browns.
The Global Boundary Stratotype Section and Point for the base of the Danian Stage is also the base GSSP for the Paleocene, Paleogene, "Tertiary", and Cenozoic at El Kef, Tunisia.
Mesozoic
[edit | edit source]Cretaceous
[edit | edit source]Kirtland Formation
[edit | edit source]Fruitland Formation
[edit | edit source]Mesa Verde Group
[edit | edit source]Mancos Shale
[edit | edit source]
Uinta Piceance Basin stratigraphic column. Orange is the Green River System, dark purple is the Mesaverde System, dark green is the Ferron/Wasatch Plateau System, light green is the Mancos/Mowry System, and light purple is the Phosphoria System. Green circles represent significant oil production, open star-circles represent significant gas production, and "S"s represent source rocks.
Maastrichtian
[edit | edit source]Lewis Shale
[edit | edit source]
Generalized stratigraphic column of Upper Cretaceous strata in the San Juan Basin, northwestern New Mexico, shows the stratigraphic positions of the nodosaurids I. zephyri and Glyptodontopelta mimus and the ankylosaurids Ahshislepelta minor, Nodocephalosaurus kirtlandensis, and Ziapelta sanjuanensis. Ankylosaur occurrence data are from Sullivan & Lucas (2015).
Campanian
[edit | edit source]Santonian
[edit | edit source]Cody Shale
[edit | edit source]Underlies: Mesaverde Formation.
Overlies: Frontier Formation.
Thickness: 500-1000 m.
Extent: Wyoming, Idaho, Montana.
The formation is described as follows: upper part is buff, sandy shale and thinly laminated buff sandstone; lower part is dark gray, thin-bedded marine shale.[127]
The formation is divided into many members that vary regionally. Alphabetically:[128]
- Ardmore Bentonite Beds (WY)
- Belle Fourche Formation (Belle Fourche Member) (MT, WY)
- Carlile Formation (Carlile Member) (MT, WY)
- Claggett Member (MT, WY)
- Eldridge Creek Member (MT)
- Gammon Ferruginous Member (MT, WY)
- Greenhorn Limestone (Greenhorn Calcareous Member) (MT)
- Niobrara Formation (Niobrara Member) (MT, WY)
- Sage Breaks Member (WY)
- Shannon Sandstone Member (MT, WY)
- Steele Member (WY)
- Sussex Sandstone Member (WY)
- Telegraph Creek Member (MT, WY)
- Wallace Creek Tongue (WY)
The Greenhorn is classified as a formation in Colorado and Kansas.
Frontier Formation
[edit | edit source]

Age: Cenomanian-Coniacian.
Subunits: Torchlight Sandstone Member, Peay Sandstone Member
Underlies: Cody Shale.
Overlies: Mowry Shale, Thermopolis Shale.
The formation is thick, lenticular, grey sandstone, gray shale, carbonaceous shale, and bentonite.[129]
The image on the right shows an outcrop of the Frontier Sandstone (Frontier Formation). The rocks in this area have been tectonically tilted - the beds are dipping toward the viewer. The Upper Cretaceous Frontier Sandstone is a coarse-grained, shallow water marine to shoreline unit. Marine deposits of Cretaceous age are widespread in western North America - they represent sedimentation during a sea level highstand. At this time, global sea level became so high that an ocean called the Western Interior Seaway separated eastern and western North America. The Cretaceous transgression was a result of relatively rapid seafloor spreading rates (tectonic divergence) in some oceans basins. This lifted considerable portions of the seafloor, which raised global sea levels.
Dinosaur remains are among the fossils that have been recovered from the formation.[130]
- Nodosaurus textilis
- Stegopelta landerensis - "Partial postcranium, osteoderms, [and] fragments of skull."[130]
- Hadrosauroidea indet. Footprints (Upper)[131]
- Callichimaera perplexa
Turonian
[edit | edit source]Cenomanian
[edit | edit source]Albian
[edit | edit source]Aptian
[edit | edit source]Barremian
[edit | edit source]Hauterivian
[edit | edit source]Valanginian
[edit | edit source]Berriasian
[edit | edit source]Jurassic
[edit | edit source]Tithonian
[edit | edit source]Kimmeridgian
[edit | edit source]Oxfordian
[edit | edit source]Callovian
[edit | edit source]Bathonian
[edit | edit source]Bajocian
[edit | edit source]Aalenian
[edit | edit source]Toarcian
[edit | edit source]Pliensbachian
[edit | edit source]Sinemurian
[edit | edit source]Hettangian
[edit | edit source]Triassic
[edit | edit source]Glen Canyon Sandstone
[edit | edit source]
The cliff-forming rocks seen here are eolian sandstones in Dinosaur National Monument, Utah. The unit is locally called the Glen Canyon Sandstone, but it's equivalent to the Navajo Sandstone (elsewhere, it's called the Nugget Sandstone). The Navajo/Nugget/Glen Canyon is a thick quartzose sandstone succession with abundant cross-bedding. Cross-bedding refers to tilted layers between horizontal layers, and forms in a one-directional current by wind or water (in this case, wind). The sandstone is part of a sand dune deposit in an ancient, vast desert, or "sand sea", technically called an "erg". The reddish-colored, partly vegetated slopes below the Glen Canyon are part of the Upper Triassic Chinle Formation, a multicolored, nonmarine unit consisting of coarse-grained siliciclastics (fluvial channel facies) and fine-grained siliciclastics (floodplain facies). Quartz-permineralized fossil wood is famously common in the Chinle Formation (for example, at Petrified Forest National Park in Arizona). Stratigraphy: Glen Canyon Sandstone (Upper Triassic-Lower Jurassic) over Chinle Formation (Upper Triassic) Locality: outcrop near Cub Creek Road, Dinosaur National Monument, northern Uintah County, northeastern Utah, USA
Chinle Formation
[edit | edit source]




From top to bottom: (youngest to oldest)
5 – Rounded tan domes of the Navajo Sandstone,
4 – layered red Kayenta Formation,
3 – cliff-forming, vertically-jointed, red Wingate Sandstone,
2 – slope-forming, purplish Chinle Formation, layered, lighter-red Moenkopi Formation, and
1 – white, layered Cutler Formation sandstone.
Picture from Glen Canyon National Recreation Area, Utah. Credit: Matt Affolter (QFL247) (talk) (Transferred by Citypeek/Original uploaded by Matt Affolter (QFL247)).{{free media}}
Sentinel Mesa in Monument Valley, Arizona, USA.
The 5 expressed geologic units:
- 5--(resistant caprock)-Shinarump Conglomerate
4--(horizontal, layered)-Moenkopi Formation
3--(cliffs)-De Chelly Sandstone-(also in Canyon De Chelly National Monument)
2--(skirts)-Organ Rock Formation- (of Utah, & northern Arizona)
1--(local regions of Mon. Vall.)-Cedar Mesa Sandstone
Primary lithology: fluvial, mudstone, siltstone, and sandstone, other lithology: paleosols.
Coordinates: 36.155°N 109.579°W.
Region: Colorado Plateau.
Extent: Utah, Colorado, New Mexico, Arizona and Nevada.
Subunits:
- Shinarump Conglomerate
- Salitral Shale
- Mesa Redondo Member
- Bluewater Creek Member
- Blue Mesa Member
- Moss Back Member
- Poleo Sandstone
- Petrified Forest Member
- Owl Rock Member
- Rock Point Member
- Church Rock Member
The stratigraphically lowest formation is the Temple Mountain Member; however, in most areas, the basal member is the Shinarump Conglomerate (Shinarump Member).[132]
The Shinarump is a braided-river system channel-deposit facies.[133] The Monitor Butte Member, which overlies the Shinarump in most areas, is an overbank (distal floodplain) facies with lacustrine deposits, overlain in western areas by the channel-deposit facies Moss Back Member. More commonly, the Monitor Butte grades into the Petrified Forest Member. The Petrified Forest is predominately overbank deposits with thin lenses of channel-deposit facies and lacustrine deposits.
The Petrified Forest Member can be divided into the Lower Petrified Forest Member and Upper Petrified Forest Member in Arizona and New Mexico, separated by the Sonsela Sandstone Bed. The Sonsela Sandstone is a braided-stream channel facies. The Petrified Forest grades into the Owl Rock Member. The Owl Rock is a marginal lacustrine to lacustrine facies possibly representing a large lake system. The upper Petrified Forest Member includes the thin but extensive Correo Sandstone Bed.[134] Finally, either the Rock Point Member or Church Rock Member overlie the Owl Rock. The two Members may be synonymous.[135] The two Members are complex heterolithic units, representing variously braided-river facies, lacustrine, and overbank deposits.
Underlies: Wingate Sandstone, Moenave Formation, Nugget Sandstone.
Overlies: Moenkopi Formation or Cutler Group.
The Chinle Formation is part of the Colorado Plateau, Basin and Range Province, and the southern section of the Interior Plains.[136]
The Chinle Formation is aged from early Late Triassic, where age correlation is based on Land Vertebrate Faunachrons, in turn based on first and last appearances of phytosaurs, with simplified stratigraphy.[137]
Sub-stage | Faunachron | Chinle Member(s) |
---|---|---|
Late Norian-Rhaetian | Apachean | [Rock Point Member/Church Rock |
Early to Middle Norian | Revueltian | Owl Rock Member
Upper Petrified Forest Member |
upper Late Carnian | Adamanian | Sonsela Sandstone
Lower Petrified Forest Moss Back Monitor Butte |
lower Late Carnian | Otischalkian | [Shinarump Conglomerate
Temple Mountain |
Asterisks (*) indicate usage by the U.S. Geological Survey. Other usages by state geological surveys.
Group rank (alphabetical – rank and formations not recognized by the USGS)[136]
- Bull Canyon Formation (NM)[138]
- Garita Creek Formation (NM)[138]
- Petrified Forest Formation (AZ, UT, NM)[139]
- Poleo Sandstone (NM)[139]
- Redonda Formation (NM)[138]
- Salitral Formation (NM*)[139]
- San Pedro Arroyo Formation (NM)[140][141]
- Santa Rosa Formation (NM)[142]
- Shinarump Conglomerate (Shinarump Formation) (NM)[139]
- Trujillo Formation (NM)[138]
- Many others – see members below
Formation rank (alphabetical – several members not recognized by the USGS):[136]
- Agua Zarca Sandstone Member (NM);[143] now considered a junior synonym for the Shinarump Conglomerate.[139]
- Bluewater Creek Member (AZ, CO, NM)[144]
- Church Rock Member (AZ*, CO*, UT*); possibly equivalent to the Rock Point Member[145]
- Correo Sandstone Member or Bed (NM)[146]
- Cuervo Sandstone Member (NM); likely preempted by Trujillo Formation[147]
- Gartra Formation (CO*, UT*)[148]
- Mesa Redondo Member (AZ*, NM*)[149]
- Monitor Butte Member (AZ*, CO*, UT*)[150][144]
- Moss Back Member (AZ*, CO*, UT*)[150]
- Owl Rock Member (AZ*, NM*, UT*)[150]
- Petrified Forest Member (AZ*, CO*, NV*, NM*, UT*)[150]
- Redonda Formation (NM)[138]
- Rock Point Formation (Rock Point Member) (AZ*, NM*)[151]
- Shinarump Conglomerate (Shinarump Member) (AZ*, NV*, NM*, UT*)[140]
- Stanaker Member (UT)[148]
- Temple Mountain Member (UT*)[152]
Geologic Province:[136]
- Rock Point Member
- Owl Rock Member
- Petrified Forest Member
- Mesa Redondo Member
- Shinarump Member
- Great Basin province*[153]
- Petrified Forest Member
- Shinarump Member
- Green River Basin*[154]
- upper member
- red siltstone member (possibly correlative with Church Rock Member)
- local sandstone and conglomerate member
- ochre siltstone member
- mottled member
- Gartra Member
- Redonda Formation
- Bull Canyon Formation
- Trujillo Formation
- Garita Creek Formation
- Orogrande Basin[140]
- San Pedro Arroyo Formation
- Shinarump Formation
- Church Rock Member
- Owl Rock Member
- Petrified Forest Member
- Moss Back Member
- Monitor Butte Member
- Shinarump Conglomerate
- upper member
- red siltstone member (possibly correlative with Church Rock Member)
- local sandstone and conglomerate member
- ochre siltstone member
- mottled member
- Gartra Member
- Rock Point Member
- Owl Rock Member
- Petrified Forest Member
- Mesa Redondo Member
- Shinarump Member
- San Juan Basin*[158]
- Rock Point Formation
- Owl Rock Formation
- Petrified Forest Formation
- Bluewater Creek/San Pedro Arroyo Formations
- Shinarump Conglomerate/Zuni Mountains Formation
- Stanaker Member
- Gartra Member
- Uinta Uplift*[148]
- Stanaker Member
- Gartra Member
- Wasatch Uplift*[146]
Parklands:
- Arches National Park
- Canyonlands National Park – see geology of the Canyonlands area
- Capitol Reef National Park – see geology of the Capitol Reef area
- Colorado National Monument
- Dinosaur National Monument
- Flaming Gorge National Recreation Area
- Glen Canyon National Recreation Area
- Gold Butte National Monument
- Grand Canyon National Park – see geology of the Grand Canyon
- Grand Staircase-Escalante National Monument
- Lake Mead National Recreation Area
- Natural Bridges National Monument
- Petrified Forest National Park
- Red Fleet State Park
- Wupatki National Monument
- Zion National Park]]– see geology of the Zion and Kolob canyons area
- Red Rock Canyon National Conservation Area
Other:
- Ghost Ranch[159]
Norian
[edit | edit source]Carnian
[edit | edit source]Ladinian
[edit | edit source]Anisian
[edit | edit source]Olenekian
[edit | edit source]Induan
[edit | edit source]In the diagram on the right, the Permian-Triassic boundary is at the base of the Induan limestone that occurs within the Yinkeng Formation.
"The Global Stratotype Section and Point (GSSP) of the Permian-Triassic boundary [...] is defined at the base of Hindeodus parvus horizon, i.e. the base of Bed 27c of Meishan section D, Changxing County, Zhejiang Province, South China."[160]
"Hindeodus parvus is now recognized as the index fossil" occurring in the Zone above the P-T boundary.[160]
Paleozoic
[edit | edit source]Permian
[edit | edit source]Changhsingian
[edit | edit source]Wuchiapingian
[edit | edit source]Capitanian
[edit | edit source]Wordian
[edit | edit source]Roadian
[edit | edit source]Kungurian
[edit | edit source]Artinskian
[edit | edit source]Sakmarian
[edit | edit source]Asselian
[edit | edit source]In the geologic timescale, the Asselian is the earliest geochronologic age or lowermost chronostratigraphic stage of the Permian, a subdivision of the Cisuralian Epoch or Series, which lasted between 298.9 and 295 million years ago (Ma), preceded by the Gzhelian (the latest or uppermost subdivision in the Carboniferous) and followed by the Sakmarian.[161]
The Artinskian still encompasses most of the lower Permian – its current definitions are more restricted. The Asselian is named after the Assel River in the southern Ural Mountains of Kazakhstan and Bashkortostan.[162]
The base of the Asselian Stage is at the same time the base of the Cisuralian Series and the Permian System, defined as the place in the stratigraphic record where fossils of the conodont Streptognathodus isolatus first appear, where the global reference profile for the base (the GSSP or golden spike) is located in the valley of the Aidaralash River, near Aqtöbe in the Ural Mountains of Kazakhstan.[163] The top of the Asselian stage (the base of the Sakmarian stage) is at the first appearance of conodont species Streptognathodus postfusus.
The Asselian contains five conodont biozones:
- zone of Streptognathodus barskovi
- zone of Streptognathodus postfusus
- zone of Streptognathodus fusus
- zone of Streptognathodus constrictus
- zone of Streptognathodus isolatus
Carboniferous
[edit | edit source]Pennsylvanian
[edit | edit source]"Specimens of Calamites cistii (Sphenophyta; Pennsylvanian, France) are described showing endophytic cavities, located in the outer cortex of the stem, a tissue that is rarely preserved. This new record shifts the appearance of this behavior back 60 Ma."[164] Two "specimens of the arborescent Calamites cistii (Sphenophyta) [were] collected from the Pennsylvanian basin of Graissessac (Hérault, France)".[164] "The specimens belong to the species Calamites cistii Brongniart, 1828 (Sphenophyta). They are housed in the Collections de Paléobotanique, Service général des Collections, University Montpellier 2 (LPM)."[164]
The Pennsylvanian lasted from 318.1 ± 1.3 to 299.0 ± 0.8 Mb2k.
Gzhelian
[edit | edit source]
The Gzhelian is an age in the International Commission on Stratigraphy (ICS) geologic timescale or a stage in the stratigraphic column, the youngest stage of the Pennsylvanian, the youngest subsystem of the Carboniferous. The Gzhelian lasted from 303.7 to 298.9 Ma.[165] It follows the Kasimovian age/stage and is followed by the Asselian age/stage, the oldest subdivision of the Permian system.
The Gzhelian is more or less coeval with the Stephanian Stage of the regional stratigraphy of Europe.
The base of the Gzhelian is at the first appearance of the Fusulinida genera Daixina, Jigulites and Rugosofusulina, or at the first appearance of the conodont Streptognathodus zethus. The top of the stage (the base of the Permian system) is at the first appearance of the conodont Streptognathodus isolatus within the Streptognathus "wabaunsensis" chronocline.[166] Six meters higher in the reference profile, the Fusulinida species Sphaeroschwagerina vulgaris aktjubensis appears.
A Global Boundary Stratotype Section and Point (golden spike) for the Gzhelian Stage is yet lacking. A candidate is a section along the Ussolka river (a tributary of the Belaya river) at the edge of the hamlet of Krasnoussolsky, about 120 kilometres south-east of Ufa and 60 kilometres north-east of Sterlitamak (in Bashkortostan).[167]
The Gzhelian Stage is subdivided into five biozones, based on the conodont genus Streptognathodus:
- Streptognathodus wabaunsensis and Streptognathodus bellus Zone
- Streptognathodus simplex Zone
- Streptognathodus virgilicus Zone
- Streptognathodus vitali Zone
- Streptognathodus simulator Zone
Kasimovian
[edit | edit source]Moscovian
[edit | edit source]Bashkirian
[edit | edit source]Mississippian
[edit | edit source]Serpukhovian
[edit | edit source]Viséan
[edit | edit source]"The first appearance of Eoparastaffella simplex in the lineage Eoparastaffela ovalis - Eoparastaffella simplex (foraminifers) [is] the new biostratigraphic criterion to define the base of the Viséan."[168]
Tournaisian
[edit | edit source]"The base of the Carboniferous System, Mississippian Sub-System and Tournaisian Stage is defined at the base of Bed 89 in Trench E' at La Serre, France. It coincides with the first appearance of the conodont Siphonodella sulcata within the evolutionary lineage from Siphonodella praesulcata to Siphonodella sulcata."[169]
Devonian
[edit | edit source]Beaverhill Lake Group
[edit | edit source]- Stratigraphic range: Middle to Late Devonian ~390–365 Ma (late Givetian to Frasnian) in the Western Canada Sedimentary Basin that is present in the southwestern Northwest Territories, northeastern British Columbia and Alberta.[170][171]
The Beaverhill Lake Group is present beneath the plains of the southwestern Northwest Territories, northeastern British Columbia and Alberta.[171] It reaches a maximum thickness of about 220 metres (720 ft) in central Alberta.[170] Outcrops of one of its formations (the Waterways) can be seen along the Athabasca and Clearwater River in the Fort McMurray area.[172]
Sub-unit | Geochronology (Age) | Lithology | Max Thickness |
Reference |
---|---|---|---|---|
Waterways Formation, Mildred Member | Late Devonian | argillaceous limestone and shale | 42.7 m (140 ft) | [170] |
Waterways Formation, Moberly Member | Middle Devonian to Late Devonian | grey, fine-grained, thin bedded limestone, fossiliferous limestone | 95.7 m (310 ft) | [170] |
Waterways Formation, Christina Member | Middle Devonian to Late Devonian | calcareous shale and argillaceous minerals|argillaceous limestone with brachiopods | 27.4 m (90 ft) | [170] |
Waterways Formation, Calmut Member | Middle Devonian to Late Devonian | argillaceous limestone with olive green shale and brachiopods | 31.1 m (100 ft) | [170] |
Waterways Formation, Firebag Member | Middle Devonian to Late Devonian | calcareous shale and minor argillaceous limestone with brachiopods | 61 m (200 ft) | |
Slave Point Formation | Middle Devonian | dolomite, minor shale laminae | 120 m (390 ft) | [170] |
Fort Vermilion Formation | Middle Devonian | dolomite or limestone | 37 m (120 ft) | [170] |
- Swan Hills, Alberta|Swan Hills area
Sub-unit | Age | Lithology | Max Thickness |
Reference |
---|---|---|---|---|
Swan Hills Formation | Middle Devonian to Late Devonian | stromatoporoid reef (micritic and pelletoidal limestone facies or coarse, porous, bioclastic limestone facies) | 152 m (500 ft) | [170] |
Waterways Formation | Middle Devonian to Late Devonian | nodular and argillaceous minerals|argillaceous limestone and shale with brachiopods, corals and ostracods | 230 m (750 ft) | [170] |
Fort Vermilion Formation | Middle Devonian | dolomite or limestone | 8 m (30 ft) | [170] |
Swan Hills Formation
[edit | edit source]Petroleum is produced from the Swan Hills Formation of the Beaverhill Lake Group in the Swan Hills area of northern Alberta.[173][174]
Waterways Formation
[edit | edit source]Slave Point Formation
[edit | edit source]Fort Vermilion Formation
[edit | edit source]Imperial Formation
[edit | edit source]The Imperial Formation occurs in the Northwest Territories of Canada, preserves fossils dating back to the Devonian period.[175]
Famennian
[edit | edit source]
"The boundary for the Frasnian/Famennian Stage Global Stratotype Section and Point (GSSP) [...] is drawn [above] in a section exposed [in the second image above] near the Upper Coumiac Quarry in the southeastern Montagne Noire, France."[176]
A specimen of Clymenia laevigata from the Upper Devonian Famennian of Poland is on the right.
On the left is a fossil of Platyclymenia intracrostata also from the Famennian of Poland.
Frasnian
[edit | edit source]Givetian
[edit | edit source]Eifelian
[edit | edit source]Emsian
[edit | edit source]Pragian
[edit | edit source]Lochkovian
[edit | edit source]Silurian
[edit | edit source]Přídolí
[edit | edit source]Ludfordian
[edit | edit source]Gorstian
[edit | edit source]Homerian
[edit | edit source]Sheinwoodian
[edit | edit source]Telychian
[edit | edit source]On the right is an image of the type locality for the Telychian base GSSP indicated by an arrow which points parallel to the bedding. Older bedding of the Aeronian is to the right. The Telychian GSSP is in the Wormwood Formation, Cefn Cerig quarry.
In the section below for the Aeronian, the lower Telychian is marked with a Ⓣ.
Aeronian
[edit | edit source]The diagram above has the GSSP for the base of the Aeronian symbolized by a Ⓐ. The upper GSSP for the end of the Aeronian is symbolized by a Ⓣ.
On the right is the type locality for the base of the Aeronian indicated by the arrow. Actual beds are perpendicular to the arrow. The base of the Aeronian is in the Cefngarreg Sandstone Formation (formerly Trefawr Formation), Trefawr track section, Crychan Forest, Central Wales.
Rhuddanian
[edit | edit source]Ordovician
[edit | edit source]Hirnantian
[edit | edit source]Katian
[edit | edit source]Sandbian
[edit | edit source]"The Lower Sandbian Nemagraptus gracilis Zone comprises one of the most widespread, and easily recognizable graptolite faunas in the Ordovician System. The base of the N. gracilis Zone also marks the base of the Upper Ordovician Series, and is internationally defined by the FAD of the eponymous species, with the Global Stratotype Section and Point (GSSP) located at Fågelsång in Scania, southern Sweden (Bergström et al., 2000, 2009)."[177]
Darriwilian
[edit | edit source]Floian
[edit | edit source]Green Point Formation
[edit | edit source]Ordovician.
Region: Newfoundland and Labrador.
The GSSP for the beginning of the Tremadocian is the Greenpoint section (49.6829°N 57.9653°W) in Gros Morne National Park, in western Newfoundland, found 101.8 m above the Greenpoint section datum within bed number 23.[178] The boundary lies within the Broom Point Member, of the Green Point Formation which is part of the Cow Head Group.[179] The first planktonic graptolites appear 4.8 m above the first appearance of Iapetognathus fluctivagus at Greenpoint section.[179]
It preserves fossils dating back to the Ordovician period.
Crystal Mountain Sandstone
[edit | edit source]Ordovician
Region: Arkansas, Oklahoma.
Underlies: Mazarn Shale.
Overlies: Collier Shale.
Thickness: 500 to 800 feet[180]
El Paso Formation
[edit | edit source]Lower Ordovician.
Nittany Dolomite
[edit | edit source]Ordovician.
Underlies: Axeman Limestone.
Overlies: Stonehenge Limestone.
Region: Pennsylvania.
Stonehenge Limestone
[edit | edit source]Lower Ordovician.
Underlies: Nittany Dolomite.
Overlies: Mines Dolomite.
Extent: Maryland, Pennsylvania, and Virginia[181]
Mines Dolomite
[edit | edit source]Underlies: Stonehenge Limestone.
Gatesburg Formation
[edit | edit source]Cambrian.
Underlies: Mines Dolomite.
Overlies: Warrior Formation.
Region: Pennsylvania.
The Gatesburg Formation is in Pennsylvania, preserves fossils dating back to the Cambrian, underlies the Mines Dolomite and overlies the Warrior Formation.
Middle Chesley Drive Group
[edit | edit source]Late Upper Cambrian.
MacNeil Brook, Nova Scotia, Canada. North shore of East Bay, near Eskasoni, Cape Breton Island, Nova Scotia.
White Leaved Oak Shale
[edit | edit source]Late Upper Cambrian.
Malvern, Gloucestershire, England.
Cwmhesgen Formation
[edit | edit source]Late Upper Cambrian.
Wales.
Léwis Formation
[edit | edit source]Late Upper Cambrian.
North Ridge, near Léwis, Quebec.
Siyangshan Formation
[edit | edit source]Late Upper Cambrian.
western Zhejiang.
Shallow Bay Formation
[edit | edit source]Late Upper Cambrian.
western Newfoundland.
Pogonip Limestone
[edit | edit source]Late Upper Cambrian.
United States.
Windfall Formation
[edit | edit source]late Upper Cambrian
near Hamburg Shaft, Eureka District, Nevada.
Trempealeau Formation
[edit | edit source]Upper Cambrian.[182]
The Trempealeau Formation, named for the town of Trempealeau in western Wisconsin, is located on the Mississippi River.
Abrigo Formationn
[edit | edit source]Upper Cambrian.
Bliss Sandstone
[edit | edit source]Upper Cambrian.
Underlies: El Paso Formation.
Warrior Formation
[edit | edit source]Cambrian
Underlies: Gatesburg Formation.
Overlies: Pleasant Hill Formation.
Extent: Bedford County, Blair County, Centre County, Huntingdon County, Pennsylvania.[183]
Thickness: 250 ft at type sections,[184] 1350 ft in Nittany Arch[185]
The Warrior Formation is described as gray, thin- to medium-bedded, fossiliferous, cyclic limestone bearing stromatolites, interbedded with shale, siltstone, and sandstone.[186]
Thickness: 250 ft at type sections,[184] 1350 ft in Nittany Arch[185]
Extent: Bedford County, Pennsylvania, Blair County, Pennsylvania, Centre County, Pennsylvania, Huntingdon County, Pennsylvania.[187]
Notable Exposures:
- Type section: Warrior Run, 1 mile east of Williamsburg, Pennsylvania, Blair County, Pennsylvania
- Warrior Creek, east of Warriors Mark Township, Huntingdon County, Pennsylvania, Huntingdon County, Pennsylvania
- Section near Waddle, Pennsylvania.[188]
Age: Relative age dating places the Warrior Formation in the middle to late Cambrian.
Pleasant Hill Limestone (Formation)
[edit | edit source]Cambrian
Underlies: Warrior Limestone.
Overlies: Waynesboro Formation.
Region: Pennsylvania.
Alum Shale Formation
[edit | edit source]Coordinates: 55°42′58″N, 13°59′00″E.
Middle Cambrian-Lower Ordovician
Country: Sweden, Denmark, Norway.
A "radiometrically anchored astrochronologic framework across the late Cambrian interval, using high-resolution aluminum (Al) series (1 mm resolution) through the Alum Shale Formation in Scania, southernmost Sweden, [is] based on the fully cored Albjära-1 well. Significant cycles with periods of 405 kyr (long eccentricity), 108 kyr (short eccentricity), 30.4 kyr (obliquity) and 18.8 kyr (precession), associated with long-term amplitude modulation of obliquity and precession, confirmed the orbital imprint on late Cambrian climate. Using the U-Pb dating at 486.78±0.53Ma for the Cambro-Ordovician boundary as anchor point, our timescale spans from ~483.9 to ~500.0 Ma, covering 7 trilobite superzones and 3 graptolite zones. The calibration indicates ages of 491.2±0.54 Ma, 493.9±0.67 Ma, 497.3±0.67 Ma and 500.4±0.67 Ma for the lower boundaries of provisional Stage10, Jiangshanian, Paibian and Guzhangian stages, respectively."[189]
Potosi Dolomite
[edit | edit source]Cambrian
Region: Illinois, Indiana, Missouri.
Underlies: Eminence Dolomite and Oneota Dolomite.
Overlies: Davis Formation,[190] Derby-Doerun Dolomite,[191] and Franconia Formation.
Coordinates: 38.091733°N 90.685176°W.
Derby-Doerun Dolomite
[edit | edit source]Upper Cambrian
Region: St. Francois County, Missouri.
Unit of Elvins Group.
Underlies: Potosi Dolomite
Overlies: Davis Formation[192]
Jordan Sandstone
[edit | edit source]Cambrian.
Underlies: Oneota Dolomite.
Overlies: St. Lawrence Formation.
Region: Illinois, Minnesota, and Wisconsin.
St. Lawrence Formation
[edit | edit source]Franconia Formation
[edit | edit source]Cambrian.
Unit of Munising Group (IN,MI, WI), Knox Group (IL), Tunnel City Group (IA,MN,WI).
Sub-units: Birkmose Member (MO), Davis Member (IL), Derby-Doe Run Member (IL), Ironton Member (IL,WI,MI), Mazomanie Member (WI), Reno Member (MO,MN,WI), Tomah Member (MO).
Underlies: Potosi Dolomite and St. Lawrence Formation.
Overlies: Ironton Sandstone.
Region: Illinois, Indiana, Iowa, Michigan, Minnesota, Missouri, Ohio, and Wisconsin.
The Franconia Formation is about 100 feet (30 m) of sandstone and green shale exposed near the town of Franconia in eastern Minnesota, north of St Paul.
Ironton Sandstone
[edit | edit source]Cambrian.
Unit of Munising Group.
Underlies: Franconia Formation.
Overlies: Galesville Sandstone.
Region: Indiana.
Collier Shale
[edit | edit source]Late Cambrian-Early Ordovician.
Underlies: Crystal Mountain Sandstone.
Region: Arkansas, Oklahoma.
The Collier Shale, a geologic formation in the Ouachita Mountains of Arkansas and Oklahoma, dating from the Late Cambrian to Early Ordovician periods, the oldest stratigraphic unit exposed in Arkansas, first described in 1892,[193] named in 1909,[194][195] with assigned type locality to the headwaters of Collier Creek in Montgomery County, Arkansas, underlies the Crystal Mountain Sandstone.
Ogon’or Formation
[edit | edit source]late Upper Cambrian
The Aksayan Stage of the Ogon’or Formation, upper part.[196]
along the Khos-Negele River, Siberia.
Huayansi Formation
[edit | edit source]The GSSP for the Paibian is defined in the "Paibi section" (Wuling Mountains, Huayuan County), an outcrop of the Huaqiao Formation. The base is the first occurrence of Glyptagnostus reticulatus which is 396 m above the base of the Huaqiao Formation.
Huayansi Formation.[197]
Bolza Quartzite
[edit | edit source]Middle to Lower Cambrian.
"Lochman-Balk (1971) and Hayes (1975) tended to favor interpreting the Bliss and correlative Coronado Sandstone and Bolsa Quartzite, to the west, as beach deposits."[198]
Stephen Formation
[edit | edit source]The Stephen Formation (51.46425°N 116.32443°W) is exposed in the Canadian Rockies of British Columbia and Alberta, on the western edge of the Western Canada Sedimentary Basin, consisting of shale, thin-bedded limestone, and siltstone that was deposited during Middle Cambrian time (513 to 497 Ma),[199] famous for the lagerstätte (exceptional preservation) of soft-bodied fossils: the Burgess Shale biota.[200][201]
The formation overlies the Cathedral escarpment, a submarine cliff; consequently it is divided into two quite separate parts, the 'thin' sequence deposited in the shallower waters atop the escarpment, and the 'thick' sequence deposited in the deeper waters beyond the cliff. Because the 'thick' Stephen Formation represents a distinct lithofacies, some authors suggest it warrants its own name, and dub it the Burgess Shale Formation.[202].
Huaqiao Formation
[edit | edit source]"The Global boundary Stratotype Section and Point (GSSP) for the base of the Guzhangian Stage (Cambrian Series 3) is defined at the base of a limestone (calcisiltite) layer 121.3 m above the base of the Huaqiao Formation in the Louyixi section along the Youshui River (Fengtan Reservoir), about 4 km northwest of Luoyixi (4 km southeast of Wangcun), in northwestern Hunan, China."[203]
Huaqiao Formation is in Hunan at (28.4° N, 109.5° E).[204]
Eldon Formation
[edit | edit source]
Cambrian ~509-500 Ma.
The Eldon Formation (51°18'8.5°N 115°55'45"W) ~509-500 Ma present on the western edge of the Western Canada Sedimentary Basin in the southern Canadian Rockies of southwestern Alberta and southeastern British Columbia,[201] is a thick sequence of massive, cliff-forming limestones and dolomites,[170][205] deposited during Middle Cambrian time, and it includes fossil stromatolites.[170] The Eldon forms the scenic cliffs at the top of Castle Mountain, and can also be seen at Mount Yamnuska and other mountains in Banff National Park and Yoho National Parks.[206]
The Eldon Formation was deposited during the Middle Cambrian, originally formed as limestone and calcareous mudstone in the intertidal to supratidal zone along the western margin of the Laurentia (North American Craton),[201][207] subsequently by dolomitization altered to dolomite and dolomitic mudstone in some areas.[170]
The Eldon Formation reaches a maximum thickness of about 500 metres (1,640 feet) at Mount Bosworth on the Alberta-British Columbia border conformably overlies the Stephen Formation, which hosts the fossils of the Burgess shale, in the south, and the Snake Indian Formation in the north, is conformably overlain by the Pika Formation, grades into the Earlie Formation to the east, the Chancellor Formation to the west, and the Titkana Formation to the north. It is probably equivalent to the Windsor Mountain Formation to the south.[170][201][208]
Bright Angel Shale
[edit | edit source]
The three units of the Tonto Group and the colorful Bright Angel Shale are easily identified as a geological sequence beneath the tall cliffs of the Redwall Limestone (the Redwall sits upon a short resistant cliff of Muav Limestone); the Tonto Group is also easily seen beside Granite Gorge of the Colorado River and the Vishnu Basement Rocks
The units of the Tonto Group:[209]
- Redwall Limestone
- Temple Butte Formation, Devonian – (409–363 Ma), channel deposits upon Muav Limestone
- Tonto Group (3 units) (~544–505 Ma)
The eastern version of the Pioche shale can be found eastwards in the Grand Canyon, as the Bright Angel Shale.[212]
The Cambrian Bright Angel Shale is the middle layer of the three member Tonto Group geologic feature. The 3-rock Tonto section famously sits upon the Great Unconformity because of the highly resistant cliffs of the base layer, vertical Tapeats Sandstone cliffs.
The Bright Angel Shale is easily identified for two reasons: its soft-greenish color stands out against the browns, reds, and whites of neighboring rock units, and its slope-forming character against mostly cliff-forming resistant rocks.
The Bright Angel Shale is about 500 feet (152 m) thick at its maximum.[209] It is a nonresistant slope-forming unit. The Bright Angel Shale consists of green and purple-red, siltstone and shale which is interbedded with red-brown to brown sandstone that is similar in lithology to the underlying Tapeats.[213] The Bright Angel Shale underlies and interfingers with Muav Limestone. The Bright Angel Shale is located in the lower elevations of the Grand Canyon, Arizona.[214] The Bright Angel Shale preserves fossils dating back to the Cambrian.[215]
Pioche Shale
[edit | edit source]The Pioche Shale is an Early to Middle Cambrian Burgess shale-type Lagerstätte in Nevada.[216]
It spans the Early–Middle Cambrian boundary; fossils from the Early Cambrian are preserved in botryoidal hematite, whereas those from the Middle Cambrian are preserved in the more familiar carbon films, and very reminiscent of the Chengjiang County preservation.[216]
It preserves arthropods and worms familiar from the Burgess Shale.[217]
It spans the early Cambrian Olenellus and basal Middle Cambrian Eokochaspis nodosa trilobite zones.[217]
Eokochaspis zone
Lower-Middle Cambrian Boundary Interval.[218]
The lower Middle Cambrian[219]
Langston Formation
[edit | edit source]Cambrian.
Underlies: Ute Formation.
Overlies: Brigham Formation.
Thickness: 30 - 498'.
Region: Idaho, Utah.
Spence Shale
[edit | edit source]
(~507.5-506 Ma)
The Spence Shale, Wuliuan, ~507.5-506 Ma, the middle member of the Langston Formation in southeastern Idaho and northeastern Utah, exposed in the Bear River Range, the Wasatch Range and the Wellsville Mountains, is known for its abundant Cambrian trilobites and the preservation of Burgess Shale-type fossils,[220] type locality: Spence Gulch in southeastern Idaho, near the town of Liberty, first described in 1908,[205] spans the Albertella and Glossopleura biozones.[220]
- Glossopleura-zone
- Albertella-zone
Sonoraspis and Albertella[221]
Wheeler Shale
[edit | edit source]Middle Cambrian ~507 Ma.
Thickness: 100–200 m (330–660 ft).
Coordinates: 39.25°N 113.33°W.
Region: House Range and Drum Mountains, Millard Co., west Utah.
The "FAD of Ptychagnostus atavus [is the primary stratigraphic tool for correlation of the base (GSSP)] for the Drumian Stage".[203]
"The Global boundary Stratotype Section and Point (GSSP) for the base of the Drumian Stage (Cambrian Series 3) is defined at the base of a limestone (calcisiltite) layer 62 m above the base of the Wheeler Formation in the Stratotype Ridge section, Drum Mountains, Utah, USA. The GSSP level contains the lowest occurrence of the cosmopolitan agnostoid trilobite Ptychagnostus atavus (base of the P. atavus Zone)."[222]
"The polymerid trilobites Ptychoparella (incorporating Elrathina as a junior synonym) and Elrathia have long stratigraphic ranges (Robison, 1964a, 1964b, 1976; Babcock, 1994a) that extend from stage 5 into the lower part of the Drumian Stage (White, 1973) and provide little help in constraining the base of the Drumian."[222]
On the right are images of key agnostoid trilobite species used for recognition of the base of the Drumian Stage.
"A, Ptychagnostus gibbus (Linnarsson), dorsal exoskeleton in shale, x 8.4, from the Wheeler Formation, c. 25 m above base, south side of Swasey Peak, House Range, Utah (R. A. Robison locality 157); KUMIP 153949. B, Ptychagnostus atavus (Tullberg), cephalon in limestone showing scrobiculate genae, x 8.1, from the Wheeler Formation, 27 m above base, House Range, Utah (R. A. Robison locality 196); KUMIP 153830. C, P. atavus (Tullberg), pygidium in limestone, x 7.8, from same locality as specimen in Figure 6B; KUMIP 153933. D, P. atavus (Tullberg), dorsal exoskeleton from shale with cone-in-cone calcite encrusting ventral surface, x 8.1 from the Wheeler Formation, c. 100 below top, “Swasey Spring quarry”, east flank of House Range, Utah (R. A. Robison locality 114); KUMIP 153930."[222]
The "Cambrian lobopodian (panarthropod) worm Hallucigenia sparsa [is] from the Burgess Shale (Cambrian Series 3, Stage 5)."[223]
Burgess Shale
[edit | edit source]
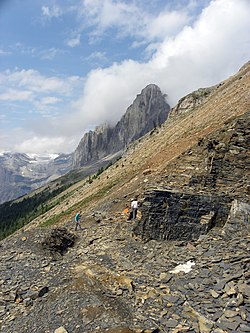
Def. a "rock formation in the Canadian Rockies that contains very many fossils from the Cambrian period"[224] is called the Burgess Shale.
The Burgess Shale is a fossil-bearing deposit exposed in the Canadian Rockies of British Columbia, Canada.[225][226] It is famous for the exceptional preservation of the soft parts of its fossils. At 508 Ma (Wuliuan of the middle Cambrian),[227] it is one of the earliest fossil beds containing soft-part imprints.
(~508 Ma)
Bathyuriscus–Elrathina-zone Middle Cambrian
Contemporaneous with the Burgess Shale
Oryctocephalus indicus-zone underlies Burgess Shale.
Ust’-Sema Formation
[edit | edit source]Barangol Formation
[edit | edit source]"The Lower Cambrian carbonate sequence ends with the 160-m-thick Upper Toyonian Barangol Formation, the age which is based on calcareous algae, archaeocyatids and trilobites (Zybin et al., 2000). It is uncorformably overlain by the Ust’-Sema Formation, a 1,000-m-thick basaltic sequence displaying thick conglomerates at its base, containing blocks of limestones with a similar fauna to the one identified in the Cheposh Formation (Zybin et al., 2000)."[228]
Stage 4
[edit | edit source]Cambrian ~514 – ~509 Ma.
Stage 3
[edit | edit source]Cambrian ~521 – ~514 Ma.
Stage 2
[edit | edit source]Cambrian: ~529 – ~521 Ma.
"Hallucigeniids are [...] an important and widespread component of disparate Cambrian communities from late in the Terreneuvian (Cambrian Stage 2) through the ‘middle’ Cambrian (Series 3); their apparent decline in the latest Cambrian may be partly taphonomic. The cone-in-cone construction of hallucigeniid sclerites is shared with the sclerotized cuticular structures (jaws and claws) in modern onychophorans."[223]
In the image on the right "Hallucigenia sparsa [is] from the Burgess Shale: (a,b) Smithsonian Institution, National Museum of Natural History (NMNH) 83935 (holotype), articulated specimen, showing seven pairs of spines, partially decayed towards the rear, presumed head to the right. (a) composite image of part and counterpart; (b) enlargement of the basal part of the spines; (c,d) Royal Ontario Museum (ROM) 61513, complete specimen showing seven pairs of spines and backscatter image of boxed area (d); (e–i) ROM 57776, backscatter images (overview and close-ups of boxed areas) of spine showing four internal cones and lineations; (g) ROM 61513, backscatter image showing lineations and a distal cone; (j–o) ROM 62269, backscatter images of several spines, showing elemental distribution of carbon (l) and phosphorous (m) and details of ornamentation near spines’ mid-length (n) and base (o) (arrows indicate local disturbances in the rhomboid pattern); (p) ROM 61513, backscatter image showing details of ornamentation showing scales in positive relief (top left) and negative relief below the carbon film. Ba, basal region of spines; C, cone; Li, lineations. Scale bars: (a–d) 1000 µm; (e,j–m) 100 µm; (f–i) 50 µm; (n–p) 10 µm."[223]
Zhujiaqing Formation
[edit | edit source]Yunnan, China.
Pestrotsvet Formation
[edit | edit source]Near the Aldan River on the Siberian Platform.
Conasauga Formation
[edit | edit source]Cambrian.
Underlies: Copper Ridge Dolomite, Kerbel Formation, and Knox Dolomite.
Overlies: Rome Formation
Region: Appalachia and Southeastern United States.
Extent: Georgia, Mississippi, Ohio, and Virginia[229]
Rome Formation
[edit | edit source]

Middle Cambrian-Upper Ordovician.
Region: Appalachia, Midwestern United States and Southeastern United States.
Underlies: Conasauga Formation.
Overlies: Mount Simon Sandstone and Shady Dolomite.
Extent: Mississippi, Ohio, Tennessee, and Virginia.[230]
The prominent linear feature from upper left to lower right is the Copper Creek Thrust Fault in Tennessee. Thrust faults are low-angle reverse faults, formed by compressional stress. They are common in mountain belts formed by tectonic collision - this example is in the Appalachian Mountains (= result of a collision between Africa and North America during the Pennsylvanian). The folded rocks above the fault are part of the Rome Formation (Middle Cambrian). The tilted rocks below the fault at lower left are Moccasin Formation limestones (Middle Ordovician). Locality: roadcut along the eastern side of Rt. 25E, just south of the Clinch River, north of Clinch Mountain, far-northern Grainger County, northeastern Tennessee, USA (36° 22' 54.64" North latitude, 83° 26' 48.86" West longitude).
Elbrook Formation
[edit | edit source]Cambrian.
Region: Appalachia and Southeastern United States.
Underlies: Conococheague Formation.
Overlies: Waynesboro Formation.
Region: Appalachia and Southeastern United States.
Extent: Virginia[231] and West Virginia.
Shady Dolomite
[edit | edit source]Cambrian Series 2: 521-509 Ma.
Underlies: Rome Formation, Elbrook Formation.
Overlies: Weisner Quartzite, Chilhowee Group.
Region: Appalachia and Southeastern United States.
The Shady Dolomite is composed of marine sedimentary rocks of early Cambrian age. It outcrops along the eastern margin of the Blue Ridge Mountains province in the southeastern United States and can be found in outcrops in the states of Alabama, Georgia, Tennessee, North Carolina, and Virginia. It is also can be found in the subsurface of Kentucky, Ohio, and West Virginia. The Shady is predominantly composed of dolostone and limestone with lesser amounts of mudrock. It contains fossils of trilobites, Archaeocyatha (archaeocyathids), algae, brachiopods, and echinoderms, along with the enigmatic fossil Salterella.[232][233][234][235][236][237] The Shady Dolomite was first described in 1903[238] and was named for exposures in the Shady Valley of Johnson County in the state of Tennessee. Near Austinville, Virginia, the Shady hosts ore deposits that have been mined extensively for lead and zinc ore.[239]
The Shady Dolomite was deposited on the margin of the paleocontinent of Laurentia. The rocks that make up the Shady represent sediment deposition on and around an ancient carbonate platform.[240][241][242]
The initial carbonate sedimentation of the Shady began on the southern shelf of Laurentia and formed on top of the siliciclastic sequence that now make up the rocks of the Chilhowee Group.[243] These carbonate sediments formed a carbonate ramp that over time developed into a rimmed carbonate shelf.[241][242]
Weisner Quartzite
[edit | edit source]Weisner Quartzite is a member of the Chilhowee Group.
Poleta Formation
[edit | edit source]The Poleta Formation occurs in Esmeralda County, Nevada, USA.[244]
Sekwi Formation
[edit | edit source]Cambrian.
The Sekwi Formation occurs in the Northwest Territories, Canada.[245][246]
Mural Formation
[edit | edit source]The Mural Formation occurs in the north slope of Mount Mumm, Alberta, Canada.[247]
Mahto Formation
[edit | edit source]The Mahto Formation occurs at Mumm Peak on the west side of Hitka Pass, western Alberta, Canada.[248]
Nagaur Group
[edit | edit source]Ediacaran - Cambrian Boundary.
The FAD of Trichophycus pedum, earliest appearance, around 542 Ma,[249] which was contemporaneous with the last of the Ediacaran biota, is used to help define the dividing line, considered geologically at 541 Ma, between the Ediacaran and Cambrian.[250][251] It is last seen in the fossil record during the Cenomanian (99.7 Ma).[252]
Manzherok Formation
[edit | edit source]Lower Cambrian.
"The Manzherok Formation is essentially a thick (up to 1,250 m) sequence of Lower Cambrian basaltic lavas that overly unconformably the Baratal Formation. Blocks of brecciated silicified carbonate rocks which reflect accumulation in a slope depositional environment are present in places. They contain algae, microphytoliths and sponge spicules (Safonova et al., 2011, Zybin et al., 2000)."[228]
Eskongo Formation
[edit | edit source]Vendian-Early Cambrian.
"The Eskongo Formation contains microphytolites, calcareous algae and shelly microfauna characteristic of a Vendian-Early Cambrian age (Terleev, 1991). A lot of sponge spicules (Protospongia sp. and Chancelloria sp. and specimens of Monaxonellida, Hexactinellida and Tetraxonida) were also identified in the siliceous levels of this Formation (Zybin et al., 2000)."[228]
Mount Simon Sandstone
[edit | edit source]Basal unit of Potsdam Sandstone.
Underlies: Eau Claire Formation and Rome Formation
Overlies: Hinckley Sandstone and Middle Run Formation
Thickness: up to 2,000 feet (610 m).
Extent: Illinois, Indiana, Kentucky, Michigan, Minnesota, Ohio, and Wisconsin.
Mount Simon Sandstone was deposited in a nearshore environment, unconformably overlying Precambrian basement.[253]
Precambrian
[edit | edit source]Baratal Formation
[edit | edit source]Vendian.
The "Baratal Formation [is] made essentially of thick-bedded partly stromatolitc limestones".[228]
The "Baratal Formation contains microphytolites of a Vendian age (Buslov et al., 1993, Zybin and Sergeev, 1978)."[228]
Hinckley Sandstone
[edit | edit source]Middle Run Formation
[edit | edit source]Precambrian
Underlies: Mount Simon Sandstone.
Overlies: "East Granite-Rhyolite Province igneous and volcanic rocks".
Extent: Indiana and Ohio.
After drilling through Mt. Simon sandstone, past the 3,500 ft mark, there was more sandstone, which is the Middle Run Formation. Drilling reached a depth of 5,370 ft of this same formation before the drill bit was stuck—without reaching the Precambrian basement rocks.[254]
Ediacaran
[edit | edit source]"In the central Flinders Ranges the 4.5 km thick Umberatana Group encompasses the two main phases of glacial deposition (see Thomas et al., 2012). The carbonaceous, calcareous and pyritic Tindelpina Shale Member, of the interglacial Tapley Hill Formation, caps the Fe-rich diamictite and tillite formations of the Sturt glaciation. The upper Cryogenian glacials of the Elatina Formation are truncated by the Nuccaleena Formation at the base of the Wilpena Group and the Ediacaran System."[255]
"In 2004, the Global Stratotype Section and Point (GSSP) for the terminal Proterozoic was placed near the base of the Nuccaleena Formation in Enorama Creek in the central Flinders Ranges [in the image on the right], thus establishing the Ediacaran System and Period (Knoll et al., 2006). As the Nuccaleena Formation has not been accurately dated, a date of c. 635 Ma from near-correlative levels in Namibia and China is presumed for the base of the Ediacaran (Hoffmann et al., 2004; Condon et al., 2005; Zhang et al., 2005)."[255]
Cryogenian
[edit | edit source]The Cryogenian is a geologic period that lasted from 635 to 720 Ma.[256]
Tonian
[edit | edit source]Stenian
[edit | edit source]Apache Group
[edit | edit source]Troy Quartzite
[edit | edit source]Mescal Limestone
[edit | edit source]Dripping Spring Quartzite
[edit | edit source]~1,400 Ma (1.4 Ba).
Underlies: Mescal Limestone.
Overlies: Pioneer Shale.
Thickness: 620 feet (189 m) max.[257]
Extent: central Arizona transition zone-(Sierra Ancha range, Globe, Arizona region, and south to Catalina Mountains, northeast regions.
Barnes Conglomerate
[edit | edit source]Pioneer Shale
[edit | edit source]Scanlon Conglomerate
[edit | edit source]Ectasian
[edit | edit source]Calymmian
[edit | edit source]The Calymmian Period is the first geologic period in the Mesoproterozoic Era and lasted from 1600 Mya to 1400 Mya.
Kombolgie Formation
[edit | edit source]"The 1400 to 1500 My old Kombolgie Formation [Carpentarian] of the MacArthur Basin of the Northern Territory overlies or has overlain unconformity-type uranium deposits".[258]
McMinn Formation
[edit | edit source]"A study of clay minerals isolated from carbonate rocks of the McMinn Formation provides an isochron age of 1429 ± 31 Ma and thus the age for cessation of the Proterozoic McArthur basin sedimentation."[259]
Upper Barney Creek Formation
[edit | edit source]"A second sequence of measurements on a homogeneous dolomite siltstone ca. 23 km southeast of the McArthur River (H.Y.C.) Pb-Zn deposits yields an Rb-Sr age of 1537 ± 52 Ma for the Upper Barney Creek Formation in broad agreement with recent age determinations on feldspar beds of this horizon."[259]
Bungle Bungle Dolomite
[edit | edit source]Zoroaster Granite
[edit | edit source]
"Mafic to intermediate-composition plutons intruded the supracrustal rocks at 1741 ± 1 Ma (Zoroaster granite), 1737 ± 1 Ma (Grapevine Camp granite), 1730 ± 3 Ma (Trinity granodiorite), 1717 ± 1 Ma (Ruby granodiorite), and 1713 ± 2 Ma (Horn diorite)."[260]
"Zircons from the Zoroaster granite are colorless, euhedral crystals that are doubly terminated and prismatic (aspect ratios = 2–3). U-Pb data for five single-crystal zircon fractions [...] ranged from concordant to 3.6% normally discordant and yielded an upper-intercept age of 1741 ± 1 Ma (lower intercept = 133 ± 100 Ma; MSWD = 0.79), [interpreted as] the crystallization age of the Zoroaster pluton."[260]
Statherian
[edit | edit source]Belcher Group
[edit | edit source]The "isotope geochemistry of sulfate minerals from the Belcher Group, in subarctic Canada, [...] record ambient sulfate in the immediate aftermath of the GOE (ca. 2,018 Ma). These sulfate minerals captured negative triple-oxygen isotope anomalies as low as ∼ −0.8‰. Such negative values occurring shortly after the [Great Oxidation Event (ca. 2,400 to 2,050 Ma)] GOE require a rapid reduction in primary productivity of >80%, although even larger reductions are plausible. [...] A geologically unprecedented reduction in the size of the biosphere occurred across the end-GOE transition."[261]
Rhyacian
[edit | edit source]Siderian
[edit | edit source]Neoarchean
[edit | edit source]Mesoarchean
[edit | edit source]Paleoarchean
[edit | edit source]Eoarchean
[edit | edit source]Hadean
[edit | edit source]~4600 – 4000 Ma.
Neohadean
[edit | edit source]The Neohadean is divided into the Acastan (4.1–4.0 Ga) and the Promethean periods (4.0–3.9 Ga).[262]
Traces of carbon minerals interpreted as "remains of biotic life" were found in 4.1-billion-year-old rocks in Western Australia.[263][264]
Zircons have been found that indicate that Australian Hadean rock holds minerals pointing to the existence of plate tectonics as early as 4 billion years ago (approximately 600 million years after Earth's formation).[265][266]
Roraima Group
[edit | edit source]Some folding occurred before these were overlain by the locally unconformable almost flat lying Roraima Group.[267]
Statherian Burro-Burro Group
[edit | edit source]Iwokrama Formation
[edit | edit source]Xenocryst (or relict) Hadean zircons enclosed in older rocks indicate that younger rocks have formed on older terranes and have incorporated some of the older material occur in the Guiana shield from the Iwokrama Formation of southern Guyana where zircon cores have been dated at 4.22 Ga.[268]
Muruwa Formation
[edit | edit source]Barama-Mazaruni Supergroup
[edit | edit source]Most of the geology of northern Guyana consists of Palaeoproterozoic Orosirian greenstone belts (Barama-Mazaruni Supergroup) intruded by granites. These are overlain unconformably by the Statherian Burro-Burro Group, which consist of the Muruwa Formation sandstones and Iwokrama Formation felsic volcanics. Both are intruded by granites associated with the Iwokrama Formation. Some folding occurred before these were overlain by the locally unconformable almost flat lying Roraima Group.[267]
Mesohadean
[edit | edit source]The Mesohadean is divided into the Canadian (4.3–4.2 Ga) and the Procrustean periods (4.2–4.1 Ga).[262]
Paleohadean
[edit | edit source]The Paleohadean Era consists of the Hephaestean (4.5–4.4 Ga) and the Jacobian periods (4.4–4.3 Ga). [262]
The oldest dated zircon crystals, enclosed in a metamorphosed sandstone conglomerate in the Jack Hills of the Narryer Gneiss Terrane of Western Australia, date to 4.404 ± 0.008 Ga.[269] This zircon is a slight outlier, with the oldest consistently-dated zircon falling closer to 4.35 Ga[269]
The Chaotian and Prenephelean Eons precede the Hadean.[262]
Zircons have been found that indicate that liquid water must have existed as long ago as 4.4 billion years ago, very soon after the formation of the Earth.[270] This requires the presence of an atmosphere. The cool early Earth theory covers a range from about 4.4 to about 4.1 billion years.
Eon | Era | Age (Ga) |
---|---|---|
Hadean | Jack Hillsian or Zirconian | 4.404 to 4.030 |
Chaotian | 4.568 to 4.404 |
Chaotian
[edit | edit source]4568 – 4404 Ma.
Eon | Era | Period | Age (Ga) |
---|---|---|---|
Chaotian | Neochaotian | Titanomachaen | > ~4.5 |
Hyperitian | |||
Eochaotian | Erebrean | ||
Nephelean |
Hypotheses
[edit | edit source]- To obtain stratigraphic columns in locations where exposures do not occur, corings may provide alternatives.
See also
[edit | edit source]- Genetics/Ammonoids
- Genetics/Human DNA
- Genetics/Human teeth
- Geochronology/Archaeology
- Geochronology/Cenozoic
- Geochronology/Dates
- Geochronology/Dendrochronology
- Geochronology/Dye 3
- Geochronology/Geomagnetic Polarity Time Scale
- Geochronology/Ice cores
- Geochronology/Ice cores/Black ice
- Geochronology/Ice cores/Brittle ice
- Geochronology/Ice cores/Clear ice
- Geochronology/Ice cores/Firns
- Geochronology/Ice cores/Sea ice
- Geochronology/Medieval Warm Period
- Geochronology/Mesozoic
- Geochronology/Middle Ages
- Geochronology/Neoglaciations
- Geochronology/Optically stimulated luminescence
- Geochronology/Paleontology
- Geochronology/Paleozoic
- Geochronology/Palynology
- Geochronology/Radiocarbon dating
- Geochronology/Stratigraphy
- Geochronology/Tephra layers
- Geochronology/Uranium-thorium dating
- Paleanthropology
- Geology
References
[edit | edit source]- ↑ SemperBlotto (27 August 2014). "stratigraphy". San Francisco, California: Wikimedia Foundation, Inc. Retrieved 2014-09-21.
{{cite web}}
:|author=
has generic name (help) - ↑ William Smith, Strata Identified by Organized Fossils, London: W. Arding, 1816
- ↑ Patrick Wyse Jackson, The Chronologers' Quest: The Search for the Age of the Earth, Cambridge University Press, 2006 isbn=1139457578 }}
- ↑ Levin, H.L. (2009). The Earth Through Time (9 ed.). John Wiley and Sons. p. 15. https://books.google.com/books?id=D0yl7Cqsu78C&pg=PA15&dq=principle+of+original+horizontality&hl=en&ei=Vq_yTIrgO4TDhAfw9fSxDQ&sa=X&oi=book_result&ct=result&resnum=5&ved=0CDsQ6AEwBA#v=onepage&q=principle%20of%20original%20horizontality&f=false. Retrieved 28 November 2010.
- ↑ In, Geology. "The Principle of Lateral Continuity". Retrieved 2018-04-10.
- ↑ See "Reading Rocks by Wesleyan University" retrieved May 8, 2011
- ↑ D. Armstrong, F. Mugglestone, R. Richards and F. Stratton, OCR AS and A2 Geology, Pearson Education Limited, 2008, p. 276 isbn: 978-0-435-69211-7
- ↑ Equinox (11 January 2013). "facies". San Francisco, California: Wikimedia Foundation, Inc. Retrieved 17 March 2019.
{{cite web}}
:|author=
has generic name (help) - ↑ Reading, H. G. (1996). Sedimentary Environments and Facies. Blackwell Scientific Publications. ISBN 0-632-03627-3.
- ↑ Parker, Sybil P. (1984). McGraw-Hill Concise Encyclopedia of Science and Technology. McGraw-Hill. p. 705. ISBN 0-07-045482-5.
- ↑ Stanley, Steven M. (1999). Earth System History. New York: W.H. Freeman and Company. pp. 134. ISBN 0-7167-2882-6.
- ↑ Nurgalieva, N. G.; Vinokurov, V. M.; Nurgaliev, D. K. (2007). "The Golovkinsky strata formation model, basic facies law and sequence stratigraphy concept: Historical sources and relations". Russian Journal of Earth Sciences 9. doi:10.2205/2007ES000222.
- ↑ 13.0 13.1 13.2 P. J. Barrett; B. P. Kohn; R. A. Askin; J. G. McPherson (1971). "Preliminary report on Beacon Supergroup studies between the Hatherton and Mackay glaciers, Antarctica". New Zealand Journal of Geology and Geophysics 14 (3): 605-14. doi:10.1080/00288306.1971.10421951. http://www.tandfonline.com/doi/pdf/10.1080/00288306.1971.10421951. Retrieved 2014-09-27.
- ↑ Evelyn Simak (16 November 2007). "Layers of glacial sediments resting on chalk base". Photograph. Retrieved 2016-01-24.
- ↑ 15.0 15.1 Eleyne Phillips (16 December 2004). "Glossary of Glacier Terminology". Reston, Virginia USA: United States Geological Survey. Retrieved 2014-11-09.
- ↑ "lias". San Francisco, California: Wikimedia Foundation, Inc. 29 December 2014. Retrieved 2015-02-19.
- ↑ Joe Fudge (31 December 2014). "Pictures: VIMS studies sediment core samples". Virginia: DailyPress. Retrieved 2015-01-12.
- ↑ Tamara Dietrich (1 January 2015). "VIMS geologists use sediment cores as a window to the past". DailyPress. Retrieved 2015-01-12.
- ↑ 19.0 19.1 Steve Kuehl (1 January 2015). "VIMS geologists use sediment cores as a window to the past". DailyPress. Retrieved 2015-01-12.
- ↑ Christopher Hein (1 January 2015). "VIMS geologists use sediment cores as a window to the past". DailyPress. Retrieved 2015-01-12.
- ↑ Mike Walker; Sigfus Johnsen; Sune Olander Rasmussen; Trevor Popp; Jørgen-Peder Steffensen; Phil Gibbard; Wim Hoek; John Lowe et al. (2009). "Formal definition and dating of the GSSP (Global Stratotype Section and Point) for the base of the Holocene using the Greenland NGRIP ice core, and selected auxiliary records". Journal of Quaternary Science 24 (1): 3-17. doi:10.1002/jqs.1227. http://www.stratigraphy.org/GSSP/Holocene.pdf. Retrieved 2015-01-18.
- ↑ International Commission on Stratigraphy. "ICS chart containing the Quaternary and Cambrian GSSPs and new stages (v 2018/07) is now released!". Retrieved 15 July 2018.
{{cite web}}
:|archive-date=
requires|archive-url=
(help) - ↑ "'Meghalayan Age' makes the state a part of geologic history". Hindustan Times. 18 July 2018. Retrieved 26 July 2018.
- ↑ "Formal subdivision of the Holocene Series/Epoch" (PDF).
- ↑ 25.0 25.1 International Commission on Stratigraphy. "GSSP Table – All Periods". GSSPs. Retrieved 15 July 2018.
- ↑ 26.0 26.1 Maria Bianca Cita; Philip L. Gibbard; Martin J. Head; the ICS Subcommission on Quaternary Stratigraphy (September 2012). "Formal ratification of the GSSP for the base of the Calabrian Stage (second stage of the Pleistocene Series, Quaternary System)". Episodes 35 (3): 388-97. http://www.stratigraphy.org/GSSP/Calabrian2.pdf. Retrieved 2015-01-18.
- ↑ Philip L. Gibbard; Martin J. Head (September 2010). "The newly-ratified definition of the Quaternary System/Period and redefinition of the Pleistocene Series/Epoch, and comparison of proposals advanced prior to formal ratification". Episodes 33 (3): 152-8. http://www.stratigraphy.org/GSSP/Quaternary&Pleistocene.pdf. Retrieved 2015-01-20.
- ↑ D. Rio; R. Sprovieri; D. Castradori; E. Di Stefano (June 1998). "The Gelasian Stage (Upper Pliocene): A new unit of the global standard chronostratigraphic scale". Episodes 21 (2): 82-7. http://www.stratigraphy.org/GSSP/Gelasian.pdf. Retrieved 2015-01-20.
- ↑ D. Castradori; D. Rio; F. J. Hilgen; L. J. Lourens (June 1998). "The Global Standard Stratotype-section and Point (GSSP) of the Piacenzian Stage (Middle Pliocene)". Episodes 21 (2): 88-93. http://www.stratigraphy.org/GSSP/Piacenzian.pdf. Retrieved 2015-01-23.
- ↑ 30.0 30.1 30.2 30.3 30.4 30.5 30.6 Love, D.W. (1989). "Bidahochi Formation; an interpretive summary". New Mexico Geological Society Field Conference Guidebook 40: 273–280. https://nmgs.nmt.edu/publications/guidebooks/downloads/40/40_p0273_p0280.pdf. Retrieved 8 October 2020.
- ↑ "Geologic Formations". Petrified Forest National Park, Arizona. National Park Service. 13 September 2020. Retrieved 24 June 2021.
- ↑ 32.0 32.1 Repenning, Charles A.; Irwin, James H. (1954). "Bidahochi Formation of Arizona and New Mexico: GEOLOGICAL NOTES". AAPG Bulletin 38: 1821–1826. doi:10.1306/5CEAE040-16BB-11D7-8645000102C1865D.
- ↑ Hackman, R.J.; Olson, A.B. (1977). "Geology, structure, and uranium deposits of the Gallup 1 degree x 2 degrees quadrangle, New Mexico and Arizona". U.S. Geological Survey Miscellaneous Investigations Series Map I-981. http://ngmdb.usgs.gov/Prodesc/proddesc_9849.htm. Retrieved 23 June 2021.
- ↑ 34.0 34.1 34.2 34.3 34.4 Dickinson, W. R. (2013). "Rejection of the lake spillover model for initial incision of the Grand Canyon, and discussion of alternatives". Geosphere 9 (1): 1–20. doi:10.1130/GES00839.1.
- ↑ 35.0 35.1 Dallegge, Todd A.; Ort, Michael H.; McIntosh, William C. (2003). "Mio-Pliocene chronostratigraphy, basin morphology and paleodrainage relations derived from the Bidahochi Formation, Hopi and Navajo Nations, northeastern Arizona". The Mountain Geologist 40 (3): 55–82. https://archives.datapages.com/data/rmag/mg/2003/dallegge.htm. Retrieved 24 June 2021.
- ↑ 36.0 36.1 Sears, J.W. (2013). "Late Oligocene–early Miocene Grand Canyon: A Canadian connection". GSA Today 23 (11): 4–10. doi:10.1130/GSATG178A.1.
- ↑ Blakey, Ronald C. (2008). Ancient landscapes of the Colorado plateau (1st ed.). Grand Canyon, AZ: Grand Canyon Association. ISBN 978-1934656037.
- ↑ Schmidt, Karl-Heinz (August 1991). "Tertiary palaeoclimatic history of the southeastern Colorado Plateau". Palaeogeography, Palaeoclimatology, Palaeoecology 86 (3–4): 283–296. doi:10.1016/0031-0182(91)90086-7.
- ↑ "Scala dei Turchi (AG)". www.geositidisicilia.it. Retrieved 2021-08-12.
- ↑ John A. Van Couvering; Davide Castradori; Maria Bianca Cita; Frederik J. Hilgen; Domenico Rio (September 2000). "The base of the Zanclean Stage and of the Pliocene Series". Episodes 23 (3): 179-87. http://www.stratigraphy.org/GSSP/Zanclean.pdf. Retrieved 2015-01-23.
- ↑ 41.0 41.1 F.J. Hilgen; S. Iaccarino; W. Krijgsman; G. Villa; C.G. Langereis (2000). "The Global Boundary Stratotype Section and Point (GSSP) of the Messinian Stage (uppermost Miocene)". Episodes 23 (3): 172-178. http://www.stratigraphy.org/GSSP/Messinian.pdf. Retrieved 2017-08-20.
- ↑ 42.0 42.1 42.2 42.3 42.4 Rik Houthuys & Johan Matthijs (2020). "Reinterpretation of the Neogene sediments of the Bree Uplift, NE Belgium". Geologica Belgica 23 (3-4): 345-363. https://popups.uliege.be/1374-8505/index.php?id=6812. Retrieved 19 October 2021.
- ↑ 43.00 43.01 43.02 43.03 43.04 43.05 43.06 43.07 43.08 43.09 43.10 43.11 Stephen Louwye, Jef Deckers, Jasper Verhaegen, Rieko Adriaens & Noël Vandenberghe (2020). "A review of the lower and middle Miocene of northern Belgium". Geologica Belgica 23 (3-4): 137-156. https://biblio.ugent.be/publication/8694505/file/8694508. Retrieved 19 October 2021.
- ↑ Zachos, J.; Pagani, M.; Sloan, L.; Thomas, E.; Billups, K. (2001). "Trends, rhythms, and aberrations in global climate 65 Ma to present". Science 292 (5517): 686–693. doi:10.1126/science.1059412. PMID 11326091.
- ↑ Prothero, D. (May 2005). Tertiary to Present | Oligocene. Encyclopedia of Geology. pp. 472–478. doi:10.1016/B0-12-369396-9/00056-3. ISBN 978-0-12-369396-9.
- ↑ 46.0 46.1 Torsvik, Trond H.; Cocks, L. Robin M. (2017). Earth history and palaeogeography. Cambridge, United Kingdom: Cambridge University Press. ISBN 9781107105324.
- ↑ Wilson, G.S.; Pekar, S.F.; Naish, T.R.; Passchier, S.; DeConto, R. (2008). "Chapter 9 The Oligocene–Miocene Boundary – Antarctic Climate Response to Orbital Forcing". Developments in Earth and Environmental Sciences 8: 369–400. doi:10.1016/S1571-9197(08)00009-8.
- ↑ O’Brien, Charlotte L.; Huber, Matthew; Thomas, Ellen; Pagani, Mark; Super, James R.; Elder, Leanne E.; Hull, Pincelli M. (13 October 2020). "The enigma of Oligocene climate and global surface temperature evolution". Proceedings of the National Academy of Sciences. 117 (41): 25302–25309. doi:10.1073/pnas.2003914117.
- ↑ Prothero, D. (May 2005). Tertiary to Present | Oligocene. Encyclopedia of Geology. pp. 472–478. doi:10.1016/B0-12-369396-9/00056-3. ISBN 978-0-12-369396-9.
- ↑ O’Brien, Charlotte L.; Huber, Matthew; Thomas, Ellen; Pagani, Mark; Super, James R.; Elder, Leanne E.; Hull, Pincelli M. (13 October 2020). "The enigma of Oligocene climate and global surface temperature evolution". Proceedings of the National Academy of Sciences. 117 (41): 25302–25309. doi:10.1073/pnas.2003914117.
- ↑ Lear, C. H. (14 January 2000). "Cenozoic Deep-Sea Temperatures and Global Ice Volumes from Mg/Ca in Benthic Foraminiferal Calcite". Science 287 (5451): 269–272. doi:10.1126/science.287.5451.269.
- ↑ Pälike, Heiko; Norris, Richard D.; Herrle, Jens O.; Wilson, Paul A.; Coxall, Helen K.; Lear, Caroline H.; Shackleton, Nicholas J.; Tripati, Aradhna K. et al. (22 December 2006). "The Heartbeat of the Oligocene Climate System". Science 314 (5807): 1894–1898. doi:10.1126/science.1133822.
- ↑ Coxall, H.K.; Pearson, P.N. (2007). "The Eocene–Oligocene Transition". In Williams, M.; Haywood, A.M.; Gregory, F.J.; Schmidt, D.N. (eds.). Deep-Time Perspectives on Climate Change: Marrying the Signal from Computer Models and Biological Proxies. The Micropalaeontological Society, Special Publications. London: The Geological Society. pp. 351–387.
- ↑ Liu, Z.; Pagani, M.; Zinniker, D.; DeConto, R.; Huber, M.; Brinkhuis, H.; Shah, S. R.; Leckie, R. M. et al. (27 February 2009). "Global Cooling During the Eocene-Oligocene Climate Transition". Science 323 (5918): 1187–1190. doi:10.1126/science.1166368.
- ↑ O’Brien, Charlotte L.; Huber, Matthew; Thomas, Ellen; Pagani, Mark; Super, James R.; Elder, Leanne E.; Hull, Pincelli M. (13 October 2020). "The enigma of Oligocene climate and global surface temperature evolution". Proceedings of the National Academy of Sciences. 117 (41): 25302–25309. doi:10.1073/pnas.2003914117.
- ↑ Scher, H. D.; Martin, E. E. (21 April 2006). "Timing and Climatic Consequences of the Opening of Drake Passage". Science 312 (5772): 428–430. doi:10.1126/science.1120044.
- ↑ Prothero, D. (May 2005). Tertiary to Present | Oligocene. Encyclopedia of Geology. pp. 472–478. doi:10.1016/B0-12-369396-9/00056-3. ISBN 978-0-12-369396-9.
- ↑ Torsvik, Trond H.; Cocks, L. Robin M. (2017). Earth history and palaeogeography. Cambridge, United Kingdom: Cambridge University Press. pp. 241–245. ISBN 9781107105324.
- ↑ Lagabrielle, Yves; Goddéris, Yves; Donnadieu, Yannick; Malavieille, Jacques; Suarez, Manuel (30 March 2009). "The tectonic history of Drake Passage and its possible impacts on global climate". Earth and Planetary Science Letters 279 (3-4): 197–211. doi:10.1016/j.epsl.2008.12.037.
- ↑ 60.0 60.1 60.2 Prothero, D. (May 2005). Tertiary to Present | Oligocene. 472–478. doi:10.1016/B0-12-369396-9/00056-3. ISBN 978-0-12-369396-9.
- ↑ 61.0 61.1 61.2 Mackensen, Andreas (Dec 2004). "Changing Southern Ocean palaeocirculation and effects on global climate". Antarctic Science 16 (4): 369–389. doi:10.1017/S0954102004002202.
- ↑ 62.0 62.1 62.2 Via, Rachael; Thomas, D. (June 2006). "Evolution of Antarctic thermohaline circulation: Early Oligocene onset of deep-water production in the North Atlantic". Geology 34 (6): 441–444. doi:10.1130/G22545.1.
- ↑ 63.0 63.1 Katz, M; Cramer, B.; Toggweiler, J.; Esmay, G.; Liu, C.; Miller, K.; Rosenthal, Y.; Wade, B. et al. (May 2011). "Impact of Antarctic Circumpolar Current development on late Paleogene ocean structure". Science 332 (6033): 1076–1079. doi:10.1126/science.1202122. PMID 21617074.
- ↑ 64.0 64.1 64.2 64.3 64.4 Lyle, Mitchell; Barron, J.; Bralower, T.; Huber, M.; Olivarez Lyle, A.; Ravelo, A. C.; Rea, D. K.; Wilson, P. A. (April 2008). "Pacific Ocean and Cenozoic evolution of climate". Reviews of Geophysics 46 (2): RG2002. doi:10.1029/2005RG000190. https://deepblue.lib.umich.edu/bitstream/2027.42/95039/1/rog1637.pdf.
- ↑ Torsvik, Trond H.; Cocks, L. Robin M. (2017). Earth history and palaeogeography. Cambridge, United Kingdom: Cambridge University Press. pp. 241–245. ISBN 9781107105324.
- ↑ Prothero, D. (May 2005). Tertiary to Present | Oligocene. Encyclopedia of Geology. pp. 472–478. doi:10.1016/B0-12-369396-9/00056-3. ISBN 978-0-12-369396-9.
- ↑ Torsvik, Trond H.; Cocks, L. Robin M. (2017). Earth history and palaeogeography. Cambridge, United Kingdom: Cambridge University Press. pp. 241–245. ISBN 9781107105324.
- ↑ Prothero, D. (May 2005). Tertiary to Present | Oligocene. Encyclopedia of Geology. pp. 472–478. doi:10.1016/B0-12-369396-9/00056-3. ISBN 978-0-12-369396-9.
- ↑ Torsvik, Trond H.; Cocks, L. Robin M. (2017). Earth history and palaeogeography. Cambridge, United Kingdom: Cambridge University Press. pp. 241–245. ISBN 9781107105324.
- ↑ Prothero, D. (May 2005). Tertiary to Present | Oligocene. Encyclopedia of Geology. pp. 472–478. doi:10.1016/B0-12-369396-9/00056-3. ISBN 978-0-12-369396-9.
- ↑ van Hinsbergen, D. J. J.; Lippert, P. C.; Dupont-Nivet, G.; McQuarrie, N.; Doubrovine, P. V.; Spakman, W.; Torsvik, T. H. (15 May 2012). "Greater India Basin hypothesis and a two-stage Cenozoic collision between India and Asia". Proceedings of the National Academy of Sciences 109 (20): 7659–7664. doi:10.1073/pnas.1117262109.
- ↑ Torsvik, Trond H.; Cocks, L. Robin M. (2017). Earth history and palaeogeography. Cambridge, United Kingdom: Cambridge University Press. pp. 241–245. ISBN 9781107105324.
- ↑ DeCelles, Peter G.; Quade, Jay; Kapp, Paul; Fan, Majie; Dettman, David L.; Ding, Lin (January 2007). "High and dry in central Tibet during the Late Oligocene". Earth and Planetary Science Letters 253 (3-4): 389–401. doi:10.1016/j.epsl.2006.11.001.
- ↑ Prothero, D. (May 2005). Tertiary to Present | Oligocene. Encyclopedia of Geology. pp. 472–478. doi:10.1016/B0-12-369396-9/00056-3. ISBN 978-0-12-369396-9.
- ↑ Orme, Antony R. (2007). "The Tectonic Framework of South America". Physical Geography of South America. Oxford University Press. pp. 12–17. https://archive.org/details/physicalgeograph00vebl.
- ↑ Prothero, D. (May 2005). Tertiary to Present | Oligocene. Encyclopedia of Geology. pp. 472–478. doi:10.1016/B0-12-369396-9/00056-3. ISBN 978-0-12-369396-9.
- ↑ Prothero, D. (May 2005). Tertiary to Present | Oligocene. Encyclopedia of Geology. pp. 472–478. doi:10.1016/B0-12-369396-9/00056-3. ISBN 978-0-12-369396-9.
- ↑ Retallack, G.J. (1983). "Late Eocene and Oligocene paleosols from Badlands National Park, South Dakota". Geological Society of America Special Paper 193. ISBN 9780813721934.
- ↑ Zanazzi, Alessandro; Kohn, Matthew J.; MacFadden, Bruce J.; Terry, Dennis O. (February 2007). "Large temperature drop across the Eocene–Oligocene transition in central North America". Nature 445 (7128): 639–642. doi:10.1038/nature05551.
- ↑ Eldrett, James S.; Harding, Ian C.; Wilson, Paul A.; Butler, Emily; Roberts, Andrew P. (March 2007). "Continental ice in Greenland during the Eocene and Oligocene". Nature 446 (7132): 176–179. doi:10.1038/nature05591.
- ↑ Coxall, H.K.; Pearson, P.N. (2007). "The Eocene–Oligocene Transition". In Williams, M.; Haywood, A.M.; Gregory, F.J.; Schmidt, D.N. (eds.). Deep-Time Perspectives on Climate Change: Marrying the Signal from Computer Models and Biological Proxies. The Micropalaeontological Society, Special Publications. London: The Geological Society. pp. 351–387.
- ↑ Berggren, William A.; Prothero, Donald R. (1992). "Eocene-Oligocene climatic and biotic evolution: an overview". Eocene-Oligocene Climatic and Biotic Evolution. Princeton University Press. p. 1. doi:10.1515/9781400862924.1.
- ↑ O’Brien, Charlotte L.; Huber, Matthew; Thomas, Ellen; Pagani, Mark; Super, James R.; Elder, Leanne E.; Hull, Pincelli M. (13 October 2020). "The enigma of Oligocene climate and global surface temperature evolution". Proceedings of the National Academy of Sciences 117 (41): 25302–25309. doi:10.1073/pnas.2003914117.
- ↑ Berggren, William A.; Prothero, Donald R. (1992). "Eocene-Oligocene climatic and biotic evolution: an overview". Eocene-Oligocene Climatic and Biotic Evolution. Princeton University Press. p. 1. doi:10.1515/9781400862924.1.
- ↑ O’Brien, Charlotte L.; Huber, Matthew; Thomas, Ellen; Pagani, Mark; Super, James R.; Elder, Leanne E.; Hull, Pincelli M. (13 October 2020). "The enigma of Oligocene climate and global surface temperature evolution". Proceedings of the National Academy of Sciences. 117 (41): 25302–25309. doi:10.1073/pnas.2003914117.
- ↑ Prothero, D. (May 2005). Tertiary to Present | Oligocene. Encyclopedia of Geology. pp. 472–478. doi:10.1016/B0-12-369396-9/00056-3. ISBN 978-0-12-369396-9.
- ↑ O’Brien, Charlotte L.; Huber, Matthew; Thomas, Ellen; Pagani, Mark; Super, James R.; Elder, Leanne E.; Hull, Pincelli M. (13 October 2020). "The enigma of Oligocene climate and global surface temperature evolution". Proceedings of the National Academy of Sciences. 117 (41): 25302–25309. doi:10.1073/pnas.2003914117.
- ↑ Prothero, D. (May 2005). Tertiary to Present | Oligocene. Encyclopedia of Geology. pp. 472–478. doi:10.1016/B0-12-369396-9/00056-3. ISBN 978-0-12-369396-9.
- ↑ Berggren, William A.; Prothero, Donald R. (1992). "Eocene-Oligocene climatic and biotic evolution: an overview". Eocene-Oligocene Climatic and Biotic Evolution. Princeton University Press. p. 1. doi:10.1515/9781400862924.1.
- ↑ Prothero, D. (May 2005). Tertiary to Present | Oligocene. Encyclopedia of Geology. pp. 472–478. doi:10.1016/B0-12-369396-9/00056-3. ISBN 978-0-12-369396-9.
- ↑ Lyle, Mitchell; Gibbs, Samantha; Moore, Theodore C.; Rea, David K. (2007). "Late Oligocene initiation of the Antarctic Circumpolar Current: Evidence from the South Pacific". Geology 35 (8): 691. doi:10.1130/G23806A.1.
- ↑ O’Brien, Charlotte L.; Huber, Matthew; Thomas, Ellen; Pagani, Mark; Super, James R.; Elder, Leanne E.; Hull, Pincelli M. (13 October 2020). "The enigma of Oligocene climate and global surface temperature evolution". Proceedings of the National Academy of Sciences. 117 (41): 25302–25309. doi:10.1073/pnas.2003914117.
- ↑ Francis, J.E.; Marenssi, S.; Levy, R.; Hambrey, M.; Thorn, V.C.; Mohr, B.; Brinkhuis, H.; Warnaar, J. et al. (2008). "Chapter 8 From Greenhouse to Icehouse – The Eocene/Oligocene in Antarctica". Developments in Earth and Environmental Sciences 8: 309–368. doi:10.1016/S1571-9197(08)00008-6.
- ↑ Pearson, Paul N.; Foster, Gavin L.; Wade, Bridget S. (October 2009). "Atmospheric carbon dioxide through the Eocene–Oligocene climate transition". Nature 461 (7267): 1110–1113. doi:10.1038/nature08447.
- ↑ Francis, J.E.; Marenssi, S.; Levy, R.; Hambrey, M.; Thorn, V.C.; Mohr, B.; Brinkhuis, H.; Warnaar, J.; Zachos, J.; Bohaty, S.; DeConto, R. (2008). "Chapter 8 From Greenhouse to Icehouse – The Eocene/Oligocene in Antarctica". Developments in Earth and Environmental Sciences. 8: 309–368. doi:10.1016/S1571-9197(08)00008-6.
- ↑ 96.0 96.1 von der Heydt, Anna; Dijkstra, Henk A. (May 2008). "The effect of gateways on ocean circulation patterns in the Cenozoic". Global and Planetary Change. 1-2 62 (1–2): 132–146. doi:10.1016/j.gloplacha.2007.11.006.
- ↑ Allen, Mark; Armstrong, Howard (July 2008). "Arabia-Eurasia cooling and the forcing of mid-Cenozoic global cooling". Palaeogeology, Palaeoclimatology, Palaeoecology. 1-2 265 (1–2): 52–58. doi:10.1016/j.palaeo.2008.04.021. http://dro.dur.ac.uk/14557/1/14557.pdf.
- ↑ "Haughton". http://www.passc.net/EarthImpactDatabase/New%20website_05-2018/Index.html. Planetary and Space Science Centre University of New Brunswick Fredericton. Retrieved 2017-10-09.
- ↑ Sherlock, S.C.; Kelley, S.P. (2005). "Re-evaluating the age of the Haughton impact event". Meteoritics & Planetary Science 40 (12): 1777–1787. doi:10.1111/j.1945-5100.2005.tb00146.x.
- ↑ Breining, Greg (2007). "Most-Super Volcanoes". Super Volcano: The Ticking Time Bomb Beneath Yellowstone National Park. St. Paul, MN: Voyageur Press. pp. 256 pg. ISBN 978-0-7603-2925-2. https://archive.org/details/supervolcanotick0000brei/page/256.
- ↑ Jens Lorenz Franzen (2005). "The implications of the numerical dating of the Messel fossil deposit (Eocene, Germany) for mammalian biochronology." Annales de Paléontologie. 91 (4): 329–335. doi:10.1016/j.annpal.2005.04.002
- ↑ 102.0 102.1 H. Buness, M. Felder, G. Gabriel, F.-J. Harms: Explosives Tropenparadies. Geologie und Geophysik im Zeitraffer. In: Fossillagerstätte Grube Messel. Momentaufnahmen aus dem Eozän. Vernissage, Reihe: Unesco-Welterbe, Nr. 21/05, 13. Jhg. (2005), S. 6–11
- ↑ Thomas Nix: Untersuchung der ingenieurgeologischen Verhältnisse der Grube Messel (Darmstadt) im Hinblick auf die Langzeitstabilität der Grubenböschungen. Dissertation zum Erlangen des akademischen Grades eines Doktor-Ingenieurs (Dr.-Ing.), Fachbereich Material- und Geowissenschaften der Technischen Universität Darmstadt. Darmstadt 2003, S. 31 ff., online
- ↑ Olaf K. Lenz, Volker Wilde, Dieter F. Mertz und Walter Riegel: New palynology-based astronomical and revised 40Ar/39Ar ages for the Eocene maar lake of Messel (Germany). In: International Journal of Earth Sciences. 21. Dezember 2014, doi:10.1007/s00531-014-1126-2
- ↑ J.E. Fassett; S.G. Lucas; R.A. Zielinski; J.R. Budahn (May 2001). SG Lucas. ed. Compelling new evidence for Paleocene dinosaurs in the Ojo Alamo Sandstone, San Juan Basin, New Mexico and Colorado, USA, In: Catastrophic events and mass extinctions, Lunar and Planetary Contribution. 1053. pp. 45-6. Bibcode: 2001caev.conf.3139F. http://www.lpi.usra.edu/meetings/impact2000/pdf/3139.pdf. Retrieved 2014-08-29.
- ↑ 106.0 106.1 106.2 106.3 Fouch, T. D., Lawton, T. F., Nichols, D. J., Cashion, W. B., Cobban, W. A. (1983). Patterns and timing of synorogenic sedimentation in Upper Cretaceous rocks of central and northeast Utah. In Reynolds, M. W., and Dolly, E. D., eds., Mesozoic paleogeography of west central United States. Society of Economic Paleontologists and Mineralogists. Pp. 305-336.
- ↑ 107.0 107.1 107.2 107.3 107.4 107.5 107.6 Lawton, T. F. (1986). Fluvial systems of the Upper Cretaceous Mesaverde Group and Paleocene North Horn Formation, central Utah: A record of transition from thin-skinned to thick-skinned deformation in the foreland region. Paleotectonics and Sedimentatio in the Rocky Mountain Region, United States. AAPG Special Volumes, M 41. Pp. 423-442.
- ↑ 108.0 108.1 108.2 108.3 Lawton, T. F., Talling, P. J., Hobbs, R. S., Trexler, J. H. Jr, Weiss, M. P., Burbank, D. W. (1993). Structure and stratigraphy of Upper Cretaceous and Paleocene strata (North Horn Formation), eastern San Pitch Mountains, Utah – sedimentation at the front of the Sevier orogenic belt. United States Geological Survey, Bulletin 1787-II, Pp. 1-33.
- ↑ 109.0 109.1 109.2 109.3 Difley, R., Ekdale, A. A. (2002). Faunal implications of an environmental change before the Cretaceous-Tertiary (K-T) transition in central Utah. Cretaceous Research, 23:315-331.
- ↑ Myung-Suk, Y., Cross, A.T. (1997). Palynostratigraphy of Upper Cretaceous-Lower Tertiary strata, Price Canyon, Utah. Review of Palaeobotany and Palynology 97, p.53-66
- ↑ 111.00 111.01 111.02 111.03 111.04 111.05 111.06 111.07 111.08 111.09 111.10 111.11 111.12 111.13 Gilmore, Charles W. (1946). "Reptilian fauna of the North Horn Formation of central Utah". US Government Printing Office 210: 48. https://www.google.com/books/edition/Reptilian_Fauna_of_the_North_Horn_Format/yRNoyNAv9lIC?hl=en&gbpv=1&pg=PA42&printsec=frontcover. Retrieved 24 October 2020.
- ↑ 112.0 112.1 Cifelli, R.L., Czaplewski, N.J., Rose, K.D. (1995). Knowledge of Paleocene Mammals from the North Horn Formation, Central Utah. The Great Basin Naturalist. Vol. 55:4. Pp. 304-314
- ↑ 113.0 113.1 Cifelli, R.L., Nydam, R.L., Eaton, J.G., Gardner, J.D., Kirkland, J.I. (1999). Vertebrate Faunas of the North Horn Formation (Upper Cretaceous-Lower Paleocene), Emery and Sanpete Counties, Utah. In Gillette, D.D. (ed.) Vertebrate Paleontology in Utah. Utah Geological Survey Miscellaneous Publication 99-1: 377-388
- ↑ Difley, Rose L.; Ekdale, Allan A. (August 2002). "Footprints of Utah's Last Dinosaurs: Track Beds in the Upper Cretaceous (Maastrichtian) North Horn Formation of the Wasatch Plateau, Central Utah". PALAIOS 17 (4): 327–346. doi:10.1669/0883-1351(2002)017<0327:FOUSLD>2.0.CO;2. https://www.jstor.org/stable/3515759. Retrieved 18 October 2020.
- ↑ Spieker, E. M. (1946). Late Mesozoic and early Cenozoic history of central Utah. United States Geological Survey. Professional Paper 205-D, 117-159
- ↑ Black, Riley (2005). When Tyrannosaurus Chomped Sauropods. 25. Smithsonian Institution. p. 469. doi:10.1671/0272-4634(2005)025[0469:TRFTUC]2.0.CO;2. ISSN 0272-4634. https://www.smithsonianmag.com/science-nature/when-tyrannosaurus-chomped-sauropods-67170161/. Retrieved 13 April 2012.
- ↑ Black, Riley. "Tyrannosaurus vs. Alamosaurus". Smithsonian Magazine. Smithsonian Institution. Retrieved 23 March 2009.
- ↑ 118.0 118.1 118.2 Sampson, Scott D.; Loewon, Mark A. (27 June 2005). "Tyrannosaurus rex from the Upper Cretaceous (Maastrichtian) North Horn Formation of Utah: Biogeographic and Paleoecologic Implications". Journal of Vertebrate Paleontology 25 (2): 469–472. doi:10.1671/0272-4634(2005)025[0469:TRFTUC]2.0.CO;2. https://www.jstor.org/stable/4524461. Retrieved 18 October 2020.
- ↑ Cifelli, Richard L.; de Muizon, Christian (May 1998). "Marsupial mammal from the Upper Cretaceous North Horn Formation, Central Utah". Journal of Paleontology (Cambridge University Press) 72 (3): 532–637. doi:10.1017/S0022336000024306. https://www.cambridge.org/core/journals/journal-of-paleontology/article/marsupial-mammal-from-the-upper-cretaceous-north-horn-formation-central-utah/1BE8E382E4B350E46ADD3AB56AE43384. Retrieved 19 November 2020.
- ↑ 120.0 120.1 120.2 120.3 Lockley, M.; Harris, J.D.; and Mitchell, L. 2008. "A global overview of pterosaur ichnology: tracksite distribution in space and time." Zitteliana. B28. p. 187-198. ISSN 1612-4138.
- ↑ 121.0 121.1 121.2 121.3 121.4 121.5 Weishampel, David B; et al. (2004). "Dinosaur distribution (Late Cretaceous, North America)." In: Weishampel, David B.; Dodson, Peter; and Osmólska, Halszka (eds.): The Dinosauria, 2nd, Berkeley: University of California Press. Pp. 574-588. ISBN 0-520-24209-2.
- ↑ https://geoparkea.eus/en/geology
- ↑ https://geoparkea.eus/site_media/pdf/AAFF_MAPA_GEOPARKEA_ING_BAJA_gtZCQtH.pdf
- ↑ 124.0 124.1 124.2 124.3 124.4 124.5 124.6 124.7 Sietske Batenburg, Vicente Gilabert, Ignacio Arenillas and José Antonio Arz (20 September 2021). "Extreme Volcanism Did Not Cause The Massive Extinction Of Species In The Late Cretaceous". Science Blog. Retrieved 20 September 2021.
{{cite web}}
: CS1 maint: multiple names: authors list (link) - ↑ Vicente Gilabert, Ignacio Arenillas and José Antonio Arz (20 September 2021). "Extreme Volcanism Did Not Cause The Massive Extinction Of Species In The Late Cretaceous". Science Blog. Retrieved 20 September 2021.
- ↑ Eustoquio Molina; Laia Alegret; Ignacio Arenillas; José A. Arz; Njoud Gallala; Jan Hardenbol; Katharina von Salis; Etienne Steurbaut et al. (December 2006). "The Global Boundary Stratotype Section and Point for the base of the Danian Stage (Paleocene, Paleogene, "Tertiary", Cenozoic) at El Kef, Tunisia - Original definition and revision". Episodes 29 (4): 263-73. http://www.stratigraphy.org/GSSP/Danian.pdf. Retrieved 2015-01-19.
- ↑ Pierce, W.G., 1997, Geologic map of the Cody 1 degree x 2 degrees quadrangle, northwestern Wyoming: U.S. Geological Survey, Miscellaneous Geologic Investigations Map I-2500, scale 1:250000.
- ↑ "Geologic Unit: Cody". National Geologic Database. Geolex — Unit Summary. United States Geological Survey. Retrieved 2018-06-29.
- ↑ Pierce, W.G., 1997, Geologic map of the Cody 1 degree x 2 degrees quadrangle, northwestern Wyoming: U.S. Geological Survey, Miscellaneous Geologic Investigations Map I-2500, scale 1:250000.
- ↑ 130.0 130.1 Weishampel, David B; et al. (2004). "Dinosaur distribution (Late Cretaceous, North America)." In: Weishampel, David B.; Dodson, Peter; and Osmólska, Halszka (eds.): The Dinosauria, 2nd, Berkeley: University of California Press. Pp. 574-588. ISBN 0-520-24209-2.
- ↑ Panasci, Giulio; Varricchio, David J.; Martin, Anthony (October 10, 2021). "TRACKS OF ORNITHOPODS PUTTING THEIR BEST FEET FORWARD IN THE FRONTIER FORMATION (CONIACIAN), MONTANA". Geological Society of America Abstracts with Programs. 53 (6). https://gsa.confex.com/gsa/2021AM/meetingapp.cgi/Paper/367407.
- ↑ "Shinarump Member of Chinle Formation". Colorado River Basin Stratigraphy. USGS. 2006-05-06. Retrieved 2011-01-10.
- ↑ Dubiel, R.F. (1989). "Depositional and climatic setting of the Upper Triassic Chinle Formation, Colorado Plateau". Dawn of the Age of Dinosaurs in the American Southwest. New Mexico Museum of Natural History. pp. 171–187. https://books.google.com/books?id=Ar1hCgAAQBAJ&pg=PP1. Retrieved 28 October 2021.
- ↑ Lucas, Spencer G.; Hayden, S.N. (1989). "Triassic stratigraphy of west-central New Mexico". New Mexico Geological Society Field Conference Guidebook 40: 191–211. https://nmgs.nmt.edu/publications/guidebooks/downloads/40/40_p0191_p0211.pdf. Retrieved 30 October 2020.
- ↑ Milner, A.R. (2006). "Plant fossils from the Owl Rock or Church Rock Members, Chinle Formation, San Juan County, Utah". New Mexico Museum of Natural History and Science Bulletin 37: 410-413. https://www.google.com/books/edition/The_Triassic_Jurassic_Terrestrial_Transi/ulvmCQAAQBAJ?hl=en&gbpv=1&pg=PA410&printsec=frontcover. Retrieved 19 February 2022.
- ↑ 136.0 136.1 136.2 136.3 GEOLEX database entry for Chinle, USGS (viewed 19 March 2006)
- ↑ Litwin, R.J., Traverse, A., and Ash, S.R., 1991. Preliminary palynological zonation of the Chinle Formation, southwestern U.S.A., and its correlation to the Newark Supergroup (eastern U.S.A.). Review of Paleobotany and Palynology, v. 77, pp. 269–287.
- ↑ 138.0 138.1 138.2 138.3 138.4 138.5 Lucas, S.G.; Hunt, A.P.; Huber, P. (1990). "Triassic stratigraphy in the Sangre de Cristo Mountains, New Mexico". New Mexico Geological Society Field Conference Guidebook 41: 305–318. https://nmgs.nmt.edu/publications/guidebooks/downloads/41/41_p0305_p0318.pdf. Retrieved 12 November 2021.
- ↑ 139.0 139.1 139.2 139.3 139.4 Lucas, Spencer G.; Zeigler, Kate E.; Heckert, Andrew B.; Hunt, Adrian P. (2005). "Review of Upper Triassic stratigraphy and biostratigraphy in the Chama Basin, northern New Mexico". New Mexico Geological Society Field Conference Series 56: 170–181. https://nmgs.nmt.edu/publications/guidebooks/downloads/56/56_p0170_p0181.pdf. Retrieved 29 April 2020.
- ↑ 140.0 140.1 140.2 Lucas, S.G. (1991). "Triassic stratigraphy, paleontology and correlation, south-central New Mexico". New Mexico Geological Society Field Conference Guidebook 42: 243–253. https://nmgs.nmt.edu/publications/guidebooks/downloads/42/42_p0243_p0259.pdf. Retrieved 6 August 2020.
- ↑ Lucas, Spencer G. (1993). "The Chinle Group: revised stratigraphy and biochronology of Upper Triassic Nonmarine strata in the western United States". Museum of Northern Arizona Bulletin 59: 27–50.
- ↑ Finch, W.I.; Lupe, Robert; Ash, S.R. (1988). "Principal reference section for the Santa Rosa formation of Middle and Late Triassic age, Guadalupe County, New Mexico". U.S. Geological Survey Bulletin 1804. doi:10.3133/b1804.
- ↑ Wood, G.H.; Northrop, S.A. (1946). "Geology of the Nacimiento Mountains, San Pedro Mountain, and adjacent plateaus in parts of Sandoval and Rio Arriba Counties, New Mexico". USGS Oil and Gas Investigations OM-57. doi:10.3133/om57. https://ngmdb.usgs.gov/Prodesc/proddesc_5417.htm. Retrieved 29 April 2020.
- ↑ 144.0 144.1 Lucas, Spencer G.; Hayden, S.N. (1989). "Triassic stratigraphy of west-central New Mexico". New Mexico Geological Society Field Conference Guidebook 40: 191–211. https://nmgs.nmt.edu/publications/guidebooks/downloads/40/40_p0191_p0211.pdf. Retrieved 30 October 2020.
- ↑ 145.0 145.1 145.2 Dubiel, R.F. (1989). "Sedimentology and revised nomenclature of the Upper Triassic Chinle Formation and the Lower Jurassic Wingate Sandstone, northwestern New Mexico and northeastern Arizona". New Mexico Geological Society Field Conference Guidebook 40: 213–223. https://nmgs.nmt.edu/publications/guidebooks/downloads/40/40_p0213_p0223.pdf. Retrieved 12 November 2021.
- ↑ 146.0 146.1 Stewart, J.H.; Poole, F.G.; Wilson, R.F. (1972). "Stratigraphy and origin of the Chinle Formation and related Upper Triassic strata in the Colorado Plateau region, with sections on sedimentary petrology by R.A. Cadigan and conglomerate studies by William Thordarson and H.F. Albee". U.S. Geological Survey Professional Paper 690. doi:10.3133/pp690.
- ↑ Lucas, S.G.; Hunt, A.P. (1989). "Revised Triassic stratigraphy in the Tucumcari basin, east-central New Mexico". Dawn of the age of dinosaurs in the American southwest. New Mexico Museum of Natural History and Science. pp. 150–170. https://books.google.com/books?id=Ar1hCgAAQBAJ&pg=PA150. Retrieved 12 November 2021.
- ↑ 148.0 148.1 148.2 148.3 Sikitch, Steve W. (1965). "Upper Triassic stratigraphy in the eastern Uinta Mountains". The Mountain Geologist 2 (3): 167–172. http://archives.datapages.com/data/rmag/mg/1965/sikich.htm. Retrieved 30 October 2020.
- ↑ 149.0 149.1 149.2 Repenning, C.A.; Cooley, M.E.; Akers, J.P. (1969). "Stratigraphy of the Chinle and Moenkopi Formations, Navajo and Hopi Indian Reservations, Arizona, New Mexico, and Utah". U.S. Geological Survey Professional Paper. Professional Paper 521-B: B1–B34. doi:10.3133/pp521B.
- ↑ 150.0 150.1 150.2 150.3 150.4 Dubiel, R.F. (1987). "Sedimentology and new fossil occurrences of the Upper Triassic Chinle Formation, southeastern Utah". Four Corners Geological Society Field Conference Guidebook 10: 99–107. https://archives.datapages.com/data/fcgs/data/018/018001/99_four-corners180099.htm. Retrieved 12 November 2021.
- ↑ Dubiel, R.F. (1989). "Depositional and climatic setting of the Upper Triassic Chinle Formation, Colorado Plateau". Dawn of the Age of Dinosaurs in the American Southwest. New Mexico Museum of Natural History. pp. 171–187. https://books.google.com/books?id=Ar1hCgAAQBAJ&dq=Dawn+of+the+age+of+dinosaurs&pg=PP1. Retrieved 28 October 2021.
- ↑ O'Sullivan, R.B.; MacLachlan, M.E. (1975). "Triassic rocks of the Moab-White Canyon area, southeastern Utah". Four Corners Geological Society Field Conference Guidebook, 8th Field Conference (8): 129–141. https://archives.datapages.com/data/fcgs/data/013/013001/129_four-corners130129.htm. Retrieved 12 November 2021.
- ↑ Hintze, L.F.; Axen, G.J. (1995). "Geologic map of the Scarecrow Peak Quadrangle, Washington County, Utah, and Lincoln County, Nevada". U.S. Geological Survey Geologic Quadrangle Map GQ-1759. http://ngmdb.usgs.gov/Prodesc/proddesc_307.htm. Retrieved 16 November 2021.
- ↑ 154.0 154.1 Poole, F.G.; Stewart, J.H. (1964). "Chinle Formation and Glen Canyon Sandstone in northeastern Utah and northwestern Colorado". U.S. Geological Survey Professional Paper 501-D: D30–D39. doi:10.3133/pp501D.
- ↑ Lehman, T.M. (1994). "The saga of the Dockum Group and the case of the Texas/New Mexico boundary fault". New Mexico Bureau of Mines & Mineral Resources Bulletin 150: 37–51. https://geoinfo.nmt.edu/publications/monographs/bulletins/downloads/150/B150.pdf. Retrieved 1 September 2020.
- ↑ Cather, S.M.; Zeiger, Kate E.; Mack, Greg H.; Kelley, Shari A. (2013). "Toward standardization of Phanerozoic stratigraphic nomenclature in New Mexico". New Mexico Geological Society Spring Meeting: 12. https://citeseerx.ist.psu.edu/viewdoc/download?doi=10.1.1.667.3513&rep=rep1&type=pdf#page=14. Retrieved 26 August 2020.
- ↑ 157.0 157.1 157.2 "Tucumcari sheet". Geologic atlas of Texas. University of Texas-Austin, Bureau of Economic Geology. 1983.
- ↑ Lucas, Spencer G. (2021). "Triassic stratigraphy of the southeastern Colorado Plateau, west-central New Mexico". New Mexico Geological Society Field Conference Series 72: 229–240. https://nmgs.nmt.edu/publications/guidebooks/downloads/71/71_p0229_p0240.pdf. Retrieved 24 August 2021.
- ↑ "Archived copy". Retrieved 10 January 2011.
{{cite web}}
:|archive-date=
requires|archive-url=
(help) - ↑ 160.0 160.1 Yin Hongfu; Zhang Kexin; Tong Jinnan; Yang Zunyi; Wu Shunbao (June 2001). [http://www.stratigraphy.org/GSSP/Induan.pdf "The Global Stratotype Section and Point (GSSP) of the Permian-Triassic Boundary"]. Episodes 24 (2): 102-14. http://www.stratigraphy.org/GSSP/Induan.pdf. Retrieved 2015-01-20.
- ↑ Gradstein, F.M.; Ogg, J.G. & Smith, A.G. (2004). A Geologic Time Scale 2004. Cambridge University Press.
- ↑ The Nonmarine Permian: Volume 30 of Bulletin of the New Mexico Museum of Natural History and Science, page 48. Editors Spencer G. Lucas, Kate E. Zeigler, 2005
- ↑ Davydov, V.I.; Glenister, B.F.; Spinosa, C.; Ritter, S.M.; Chernykh, V.V.; Wardlaw, B.R. and Snyder, W.S. (1998). "Proposal of Aidaralash as Global Stratotype Section and Point (GSSP) for base of the Permian System". Episodes 21 (1): 11–18.
- ↑ 164.0 164.1 164.2 Olivier Béthoux, Jean Galtier, and André Nel (1 August 2004). "Earliest Evidence of Insect Endophytic Oviposition". Palaios 19 (4): 408-413. doi:10.1669/0883-1351(2004)019<0408:EEOIEO>2.0.CO;2. https://www.researchgate.net/profile/Olivier-Bethoux-2/publication/250082976_Earliest_Evidence_of_Insect_Endophytic_Oviposition/links/54fd9bfb0cf270426d12c5c5/Earliest-Evidence-of-Insect-Endophytic-Oviposition.pdf. Retrieved 12 October 2021.
- ↑ Gradstein, F.M.; Ogg, J.G. & Smith, A.G. (2004). A Geologic Time Scale 2004. Cambridge University Press.
- ↑ Davydov, V.I.; Glenister, B.F.; Spinosa, C.; Ritter, S.M.; Chernykh, V.V.; Wardlaw, B.R. & Snyder, W.S. (1998). "Proposal of Aidaralash as Global Stratotype Section and Point (GSSP) for base of the Permian System". Episodes 21 (1): 11-18. Archived on 2007-09-28. Error: If you specify
|archivedate=
, you must also specify|archiveurl=
. https://web.archive.org/web/20070928122140/http://www.episodes.org/backissues/211/11-18-vladimir.pdf. Retrieved 2007-09-28. - ↑ Chernykh, V.V.; Chuvashov, B.I.; Davydov, V.I.; Schmitz, M. & Snyder, W.S. (2006). "Usolka section (southern Urals, Russia): a potential candidate for GSSP to define the base of the Gzhelian Stage in the global chronostratigraphic scale". Geologija 49 (2): 205–217. Archived on 2007-12-14. Error: If you specify
|archivedate=
, you must also specify|archiveurl=
. https://web.archive.org/web/20071214152438/http://www.geo-zs.si/publikacije_arhiv/Clanki/Geologija_49_2/205-218_chernykh.pdf. Retrieved 2007-12-14. - ↑ François-Xavier Devuyst; Luc Hance; Hongfei Hou; Xianghe Wu; Shugang Tian; Michel Coen; George Sevastopulo (June 2003). "A proposed Global Stratotype Section and Point for the base of the Viséan Stage (Carboniferous): the Pengchong section, Guangxi, South China". Episodes 26 (2): 105. http://www.stratigraphy.org/GSSP/Tournaisian.pdf. Retrieved 2015-01-29.
- ↑ S. I. Kaiser (2009). "GSSP for Tournaisian Stage". Stratigraphy.org. Retrieved 2015-01-29.
- ↑ 170.00 170.01 170.02 170.03 170.04 170.05 170.06 170.07 170.08 170.09 170.10 170.11 170.12 170.13 170.14 170.15 Glass, D.J. (editor) 1997. Lexicon of Canadian Stratigraphy, vol. 4, Western Canada including eastern British Columbia, Alberta, Saskatchewan and southern Manitoba. Canadian Society of Petroleum Geologists, Calgary, 1423 p. on CD-ROM. ISBN 0-920230-23-7.
- ↑ 171.0 171.1 Canadian Society of Petroleum Geologists and Alberta Geological Survey (1994). "The Geological Atlas of the Western Canada Sedimentary Basin, Chapter 11: Beaverhill Lake Group of the Western Canada Sedimentary Basin". Compiled by Mossop, G.D. and Shetsen, I. Retrieved 2016-06-20.
{{cite web}}
:|archive-date=
requires|archive-url=
(help) - ↑ Norris, A.W. 1963. Devonian stratigraphy of northeastern Alberta and northwestern Saskatchewan. Geological Survey of Canada, Memoir 313.
- ↑ Barss, D.L., Copland, A.B., and Ritchie, W.D., 1970, Middle Devonian Reefs, Rainbow Area, Alberta, in Geology of Giant Petroleum Fields, AAPG Memoir 14, Halbouty, M.T., editor, Tulsa: American Association of Petroleum Geologists, pp. 18-49
- ↑ Hemphill, C.R., Smith, R.I., and Szabo, F., 1970, Geology of Beaverhill Lake Reefs, Swan Hills Area, Alberta, in Geology of Giant Petroleum Fields, AAPG Memoir 14, Halbouty, M.T., editor, Tulsa: American Association of Petroleum Geologists, pp. 50-90
- ↑ Various Contributors to the Paleobiology Database. "Fossilworks: Gateway to the Paleobiology Database". Retrieved 17 December 2021.
{{cite web}}
:|author=
has generic name (help); Paleozoic Era Canada. - ↑ G Klapper; R Feist; R T Becker; M R House (December 1993). "Definition of the Frasnian/Famennian Stage Boundary". Episodes 16 (4): 433-41. http://www.stratigraphy.org/GSSP/Famennian.pdf. Retrieved 2015-01-27.
- ↑ J.C. Gutiérrez-Marco; D. Goldman; J. Reyes-Abril; J. Gómez (2011). J.C. Gutiérrez-Marco, I. Rábano and D. García-Bellido. ed. A Preliminary Study of Some Sandbian (Upper Ordovician) Graptolites from Venezuela, In: Ordovician of the World. Madrid: Instituto Geológico y Minero de España. pp. 199-206. ISBN 978-84-7840-857-3. http://digital.csic.es/bitstream/10261/60947/1/ORDOVICIAN%20OF%20THE%20WORLD_199_206.pdf. Retrieved 2015-01-15.
- ↑ "GSSP Table - Paleozoic Era". Geologic TimeScale Foundation. Retrieved 24 November 2012.
- ↑ 179.0 179.1 Cooper, Roger; Nowlan, Godfrey; Williams, S. H. (March 2001). "Global Stratotype Section and Point for base of the Ordovician System". Episodes 24 (1): 19-28. doi:10.18814/epiiugs/2001/v24i1/005. https://stratigraphy.org/gssps/files/tremadocian.pdf. Retrieved 6 December 2020.
- ↑ McFarland, John David (2004). "Stratigraphic summary of Arkansas". Arkansas Geological Commission Information Circular 36: 18. Archived on 2016-12-21. Error: If you specify
|archivedate=
, you must also specify|archiveurl=
. https://web.archive.org/web/20161221195953/http://www.geology.ar.gov/pdf/IC-36_v.pdf. Retrieved 2018-01-05. - ↑ Paleozoic Sedimentary Successions of the Virginia Valley & Ridge and Plateau
- ↑ "Trempealeau Formation". USGS.
- ↑ USGS Mineral Resources On-Line Spatial Data, retrieved 4 Mar. 2011
- ↑ 184.0 184.1 Butts, Charles, 1918, Geologic section of Blair and Huntingdon Counties, central Pennsylvania: American Journal of Science, 4th series, v. 46, p. 523-537.
- ↑ 185.0 185.1 Wilson, J.L., 1952, Upper Cambrian stratigraphy in the central Appalachians: Geological Society of America Bulletin, v. 63, no. 3, p. 275-322.
- ↑ Berg, T.M. (compiler), 1980, Geologic map of Pennsylvania: Pennsylvania Geological Survey State Map, 4th series, 1, 1 sheet, scale 1:250,000 [1]
- ↑ USGS Mineral Resources On-Line Spatial Data, retrieved 4 Mar. 2011
- ↑ Tasch, Paul, 1951, Fauna and Paleoecology of the Upper Cambrian Warrior Formation of Central Pennsylvania, Journal of Paleontology, Vol. 25, No. 3, pp. 275-306, pls. 44-47, May 1951 abstract
- ↑ Zhengfu Zhao, Nicolas Thibault, Tais W. Dahl, Niels H. Schovsbo, Aske L. Sørensen, Christian M.Ø. Rasmussen, and Arne T. Nielsen (April 2021). Synchronizing Rock Clocks in the Cambrian, In: vEGU21, the 23rd EGU General Assembly. EGU21. Online: European Geosciences Union (EGU). pp. 5082. doi:10.5194/egusphere-egu21-5082. Bibcode: 2021EGUGA..23.5082Z. https://ui.adsabs.harvard.edu/abs/2021EGUGA..23.5082Z/abstract. Retrieved 24 March 2022.
- ↑ General Stratigraphic Column for Paleozoic Rocks in Indiana
- ↑ Thompson, Thomas L., 2001, Lexicon of Stratigraphic Nomenclature in Missouri, Missouri Department of Natural Resources, Division of Geology and Land Survey, Report of Investigation Number 73, p 229
- ↑ Thompson, Thomas L., 2001, Lexicon of Stratigraphic Nomenclature in Missouri, Missouri Department of Natural Resources, Division of Geology and Land Survey, Report of Investigation Number 73, p 83-4
- ↑ Griswold, L.S. (1892). "Whetstones and the novaculites". Annual Report of the Geological Survey of Arkansas for 1890 3.
- ↑ Purdue, Albert Homer (1909). Slates of Arkansas. Geological Survey of Arkansas. pp. 30, 31.
- ↑ Purdue, Albert Homer (1909). "Structure and stratigraphy of the Ouachita Ordovician area, Arkansas (abstract)". Geological Society of America Bulletin 19: 557. doi:10.1130/GSAB-19-513.
- ↑ I. V. Korovnikov (may 2014). [https://www.researchgate.net/profile/Iv-Korovnikov/publication/264979180_Trilobites_Plicatolina_lucida_Lazarenko_from_the_Upper_Cambrian_of_the_Kharaulakh_Mountains_Northeastern_Siberian_Platform/links/53faba700cf27c365cf03f8e/Trilobites-Plicatolina-lucida-Lazarenko-from-the-Upper-Cambrian-of-the-Kharaulakh-Mountains-Northeastern-Siberian-Platform.pdf "Trilobites Plicatolina lucida Lazarenko from the Upper Cambrian of the Kharaulakh Mountains (Northeastern Siberian Platform)"]. Paleontological Journal 48 (5): 465-470. doi:10.1134/S0031030114050050. https://www.researchgate.net/profile/Iv-Korovnikov/publication/264979180_Trilobites_Plicatolina_lucida_Lazarenko_from_the_Upper_Cambrian_of_the_Kharaulakh_Mountains_Northeastern_Siberian_Platform/links/53faba700cf27c365cf03f8e/Trilobites-Plicatolina-lucida-Lazarenko-from-the-Upper-Cambrian-of-the-Kharaulakh-Mountains-Northeastern-Siberian-Platform.pdf. Retrieved 24 March 2022.
- ↑ "GSSP for Jiangshanian". Retrieved 12 November 2012.
- ↑ Russell E. Clemons and Greg H. Mack (Fall 1988). Mack, G. H.; Lawton, T. F.; Lucas, S. G.. ed. Geology of Southwestern New Mexico, In: Cretaceous and Laramide Tectonic Evolution of Southwestern New Mexico. New Mexico Geological Society. pp. 45-57. https://nmgs.nmt.edu/publications/guidebooks/downloads/39/39_p0045_p0057.pdf. Retrieved 8 April 2022.
- ↑ "Lithological Unit Search". Lexicon of Canadian Geologic Units. Natural Resources Canada. Retrieved 1 August 2018.
- ↑ Collom, C. J.; Johnston, P. A.; Powell, W. G. (2009). "Reinterpretation of 'Middle' Cambrian stratigraphy of the rifted western Laurentian margin: Burgess Shale Formation and contiguous units (Sauk II Megasequence); Rocky Mountains, Canada". Palaeogeography, Palaeoclimatology, Palaeoecology 277 (1–2): 63–85. doi:10.1016/j.palaeo.2009.02.012.
- ↑ 201.0 201.1 201.2 201.3 Slind, O.L., Andrews, G.D., Murray, D.L., Norford, B.S., Paterson, D.F., Salas, C.J., and Tawadros, E.E., Canadian Society of Petroleum Geologists and Alberta Geological Survey (1994). "The Geological Atlas of the Western Canada Sedimentary Basin (Mossop, G.D. and Shetsen, I., compilers), Chapter 8: Middle Cambrian and Early Ordovician Strata of the Western Canada Sedimentary Basin". Retrieved 2018-07-13.
{{cite web}}
: CS1 maint: multiple names: authors list (link) - ↑ Fletcher, T. P.; Collins, D. (1998). "The Middle Cambrian Burgess Shale and its relationship to the Stephen Formation in the southern Canadian Rocky Mountains". Canadian Journal of Earth Sciences 35 (4): 413–436. doi:10.1139/cjes-35-4-413.
- ↑ 203.0 203.1 Shanchi Peng; Loren E. Babcock; Jingxun Zuo; Huanling Lin; Xuejian Zhu; Xianfeng Yang; Richard A. Robison; Yuping Qi et al. (March 2009). "The Global Boundary Stratotype Section and Point (GSSP) of the Guzhangian Stage (Cambrian) in the Wuling Mountains, Northwestern Hunan, China". Episodes 32 (1): 41-55. http://www.stratigraphy.org/GSSP/Guzhangian.pdf. Retrieved 2015-01-21.
- ↑ Peng, S.; Robison, R.A. (2000). "Agnostid biostratigraphy across the Middle-Upper Cambrian boundary in Hunan, China". Journal of Paleontology Memoir 53. cited in Austin Hendy. "Paibi section, bed 37a". Fossilworks. Retrieved 17 December 2021.
- ↑ 205.0 205.1 Walcott, C.D. 1908. Cambrian Geology and Palaeontology. Smithsonian Museum, Miscellaneous Collections, 53.
- ↑ Leckie, D.A. 2017. Rocks, ridges and rivers – Geological wonders of Banff, Yoho, and Jasper National Parks. Brokenpoplars, Calgary, Alberta, 217 pp. ISBN 978-0-9959082-0-8.
- ↑ Aitken, J.D. 1971. Control of lower Paleozoic sedimentary facies by the Kicking Horse Rim, southern Rocky Mountains, Canada. Bulletin of Canadian Petroleum Geology, vol. 19, no. 3, p. 557-569.
- ↑ Alberta Geological Survey, 2019. "Alberta Table of Formations". Alberta Energy Regulator. Retrieved 2 July 2019.
- ↑ 209.0 209.1 Chronic, Halka (1983). Roadside Geology of Arizona. Seattle, Washington: The Mountaineers Books. https://archive.org/details/roadsidegeology000chro.
- ↑ "Muav Lexicon entry". National Geologic Map Database Lexicon. United States Geological Survey. Retrieved 2 June 2019.
- ↑ "Tapeats Lexicon entry". National Geologic Map Database Lexicon. United States Geological Survey. Retrieved 2 June 2019.
- ↑ Geology graphic of Pioche Shale & Bright Angel Shale at 3dpards.wr.usgs.gov [2]
- ↑ "Bright Angel Lexicon entry". National Geologic Map Database Lexicon. United States Geological Survey. Retrieved 2 June 2019.
- ↑ {{cite book |last1=Hampton|first1=HM|chapter=Geologic Map of the Grand Canyon in the Vicinity of the South Rim Visitor Center|editor1-last=Kamilli |editor1-first=Robert J.|editor2-last=Richard |editor2-first=Stephen M. |date=1998 |title=Geologic Highway Map of Arizona |publisher=Arizona Geological Society and Arizona Geological Survey |ISBN 978-1-8919-2400-2 1 sheet, scale 1:62,500.
- ↑ Various Contributors to the Paleobiology Database. "Fossilworks: Gateway to the Paleobiology Database". Retrieved 17 December 2021.
{{cite web}}
:|author=
has generic name (help) - ↑ 216.0 216.1 Moore, R. A.; Lieberman, B. S. (2009). "Preservation of Early and Middle Cambrian soft-bodied arthropods from the Pioche Shale, Nevada, USA". Palaeogeography, Palaeoclimatology, Palaeoecology 277: 57–62. doi:10.1016/j.palaeo.2009.02.014.
- ↑ 217.0 217.1 Lieberman, B. S. (2003). "A New Soft-Bodied Fauna: the Pioche Formation of Nevada". Journal of Paleontology 77 (4): 674–690. doi:10.1666/0022-3360(2003)077<0674:ANSFTP>2.0.CO;2. ISSN 0022-3360.
- ↑ Sundberg, F. A.; McCollum, L. B. (2000). "Ptychopariid Trilobites of the Lower-Middle Cambrian Boundary Interval, Pioche Shale, Southeastern Nevada". Journal of Paleontology (Paleontological Society) 74 (4): 604–630. doi:10.1666/0022-3360(2000)074<0604:PTOTLM>2.0.CO;2.
- ↑ Robison, R. A.; Wiley, E. O. (1995). "A New Arthropod, Meristosoma: More Fallout from the Cambrian Explosion". Journal of Paleontology 69 (3): 447–459. doi:10.1017/s0022336000034855.
- ↑ 220.0 220.1 Kimmig, J., Strotz, L.C., Kimmig, S.R., Egenhoff, S.O., Lieberman, B.S. 2019. The Spence Shale Lagerstätte: an important window into Cambrian biodiversity. Journal of the Geological Society of London, 176, 609–619
- ↑ Stoyanow, A. (1958). "Sonoraspis and Albertella in the Inyo Mountains, California". Geological Society of America Bulletin 69 (3): 347–352. doi:10.1130/0016-7606(1958)69[347:SAAITI]2.0.CO;2. ISSN 0016-7606.
- ↑ 222.0 222.1 222.2 Loren E. Babcock; Richard A. Robison; Margaret N. Rees; Shanchi Peng; Matthew R. Saltzman (June 2007). "The Global boundary Stratotype Section and Point (GSSP) of the Drumian Stage (Cambrian) in the Drum Mountains, Utah, USA". Episodes 30 (2): 84-94. http://www.palaeobiology.geo.uu.se/ISCS/Drumian%20GSSP.pdf. Retrieved 2016-10-26.
- ↑ 223.0 223.1 223.2 Jean-Bernard Caron; Martin R. Smith; Thomas H. P. Harvey (31 July 2013). "Beyond the Burgess Shale: Cambrian microfossils track the rise and fall of hallucigeniid lobopodians". Proceedings of the Royal Society B 280 (1767): 1613. doi:10.1098/rspb.2013.1613. http://rspb.royalsocietypublishing.org/content/280/1767/20131613.short. Retrieved 2016-10-26.
- ↑ SemperBlotto (4 March 2007). "Burgess Shale". San Francisco, California: Wikimedia Foundation, Inc. Retrieved 29 March 2022.
{{cite web}}
:|author=
has generic name (help) - ↑ Butterfield, N. J. (2003-02-01). "Exceptional Fossil Preservation and the Cambrian Explosion". Integrative and Comparative Biology 43 (1): 166–177. doi:10.1093/icb/43.1.166. ISSN 1540-7063. https://academic.oup.com/icb/article/43/1/166/604533.
- ↑ Gabbott, Sarah E. (2001). Exceptional Preservation. doi:10.1038/npg.els.0001622. ISBN 978-0-470-01590-2.
- ↑ Butterfield, N.J. (2006). "Hooking some stem-group" worms": fossil lophotrochozoans in the Burgess Shale". BioEssays 28 (12): 1161–6. doi:10.1002/bies.20507. PMID 17120226.
- ↑ 228.0 228.1 228.2 228.3 228.4 Lauren Pouille, Olga Obut, Taniel Danelian and Nikolay Sennikov (November 2011). "Lower Cambrian (Botomian) polycystine Radiolaria from the Altai Mountains (southern Siberia, Russia)". Comptes Rendus Palevol 10 (8): 627-633. doi:10.1016/j.crpv.2011.05.004. https://www.sciencedirect.com/science/article/pii/S1631068311001011. Retrieved 18 March 2022.
- ↑ Paleozoic Sedimentary Successions of the Virginia Valley & Ridge and Plateau
- ↑ Paleozoic Sedimentary Successions of the Virginia Valley & Ridge and Plateau
- ↑ Paleozoic Sedimentary Successions of the Virginia Valley & Ridge and Plateau
- ↑ McMenamin, Mark A.S.; Debrenne, Françoise; Zhuravlev, Andrey Yu. (2000). "Early Cambrian Appalachian Archaeocyaths: Further age constraints from the fauna of New Jersey and Virginia, U.S.A.". Geobios 33 (6): 693–708. doi:10.1016/S0016-6995(00)80123-0. https://zenodo.org/record/259455.
- ↑ Fritz, W. H.; Yochelson, Ellis L. (1988-03-01). "The status of Salterella as a Lower Cambrian index fossil". Canadian Journal of Earth Sciences 25 (3): 403–416. doi:10.1139/e88-042. ISSN 0008-4077.
- ↑ Kobluk, David R. (1985). "Biota Preserved within Cavities in Cambrian Epiphyton Mounds, Upper Shady Dolomite, Southwestern Virginia". Journal of Paleontology 59 (5): 1158–1172. ISSN 0022-3360.
- ↑ J. F. Read, R. W. Pfeil (2) (1983). "Fabrics of Allochthonous Reefal Blocks, Shady Dolomite (Lower to Middle Cambrian), Virginia Appalachians". SEPM Journal of Sedimentary Research 53. doi:10.1306/212F82B5-2B24-11D7-8648000102C1865D. ISSN 1527-1404. https://pubs.geoscienceworld.org/jsedres/article/53/3/761-778/97586.
- ↑ Byrd, W J; Weinberg, E L; Yochelson, Ellis L (1973). "Salterella in the Lower Cambrian Shady Dolomite of southwestern Virginia". American Journal of Science 273A: 252–260. http://earth.geology.yale.edu/~ajs/1973/ajs_273A_11.pdf/252.pdf.
- ↑ Willoughby, Ralph (1977). Paleontology and stratigraphy of the Shady Formation near Austinville. Virginia Polytechnic Institute and State University, Blacks- burg, Virginia. pp. 189.
- ↑ Keith, Arthur, (1903) Description of the Cranberry quadrangle [North Carolina-Tennessee]: U. S. Geological Survey Geology Atlas, Folio 90.
- ↑ "Division of Geology and Mineral Resources - The New Jersey Zinc Company". dmme.virginia.gov. Retrieved 2020-05-09.
- ↑ Cook, Harry E., ed (1977). Deep-water Carbonate Environments. SEPM (Society for Sedimentary Geology). doi:10.2110/pec.77.25.0083. ISBN 978-1-56576-155-1. http://sp.sepmonline.org/content/sepspecpub/sepspdee/1.toccontent/25.
- ↑ 241.0 241.1 Barnaby, Roger J.; Read, J. Fred (1990-03-01). "Carbonate ramp to rimmed shelf evolution: Lower to Middle Cambrian continental margin, Virginia Appalachians". GSA Bulletin 102 (3): 391–404. doi:10.1130/0016-7606(1990)102<0391:CRTRSE>2.3.CO;2. ISSN 0016-7606. https://pubs.geoscienceworld.org/gsabulletin/article-abstract/102/3/391/182420/Carbonate-ramp-to-rimmed-shelf-evolution-Lower-to.
- ↑ 242.0 242.1 R. W. Pfeil (2), J. F. Read (1980). "Cambrian Carbonate Platform Margin Facies, Shady Dolomite, Southwestern Virginia, U.S.A.". SEPM Journal of Sedimentary Research 50. doi:10.1306/212F7978-2B24-11D7-8648000102C1865D. ISSN 1527-1404. https://pubs.geoscienceworld.org/jsedres/article/50/1/91-116/97393.
- ↑ Read, J Fred; Repetski, John E (2012). Derby, James Richard; Fritz, R D; Longacre, Susan Ann et al.. eds. "Cambrian–Lower Middle Ordovician Passive Carbonate Margin, Southern Appalachians". AAPG Memoir 98: 357–382. doi:10.1306/13331499M980271. ISBN 978-1-6298-1020-1. https://pubs.geoscienceworld.org/books/book/1267/chapter/107100018/Cambrian-Lower-Middle-Ordovician-Passive-Carbonate.
- ↑ Paleobiology Database
- ↑ F. R. Abe, B. S. Lieberman, M. C. Pope, K. Dilliard (2010). "New information on olenelline trilobites from the Cambrian Sekwi Formation in northwestern Canada". Canadian Journal of Earth Sciences, 2010, 47 (12): 1445–1449.[3]
- ↑ Life Desks, Trilobites on line
- ↑ Life Desks, Trilobites on line
- ↑ U. S. National Museum, Catalogue No. 60082.
- ↑ Srivastava, Purnima (June 2012). "Treptichnus pedum: An Ichnofossil Representing Ediacaran - Cambrian Boundary in the Nagaur Group, the Marwar Supergroup, Rajasthan, India". Proc. Indian Natl. Sci. Acad. 78 (2): 161–169. http://www.insa.nic.in/writereaddata/UpLoadedFiles/PINSA/Vol78_2012_2_Art04_161_169.pdf. Retrieved April 8, 2016.
- ↑ Subcommission on Neoproterozoic Stratigraphy - URL retrieved June 22, 2009
- ↑ International Commission on Stratigraphy, International Chronostratigraphic Chart, 2012, http://www.stratigraphy.org/column.php?id=Chart/Time%20Scale
- ↑ AK Rindsberg and DC Kopaska-Merkel. 2005. Treptichnus and Arenicolites from the Steven C. Minkin Paleozoic Footprint Site (Langsettian, Alabama, USA). Alabama Paleontological Society Monograph 1 : 121-141
- ↑ Hamblin, A. P. (2011). "Detailed outcrop and core measured sections of Upper Cambrian and Middle Ordovician sandstones (and associated facies), southwestern Ontario". Geological Survey of Canada. doi:10.4095/288671.
- ↑ Camp, Mark J. (2006-10-15). Roadside Geology of Ohio (1st ed.). Missoula, MT: Mountain Press. pp. 75–76. ISBN 9780878425242. https://www.amazon.com/Roadside-Geology-Ohio/dp/0878425241.
- ↑ 255.0 255.1 James G. Gehling; Mary L. Droser (March 2012). "Ediacaran stratigraphy and the biota of the Adelaide Geosyncline, South Australia". Episodes 35 (1): 236-46. http://www.episodes.co.in/contents/2012/march/p236-246.pdf. Retrieved 2015-01-19.
- ↑ "Chart". International Commission on Stratigraphy. Retrieved 2017-02-14.
{{cite web}}
:|archive-date=
requires|archive-url=
(help) - ↑ Lucchitta, 2001. Hiking Arizona's Geology, Hike 18, Aztec Peak Trail, Fig. 19, Formations of the Apache Group of Proterozoic age, p. 147.
- ↑ R. W. Ojakangas (1979-10-01). Sedimentation of the basal Kombolgie Formation (Upper Precambrian-Carpentarian) Northern Territory, Australia: possible significance in the genesis of the underlying Alligator Rivers unconformity-type uranium deposits. Duluth (USA): Minnesota Univ., Duluth (USA). Dept. of Geology. doi:10.2172/5722569. https://www.osti.gov/servlets/purl/5722569. Retrieved 12 March 2019.
- ↑ 259.0 259.1 M. Kralik (May 1982). "Rb-Sb age determinations on Precambrian carbonate rocks of the Carpentarian McArthur basin, Northern Territories, Australia". Precambrian Research 18 (1–2): 157-170. doi:10.1016/0301-9268(82)90044-4. https://www.sciencedirect.com/science/article/pii/0301926882900444. Retrieved 13 March 2019.
- ↑ 260.0 260.1 David P. Hawkins, Samuel A. Bowring, Bradley R. Ilg, Karl E. Karlstrom, and Michael L. Williams (September 1996). "U-Pb geochronologic constraints on the Paleoproterozoic crustal evolution of the Upper Granite Gorge, Grand Canyon, Arizona". Geological Society of America Bulletin 108 (9): 1167-1181. https://www.researchgate.net/profile/Bradley-Ilg/publication/322328128_Grand_Canyon_Geology/links/5a5a8ad7aca2727d608611d2/Grand-Canyon-Geology.pdf. Retrieved 5 April 2022.
- ↑ Malcolm S. W. Hodgskiss, Peter W. Crockford, Yongbo Peng, Boswell A. Wing, and Tristan J. Horner (27 August 2019). "A productivity collapse to end Earth’s Great Oxidation". Proceedings of the National Academy of Sciences USA 116 (35): 17207-12. doi:10.1073/pnas.1900325116. https://www.pnas.org/content/116/35/17207. Retrieved 7 September 2019.
- ↑ 262.0 262.1 262.2 262.3 "The eons of Chaos and Hades" (PDF). Solid Earth. 26 January 2010.
- ↑ Borenstein, Seth (19 October 2015). "Hints of life on what was thought to be desolate early Earth". Excite. Yonkers, NY: Mindspark Interactive Network. Associated Press. Retrieved 2015-10-20.
- ↑ Bell, Elizabeth A.; Boehnike, Patrick; Harrison, T. Mark; Mao, Wendy L. (19 October 2015). "Potentially biogenic carbon preserved in a 4.1 billion-year-old zircon". Proc. Natl. Acad. Sci. U.S.A. (Washington, D.C.: National Academy of Sciences) 112 (47): 14518–21. doi:10.1073/pnas.1517557112. ISSN 1091-6490. PMID 26483481. PMC 4664351. http://www.pnas.org/content/early/2015/10/14/1517557112.full.pdf. Retrieved 2015-10-20.
- ↑ Chang, Kenneth (December 2, 2008). "A New Picture of the Early Earth". The New York Times.
- ↑ Abramov, Oleg; Mojzsis, Stephen J. (December 2008). "Thermal State of the Lithosphere During Late Heavy Bombardment: Implications for Early Life". AGU Fall Meeting Abstracts (Fall Meeting 2008: American Geophysical Union) 1 (2008 Fall Meeting): V11E–08.
- ↑ 267.0 267.1 Gibbs, A.K & Barron, C.N (1993). The Geology of the Guiana Shield. Oxford University Press.
- ↑ Nadeau, Serge; Chen, Wei; Reece, Jimmy; Lachhman, Deokumar; Ault, Randy; Faraco, Maria; Fraga, Leda; Reis, Nelson et al. (2013-12-01). "Guyana: the Lost Hadean crust of South America?". Brazilian Journal of Geology 43 (4): 601–606. doi:10.5327/Z2317-48892013000400002.
- ↑ 269.0 269.1 Wilde, Simon A.; Valley, John W.; Peck, William H.; Graham, Colin M. (2001). "Evidence from detrital zircons for the existence of continental crust and oceans on the Earth 4.4 Gyr ago". Nature 409 (6817): 175–178. doi:10.1038/35051550. PMID 11196637.
- ↑ "Hell's milder side". Research School of Earth Sciences. Australian National University. Archived from the original on 2006-06-21.
"There was no such thing as hell on Earth". Media Release: Marketing & Communications. Australian National University. 18 November 2005.{{cite web}}
:|archive-date=
requires|archive-url=
(help)
Valley, John W.; Peck, William H.; King, Elizabeth M.; Wilde, Simon A. (April 2002). "A Cool Early Earth". Geology 30 (4): 351–354. doi:10.1130/0091-7613(2002)030<0351:ACEE>2.0.CO;2. PMID 16196254. Archived on 2013-06-16. Error: If you specify|archivedate=
, you must also specify|archiveurl=
. https://web.archive.org/web/20130616213221/http://www.geology.wisc.edu/~valley/zircons/cool_early/cool_early_home.html. Retrieved 2006-08-22. - ↑ Van Kranendonk, Martin J. (2012), Altermann, Wladyslaw; Beard, Brian L.; Hoffman, Paul F.; Johnson, Clark M.; Kasting, James F.; Melezhik, Victor A.; Nutman, Allen; Papineau, Dominic; Pirajno, Franco, "A Chronostratigraphic Division of the Precambrian", The Geologic Time Scale, Elsevier, pp. 299–392, doi:10.1016/b978-0-444-59425-9.00016-0, retrieved 2020-07-31
{{citation}}
: Text "ISBN 978-0-444-59425-9" ignored (help) - ↑ Goldblatt, C.; Zahnle, K.J.; Sleep, N.H.; Nisbet, E.G. (2 February 2010). "The Eons of Chaos and Hades". Solid Earth 1 (1): 1–3. doi:10.5194/se-1-1-2010. https://www.solid-earth.net/1/1/2010/se-1-1-2010.html.
External links
[edit | edit source]- GeoWhen Database
- Global Boundary Stratotype Section and Point (GSSP) of the International Commission on Stratigraphy