Hestiominerals

Numerous fragments of 4 Vesta were ejected by collisions one and two billion years ago that left two enormous craters occupying much of Vesta's southern hemisphere.[1][2] Debris from these events has fallen to Earth as howardite–eucrite–diogenite (HED) meteorites, which have been a rich source of information about Vesta.[3][4][5]
Vesta's Greek equivalent is Hestia.[6] Minerals likely to have originated on the asteroid 4 Vesta may be called Hestiominerals.
V asteroids[edit | edit source]


In the full image of Vesta at right, the rocky-object appears to have suffered from meteor damage.
As NASA's Dawn spacecraft took off for its next destination, this mosaic synthesizes some of the best views the spacecraft had of the giant asteroid Vesta. Dawn studied Vesta from July 2011 to September 2012. The towering mountain at the south pole — more than twice the height of Mount Everest — is visible at the bottom of the image. The set of three craters known as the "snowman" can be seen at the top left.
Vesta, minor-planet designation 4 Vesta, is one of the largest asteroids in the Solar System. It lost some 1% of its mass less than a billion years ago in a collision that left an enormous crater occupying much of its southern hemisphere. Debris from this event has fallen to Earth as howardite–eucrite–diogenite (HED) meteorites, a rich source of information about the asteroid.[4][5]
"V-type asteroids are bodies whose surfaces are constituted of basalt. In the Main Asteroid Belt, most of these asteroids are assumed to come from the basaltic crust of Asteroid (4) Vesta."[7]
The Vestian asteroids consist "of 4 Vesta, the second-most-massive of all asteroids (mean diameter of 530 km), and many small asteroids below 10 km diameter. The brightest of these, 1929 Kollaa and 2045 Peking, have an absolute magnitude of 12.2, which would give them a radius of about 7.5 km assuming the same high albedo as 4 Vesta."[8]
"A [Hierarchical Clustering Method] (HCM) numerical analysis (by Zappala 1995) determined a large group of 'core' family members, whose proper orbital elements lie in the approximate ranges"[8]
ap | ep | ip | |
---|---|---|---|
min | 2.26 AU | 0.075 | 5.6° |
max | 2.48 AU | 0.122 | 7.9° |
"This gives the approximate boundaries of the family. At the present epoch, the range of osculating orbital elements of these core members is"[8]
a | e | i | |
---|---|---|---|
min | 2.26 AU | 0.035 | 5.0° |
max | 2.48 AU | 0.162 | 8.3° |
"The Zappala 1995 analysis[9] found 235 core members. A search of a recent proper-element database (AstDys) for 96944 minor planets in 2005 yielded 6051 objects (about 6% of the total) lying within the Vesta-family region as per the first table above."[8]
Howardite–eucrite–diogenite meteorites[edit | edit source]
"Approximately 1,700 HED meteorites have been identified in the meteorite collections; however, high-pressure minerals have only been reported in one shocked eucrite18."[10]
"Using Dawn’s Gamma Ray and Neutron Detector, [elemental mapping on Vesta has been accomplished.] Global Fe/O and Fe/Si ratios are consistent with [howardite, eucrite, and diogenite] HED [meteorite] compositions."[11]
Diogenites[edit | edit source]

Def. an "achondritic stony meteorite originating from deep within the crust of the asteroid 4 Vesta"[12] is called a diogenite.
Diogenites are composed of igneous rocks of plutonic origin, having solidified slowly enough deep within Vesta's crust to form crystals which are larger than in the eucrites, which are primarily magnesium-rich orthopyroxene (enstatite), with small amounts of plagioclase and olivine.[13]
"The remaining six are interpreted as diogenitic in composition and were designated as J asteroids (mnemonic for the Johnstown diogenite)."[14]
Eucrites[edit | edit source]

Def. an "achondritic meteoritic rock consisting chiefly of pigeonite and anorthite"[15] is called a eucrite.
The eucrite specimen at top right is approximately 6 cm wide. Note the shiny black fusion crust with flow lines. The chip at lower right allows one to see the light-gray interior. The orange staining at top is a result of weathering, as these stones were not recovered until many years after they fell.
Eucrites consist of basaltic rock from the crust of 4 Vesta or a similar parent body, mostly composed of Ca-poor pyroxene, pigeonite, and Ca-rich plagioclase (anorthite).[16]
NWA 8003[edit | edit source]
"[T]issintite, vacancy-rich clinopyroxene, and super-silicic garnet in the eucrite Northwest Africa (NWA) 8003 [combined] with coesite and stishovite, the presence of these high-pressure minerals and their chemical compositions reveal that solidification of melt veins in NWA 8003 began at a pressure of >~10 GPa and ceased when the pressure dropped to <~8.5 GPa."[10]
Howardites[edit | edit source]

Def. an "achondritic stony meteorite"[17] is called a howardite.
"High precision spectrophotometry of 4 Vesta, the third largest asteroid, was used to establish the surface composition of this body and to investigate mineralogical variations across its surface."[18]
"The average surface of Vesta is analogous to howardite and/or polymict eucrite assemblages, regolith-derived members of the HED meteorite suite which consist of a eucrite matrix containing differing amounts of a diogenite component."[18]
"Color and/or spectral changes, which exhibit a consistent relationship to rotational phasing, are evident in 16 of 18 studies of Vesta dating back to 1929, including the present work. After elimination of possible sources of spurious spectral or color changes, it is concluded that these variations arise from hemispheric variations in surface materials, and hence provide a means of spatially resolving subhemispheric compositional units on the surface of Vesta."[18]
"The background surface of Vesta is a relatively dark howardite or polymict eucrite (pyroxene–plagioclase) assemblage with several compositionally distinct bright regions clustered in one hemisphere viewed around the maximum in Vesta's lightcurve. These include what appears to be an olivine-bearing unit (suggested name “Leslie Formation”) located near Vesta's equator which probably represents an impact basin (and/or its associated ejecta) that penetrated through the basaltic crust. Other high-albedo compositional units including an apparently low-calcium eucrite region and several diogenite (pyroxenite) regions, at least one located near the southern pole, may be smaller, shallower impact basins."[18]
"By analogy to the eucrite meteorites, which represent surface flows or shallow intrusions and which constitute the major component of the regolith-derived howardites and polymict eucrites, [...] the howardite/polymict eucrite units represent a regolith-gardened original surface of Vesta. It is probable that the low albedo of the background surface on Vesta is due to an age-related darkening effect similar to that inferred from the Galileo images of Gaspra and Ida. This mechanism is consistent with the correlation of absorption feature intensity with the lightcurve. Vesta appears to have an old eucritic surface, darkened with age and represented among the meteorites by the regolith-derived howardites and polymict eucrites, on which several impacts on one hemisphere have exposed fresher brighter diogenite and olivine-bearing material."[18]
"Based on qualitative analysis of the mineralogical variations as a function of rotation, a generalized lithologic map of Vesta was produced. There is reasonable control on the longitude of lithologic features but little control on their latitudes, except where specifically noted. In producing this map, discrete circular features were used on the plausible assumption that impacts have been the most important geologic process on this surface for most of the age of the solar system. The shape of the features has thus been assumed rather than derived and is subject to future revision as data improve."[18]
Maskelynites[edit | edit source]

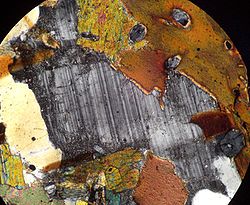
Maskelynite was first identified in the Shergotty meteorite by Gustav Tschermak von Seysenegg (1872) as an isotropic glass of an unknown origin with near labradorite composition.[19] Similar phases were found in chondrites and Martian meteorites. In 1963, D. J. Milton and P. S. de Carli produced a maskelynite-like glass by subjecting gabbro to an explosive shock wave.[20]
"Maskelynite occurs in the rocks of the central peaks of Clearwater West and Manicouagan craters, Quebec, Canada."[21]
"A clear, glassy pseudomorph of plagioclase was described in the Shergotty basaltic achondrite by Tschermak (1872) and named maskelynite."[21]
Maskelynite is "a transformation of plagioclase produced by remelting or by mechanical means."[21]
"A mechanical transformation of Stillwater gabbro to a rock resembling shergottite was achieved by Milton and DeCarli (1963) by shock-loading the gabbro at 250 to 350 kb peak pressure and a calculated temperature of no more than 350°C. They advocated restricting the term maskelynite to a non-crystalline phase clearly pseudomorphous after crystalline feldspar. Duke (1963) restudied the Shergotty meteorite in detail and suggested the maskelynite resulted from a passage of a strong shock wave through the meteorite."[21]
"Terrestrial samples containing isotropic material conforming to Milton and DeCarli's definition have been collected from two large probable ancient meteorite craters in the Canadian Shield (Beals, et al. 1963)."[21]
Plagioclase is a series of tectosilicate minerals within the feldspar group with a specific chemical composition: NaAlSi
3O
8 – CaAl
2Si
2O
8. Plagioclase in hand samples is often identified by its polysynthetic crystal twinning or 'record-groove' effect.
Analysis of thermal emission spectra from the surface of Mars suggests that plagioclase is the most abundant mineral in the crust of Mars.[22]
Anorthites[edit | edit source]

Anorthites have the chemical formula CaAl
2Si
2O
8.[23]
Anorthite is rare on the Earth[24] but abundant on the Moon.[25]
Anorthite is a rare compositional variety of plagioclase that occurs in mafic igneous rock, also occurs in metamorphic rocks of granulite facies, in metamorphosed carbonate rocks, and corundum deposits,[26] type localities are Monte Somma and Valle di Fassa, Italy. It was first described in 1823,[27] more rare in surficial rocks than it normally would be due to its high weathering potential in the Goldich dissolution series.
It also makes up much of the lunar highlands; the Genesis Rock, collected during the 1971 Apollo 15 mission, is made of anorthosite, a rock composed largely of anorthite. Anorthite was discovered in samples from comet Wild 2, and the mineral is an important constituent of Ca-Al-rich inclusions in rare varieties of chondritic meteorites.
Augites[edit | edit source]
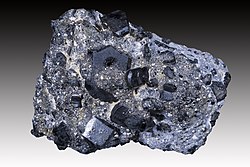
Formula: (Ca
xMg
yFe
z)(Mg
y1Fe
z1)Si
2O
6, where 0.4 ≤ x ≤ 0.9, x+y+z=1 and y1+z1=1.[28]
IMA Formula: (Ca,Mg,Fe)
2Si
2O
6.[28]
Crystal System: Monoclinic, Clinopyroxene Subgroup > Pyroxene Group.[28]
Common Impurities: Ti,Cr,Na,Mn,K.[28]
Geological Setting: Major rock forming mineral in mafic igneous rocks, ultramafic rocks, and some high-grade metamorphic rocks.[28]
Augite is an essential mineral in mafic igneous rocks; for example, gabbro and basalt and common in ultramafic rocks, occurs in relatively high-temperature metamorphic rocks such as mafic granulite and metamorphosed iron formations, commonly occurs in association with orthoclase, sanidine, labradorite, olivine, leucite, amphiboles and other pyroxenes.[29]
Clinopyroxenes[edit | edit source]
"A subgroup name for monoclinic Pyroxene Group minerals."[30]
"The most widespread members include aegirine, augite, hedenbergite and diopside."[30]
"In the Ca-Fe-Mg (diopside-hedenbergite-augite) quadrilateral, the ideal structural formula is M2M1[Si
2O
6], where M2 is a distorted octahedral site which preferentially accepts large cations such as alkaline earth/alkali atoms (Ca, but may accept transition metals) and where M1 is a smaller regular octahedral site preferentially accepting transition metals, etc. (most commonly Mg, Fe2+
). The substitutions containing no changes in valence within M2 (2+), Mi (2+), and tetrahedral sites, T (4+), is the normal scheme. A variety of additional substitution schemes, which require coupling of substituents, have been identified in these pyroxenes. Often these substitutions result in a change of species designation when they are dominant."[28]
"Substitution 1 has Na substitution in M2 and trivalent ions of Al, Fe, Cr, and Sc substituting in M1."[28]
"Substitution 2 involves Na substitution in M2 and mixed valance transition metals such as [Fe2+
0.5Ti4+
0.5] substituting in M1."[28]
"Substitution 3, also called Tschermak's Component, involves coupled M1 octahedral and tetrahedral substitutions, such as CaAl[AlSiO
6] or CaTi3+
[AlSiO
6]."[28]
"Substitution 4 is a variant of Substitution 2 and has dominant Ca in M2 with mixed valence substitution in M1 involving divalent and tetravalent Ti and Al substitution in tetrahedral sites, e.g. Ca[(Mg, Fe2+
0.5Ti4+
0.5)][AlSiO
6]. In highly substituted Ca-Fe-Mg pyroxenes, several substitutional schemes may be identified."[28]
"Substitution schemes 2 and 4 have not yet yielded named mineral species."[28]
"Augite could be considered to be a sub-calcic member of the diopside-hedenbergite series but, as defined by Morimoto et al. (1988) may have Fe>Mg or Mg>Fe without a change in name. Because of the way augite has been defined (Morimoto et al, 1988), there is the implication that in rare cases, a variety of anions may be dominant in various the various sites, including M2, M1, and T. These extreme compositions are rare in the large majority of augites. The clinopyroxenes with Wo
(5-20) are classed as pigeonite, another mineral that could be thought of as slightly alkalic clinoenstatite-clinoferrosilite."[28]
"However, there is a structural discontinuity between these pyroxenes at low-medium temperatures, and detailed chemical analysis and even structural analysis should be obtained in order to properly classify these unusual compositions. Similarly, extensive substitution of Na, for example, as in scheme 1 (Morimoto et al., 1988) could lead to Aegirine-augite or aegirine compositions depending on the other elements involved."[28]
Coesites[edit | edit source]


Coesite is a polymorph of silicon dioxide SiO
2 that is formed when very high pressure (2–3 gigapascals), and moderately high temperature (700 °C, 1,300 °F), are applied to quartz. Coesite was first synthesized by Loring Coes Jr., a chemist at the Norton Company, in 1953.[31][32]
In 1960, a natural occurrence of coesite was reported by Edward C. T. Chao,[33] in collaboration with Eugene Shoemaker, from the Barringer Crater, in Arizona, US, which was evidence that the crater must have been formed by an impact. After this report, the presence of coesite in unmetamorphosed rocks was taken as evidence of a meteorite impact event or of an atomic bomb explosion. It was not expected that coesite would survive in high pressure metamorphic rocks.
In metamorphic rocks, coesite was initially described in eclogite xenoliths from the mantle of the Earth that were carried up by ascending magmas; kimberlite is the most common host of such xenoliths.[34] In metamorphic rocks, coesite is now recognized as one of the best mineral indicators of metamorphism at very high pressures (UHP, or ultrahigh-pressure metamorphism).[35] Such UHP metamorphic rocks record subduction or continental collisions in which crustal rocks are carried to depths of 70 km (43 mi) or more. Coesite is formed at pressures above about 2.5 GPa (25 kbar) and temperature above about 700 °C. This corresponds to a depth of about 70 km in the Earth. It can be preserved as mineral inclusions in other phases because as it partially reverts to quartz, the quartz rim exerts pressure on the core of the grain, preserving the metastable grain as tectonic forces uplift and expose these rock at the surface. As a result, the grains have a characteristic texture of a polycrystalline quartz rim.
Coesite has been identified in UHP metamorphic rocks around the world, including the western Alps of Italy at Dora Maira,[35] the Erzgebirge of Germany,[36] the Lanterman Range of Antarctica,[37] in the Kokchetav Massif of Kazakhstan,[38] in the Western Gneiss region of Norway,[39] the Dabie-Shan Range in Eastern China,[40] the Himalayas of Eastern Pakistan,[41] and the Vermont Appalachian Mountains.[42][43]
Coesite is a tectosilicate with each silicon atom surrounded by four oxygen atoms in a tetrahedron. Each oxygen atom is then bonded to two Si atoms to form a framework. There are two crystallographically distinct Si atoms and five different oxygen positions in the unit cell. Although the unit cell is close to being hexagonal in shape ("a" and "c" are nearly equal and β nearly 120°), it is inherently monoclinic and cannot be hexagonal. The crystal structure of coesite is similar to that of feldspar and consists of four silicon dioxide tetrahedra arranged in Si
4O
8 and Si
8O
16 rings. The rings are further arranged into chains. This structure is metastable within the stability field of quartz: coesite will eventually decay back into quartz with a consequent volume increase, although the metamorphic reaction is very slow at the low temperatures of the Earth's surface. The crystal symmetry is monoclinic C2/c, No.15, Pearson symbol mS48.[44]
Enstatites[edit | edit source]
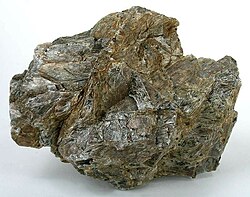
- Formula: Mg
2Si
2O
6.[45] - Crystal System: Orthorhombic.[45]
- Class (H-M): mmm (2/m 2/m 2/m) - Dipyramidal.[45]
- Space Group: Pbca.[45]
- Cell Parameters: a = 18.23 Å, b = 8.84 Å, c = 5.19 Å.[45]
- Z: 8.[45]
- Polymorph of: Akimotoite, Bridgmanite, Clinoenstatite.[45]
- Hardness: 5 - 6 on Mohs scale.[45]
- Tenacity: Brittle.[45]
- Common Impurities: Fe,Ca,Al,Co,Ni,Mn,Ti,Cr,Na,K.[45]
Enstatite and the other orthorhombic pyroxenes are distinguished from those of the monoclinic series by their optical characteristics, such as straight extinction, much weaker double refraction and stronger pleochroism.[46]
The "enstatite achondrite-like E-asteroids almost certainly derive from the melting and differentiation of highly reduced parent materials analogous to enstatite chondrites (e.g., Keil 1989)."[47]
Garnets[edit | edit source]
"Abundances of rare earth elements, Hf, Sc, Co, Cr and Th in garnet megacrysts and their volcanic hosts or matrices are used to estimate garnet/liquid partition coefficients for these elements."[48]
"The wide variation in garnet/liquid partition coefficients from kimberlites to rhyolites cannot be explained as an effect of temperature and we conclude that a major factor is the composition of the melt from which the garnet crystallized."[48]
"Garnet = Mineral Group of general formula: A3B2(SiO4)3, A = Ca, Mg, Fe2+, Mn2+; B = Al, Fe3+, Cr, V, Zr, Ti."[49]
The garnets make up two solid solution series: pyrope-almandine-spessartine (pyralspite), with the composition range [Mg,Fe,Mn]
3Al
2(SiO
4)
3; and uvarovite-grossular-andradite (ugrandite), with the composition range Ca
3[Cr,Al,Fe]
2(SiO
4)
3.
Def. a "hard transparent mineral that is often used as gemstones and abrasives"[50] is called a garnet.
"Back-scattered electron image of a typically zoned shock melt vein in NWA 8003 [shows] Cpx: clinopyroxene; Grt: garnet; Coe: coesite; Cpx: clinopyroxene; FSA: fine-grained silica aggregate.[10]
"Garnet occurs as fine dendritic or anhedral to euhedral grains in the zoned melt veins of NWA 8003 [...]."[10]
"Electron Probe Micro-Analyzer (EPMA) results [...] reveal that all garnets are super-silicic, containing 6–13 mol% majorite components [Mg
3(MgVISi)IVSi
3O
12]. The garnet grains within the eclogitic mineral assemblage contain slightly higher Si concentrations (3.09–3.13 atoms per formula unit, apfu; based on 12 analyses) than those in the central zone (3.06–3.09 apfu, 9 analyses), based on 12 oxygen atoms. In contrast, the Al concentrations in the former (1.70–1.80 apfu) are slightly lower than those in the latter (1.77–1.83 apfu)."[10]
Olivines[edit | edit source]


Def. "[a]ny of a group of olive green magnesium-iron" orthosilicates "that crystallize in the orthorhombic system"[51] is called an olivine.
At right is a visual close up of green sand which is actually olivine crystals that have been eroded from lava rocks. Some olivine crystals are still inside the lava rock.
Forsterite "(Mg2SiO4) is the magnesium rich end-member of the olivine solid solution series."[52]
"Forsterite is associated with igneous and metamorphic rocks and has also been found in meteorites. In 2005 it was also found in cometary dust returned by the Stardust probe.[53] In 2011 it was observed as tiny crystals in the dusty clouds of gas around a forming star.[54]"[52]
"Two polymorphs of forsterite are known: wadsleyite (also orthorhombic) and ringwoodite (isometric). Both are mainly known from meteorites."[52]
Orthopyroxenes[edit | edit source]

Pyroxenes that crystallize in the orthorhombic system are known as orthopyroxenes, e.g.:
- Enstatite, Mg
2Si
2O
6 - Bronzite, intermediate between enstatite and hypersthene
- Hypersthene, (Mg,Fe)SiO
3 - Eulite, intermediate between hypersthene and ferrosilite
- Ferrosilite, Fe
2Si
2O
6 - Donpeacorite, (MgMn)MgSi
2O
6 - Nchwaningite, Mn2+
2SiO
3(OH)
2⋅(H
2O)
Pigeonites[edit | edit source]

In the image on the right is a polarized light microscope image of part of a grain of orthopyroxene containing exsolution lamellae of augite from the Bushveld intrusion. The texture documents a multistage history: (1) crystallization of twinned pigeonite, followed by exsolution of augite; (2) breakdown of pigeonite to orthopyroxene plus augite; (3) exsolution of augite parallel to the former twin plane of pigeonite.
Formula: (Ca,Mg,Fe)(Mg,Fe)Si
2O
6. The calcium cation fraction can vary from 5% to 25%, with iron and magnesium making up the rest of the cations.
Pigeonite is a mineral in the clinopyroxene subgroup of the pyroxene group.
Pigeonite is found as phenocrysts in volcanic rocks on Earth and as crystals in meteorites from Mars and the Moon. In slowly cooled intrusive igneous rocks, pigeonite is rarely preserved. Slow cooling gives the calcium the necessary time to separate itself from the structure to form exsolution lamellae of calcic clinopyroxene[55], leaving no pigeonite present.[56] Textural evidence of its breakdown to orthopyroxene plus augite may be present, as shown in the accompanying microscopic image.
Pigeonite is named for its type locality on Lake Superior's shores at Pigeon Point, Minnesota, United States. It was first described in 1900.[57][58]
Quartzes[edit | edit source]

Red "thermoluminescence (RTL) emission from quartz, as a dosimeter for baked sediments and volcanic deposits, [from] older (i.e., >1 Ma), quartz-bearing known age volcanic deposits [can use as standards] independently-dated silicic volcanic deposits from New Zealand, ranging in age from 300 ka through to 1.6 Ma."[59]
"Back-scattered electron images of high-pressure minerals in NWA 8003 [show a] quartz grain adjacent to a melt vein has transformed to stishovite and silica glass."[10]
Stishovites[edit | edit source]

Stishovite is an extremely hard, dense tetragonal polymorph of silicon dioxide. It is very rare on the Earth's surface; however, it may be a predominant form of silicon dioxide in the Earth, especially in the lower mantle.[60]
Stishovite was named after Sergey M. Stishov, a Russian high-pressure physicist who first synthesized the mineral in 1961. It was discovered in Meteor Crater in 1962 by Edward C. T. Chao.[61]
Unlike other silica polymorphs, the crystal structure of stishovite resembles that of rutile TiO
2. The silicon in stishovite adopts an octahedral coordination geometry, being bound to six oxides. Similarly, the oxides are three-connected, unlike low-pressure forms of SiO2. In most silicates, silicon is tetrahedral, being bound to four oxides.[62] It was long considered the hardest known oxide (~30 GPa Vickers[63]); however, boron suboxide has been discovered[64] in 2002 to be much harder. At normal temperature and pressure, stishovite is metastable.
Stishovite can be separated from quartz by applying hydrogen fluoride (HF); unlike quartz, stishovite will not react.[61]
Large natural crystals of stishovite are extremely rare and are usually found as clasts of 1 to 2 mm in length. When found, they can be difficult to distinguish from regular quartz without laboratory analysis. It has a vitreous luster, is transparent (or translucent), and is extremely hard. Stishovite usually sits as small rounded gravels in a matrix of other minerals.
Until recently, the only known occurrences of stishovite in nature formed at the very high shock pressures (>100 kbar, or 10 GPa) and temperatures (> 1200 °C) present during hypervelocity meteorite impact into quartz-bearing rock. Minute amounts of stishovite have been found within diamonds,[65] and post-stishovite phases were identified within ultra-high-pressure mantle rocks.[66] Stishovite may also be synthesized by duplicating these conditions in the laboratory, either isostatically or through shock (see shocked quartz).[67] At 4.287 g/cm3, it is the second densest polymorph of silica, after seifertite. It has tetragonal crystal symmetry, P42/mnm, No. 136, Pearson symbol tP6.[68]
Def. a polymorph of α-quartz formed by pressures > 100 kbar or 10 GPa and temperatures > 1200 °C is called stishovite.[69]
Stishovite may be formed by an instantaneous over pressure such as by an impact or nuclear explosion type event.[70]
Minute amounts of stishovite has been found within diamonds.[65]
Tissintites[edit | edit source]

"The high-pressure minerals tissintite [Tst], vacancy-rich clinopyroxene [Cpx], and super-silicic garnet are observed in the shocked eucrite Northwest Africa (NWA) 8003 in addition to coesite and stishovite."[10]
In the image on the right are "Cpx: clinopyroxene; Opx: orthopyroxene; Aug: augite; Tro: troilite; Msk: maskelynite; Tst: tissintite; FSA: fine-grained silica aggregate."[10] "It consists dominantly of clinopyroxene with minor mineral fragments and fine-grained silica aggregates."[10]
"Based on six oxygen atoms, the tissintite in NWA 8003 has an average chemical formula (Ca
0.64Na
0.09□0.27)(Al
0.99Fe
0.01)(Si
1.62Al
0.38)O
6 with high vacancy concentrations [□0.27] on the M2 site."[10]
Troilites[edit | edit source]

This is a macro photograph of an etched surface of the Mundrabilla meteorite, a small piece of the approximately 3.9 billion-year-old meteorite that was first discovered in Western Australia in 1911. Two more giant chunks, together weighing about 17 tons, were found in 1966. Researchers can learn much from this natural crystal growth experiment since it has spent several hundred million years cooling, and would be impossible to emulate in a lab. This single slice, taken from a 6 ton piece recovered in 1966, measures only 2 square inches. The macro photograph shows a metallic iron-nickel alloy phase of kamcite (38% Ni) and taenite (6% Ni) at bottom right, bottom left, and top left. The darker material is an iron sulfide (FeS or troilite) with parallel precipitates of duabreelite (iron chromium sulfide (FeCr
2S
4).
See Also[edit | edit source]
References[edit | edit source]
- ↑ Jutzi, M.; E. Asphaug; P. Gillet; J.-A. Barrat; W. Benz (14 February 2013). "The structure of the asteroid 4 Vesta as revealed by models of planet-scale". Nature 494 (7436): 207–210. doi:10.1038/nature11892. PMID 23407535.
- ↑ Cook, Jia-Rui. "Dawn Reality-Checks Telescope Studies of Asteroids". Retrieved 30 April 2014.
{{cite web}}
:|archive-date=
requires|archive-url=
(help) - ↑ McSween, H. Y.; R. P. Binzel; M. C. De Sanctis; E. Ammannito; T. H. Prettyman; A. W. Beck; V. Reddy; L. Le Corre et al. (27 November 2013). "Dawn; the Vesta-HED connection; and the geologic context for eucrite, diogenites, and howardites". Meteoritics & Planetary Science 48 (11): 2090–21–4. doi:10.1111/maps.12108.
- ↑ 4.0 4.1 Kelley, M. S. (2003). "Quantified mineralogical evidence for a common origin of 1929 Kollaa with 4 Vesta and the HED meteorites". Icarus 165 (1): 215–218. doi:10.1016/S0019-1035(03)00149-0. https://zenodo.org/record/1259601.
- ↑ 5.0 5.1 "Vesta". NASA/JPL. 12 July 2011. Retrieved 30 July 2011.
{{cite web}}
:|archive-date=
requires|archive-url=
(help) - ↑ Wikipedia:Vesta (mythology)
- ↑ F Roig, D Nesvorný, R Gil-Hutton, D Lazzaro (March 2008). "V-type asteroids in the middle main belt". Icarus 194 (1): 125–36. doi:10.1016/j.icarus.2007.10.004. http://arxiv.org/pdf/0707.1012. Retrieved 2016-10-10.
- ↑ 8.0 8.1 8.2 8.3 Deuar (12 February 2006). Vesta family. San Francisco, California: Wikimedia Foundation, Inc. https://en.wikipedia.org/wiki/Vesta_family. Retrieved 2018-05-04.
- ↑ Vincenzo Zappalà, Philippe Bendjoya, Alberto Cellino, Paolo Farinella and Claude Froeschlé (August 1995). "Asteroid Families: Search of a 12,487-Asteroid Sample Using Two Different Clustering Techniques". Icarus 116 (2): 291-314. http://www.sciencedirect.com/science?_ob=ArticleURL&_udi=B6WGF-45NJHPF-3C&_coverDate=08%2F31%2F1995&_alid=267428521&_rdoc=1&_fmt=&_orig=search&_qd=1&_cdi=6821&_sort=d&view=c&_acct=C000056973&_version=1&_urlVersion=0&_userid=2337731&md5=6a0ee5be29f8c0489e922cb06868ae45. Retrieved 2018-5-04.
- ↑ 10.00 10.01 10.02 10.03 10.04 10.05 10.06 10.07 10.08 10.09 Run-Lian Pang, Ai-Cheng Zhang, Shu-Zhou Wang, Ru-Cheng Wang & Hisayoshi Yurimoto (16 May 2016). "High-pressure minerals in eucrite suggest a small source crater on Vesta". Nature Scientific Reports 6: 26063. doi:10.1038/srep26063.
- ↑ Thomas H. Prettyman, David W. Mittlefehidt, Naoyuki Yamashita, David J. Lawrence, Andrew W. Beck, William C. Feldman, Timothy J. McCoy, Harry Y. McSween, Michael J. Toplis, Timothy N. Titus, Pasquale Tricarico, Robert C. Reedy, John S. Hendricks, Olivier Forni, Lucille Le Corre, Jian-Yang Li, Hugau Mizzon, Vishnu Reddy, Carol A. Raymond, Christopher T. Russell (October 2012). "Elemental Mapping by Dawn Reveals Exogenic H in Vesta's Regolith". Sience 338 (6104): 242-6. doi:10.1126/science.1225354.
- ↑ diogenite. San Francisco, California: Wikimedia Foundation, Inc. December 5, 2008. https://en.wiktionary.org/wiki/diogenite. Retrieved 2013-12-20.
- ↑ Beck, A. W.; McSween, H. Y. (2010). "Diogenites as polymict breccias composed of orthopyroxenite and harzburgite". Meteoritics and Planetary Science 45 (5): 850–872. doi:10.1111/j.1945-5100.2010.01061.x.
- ↑ K Keil (2002). Geological history of asteroid 4 Vesta: The “smallest terrestrial planet”, In: Asteroids III. https://www.researchgate.net/profile/K_Keil/publication/259276198_Geological_History_of_Asteroid_4_Vesta_The_Smallest_Terrestrial_Planet/links/54d4f1380cf2970e4e63f1ba.pdf. Retrieved 2016-10-10.
- ↑ eucrite. San Francisco, California: Wikimedia Foundation, Inc. June 20, 2013. https://en.wiktionary.org/wiki/eucrite. Retrieved 2013-12-20.
- ↑ B. Mason: Meteorites. John Wiley, New York 1962.
- ↑ howardite. San Francisco, California: Wikimedia Foundation, Inc. June 20, 2013. https://en.wiktionary.org/wiki/howardite. Retrieved 2013-12-20.
- ↑ 18.0 18.1 18.2 18.3 18.4 18.5 Michael J. Gaffey (May 1997). "Surface Lithologic Heterogeneity of Asteroid 4 Vesta". Icarus 127 (1): 130-157. doi:10.1006/icar.1997.5680. https://www.sciencedirect.com/science/article/abs/pii/S0019103597956803. Retrieved 19 June 2019.
- ↑ M. Chen; A. El Goresy (1999). The Nature of "Maskelynite" in Shocked Meteorites: Not Diaplectic Glass but a Glass Quenched from Shock-Induced Dense Melt at High-Pressures, In: Proceedings of the 62nd Annual Meteoritical Society Meeting. Johannesburg. http://www.lpi.usra.edu/meetings/metsoc99/pdf/5047.pdf.
- ↑ Daniel J. Milton; Paul S. de Carli (1963). "Maskelynite: Formation by Explosive Shock". Science 140 (3567): 670–671. doi:10.1126/science.140.3567.670. PMID 17737107.
- ↑ 21.0 21.1 21.2 21.3 21.4 T. E. Bunch, Alvin J. Cohen and M. R. Dence (January-February 1967). "Natural Terrestrial Maskelynite". The American Mineralogist 52: 244-253. http://www.minsocam.org/ammin/AM52/AM52_244.pdf. Retrieved 11 December 2021.
- ↑ Milam, K. A. (2010). "Distribution and variation of plagioclase compositions on Mars". Journal of Geophysical Research: Planets 115 (E9). doi:10.1029/2009JE003495.
- ↑ [1] Anorthite}}
- ↑ Deer, W. A., R. A. Howie and J. Zussman (1966) An Introduction to the Rock Forming Minerals, Longman, ISBN 0-582-44210-9
- ↑ "Significant Lunar Minerals" (PDF). In Situ Resource Utilization (ISRU). NASA. Retrieved 23 August 2018.
{{cite web}}
:|archive-date=
requires|archive-url=
(help) - ↑ Handbook of Mineralogy
- ↑ Webmineral
- ↑ 28.00 28.01 28.02 28.03 28.04 28.05 28.06 28.07 28.08 28.09 28.10 28.11 28.12 https://www.mindat.org/min-419.html Augite
- ↑ Handbook of Mineralogy
- ↑ 30.0 30.1 https://www.mindat.org/min-7630.html Clinopyroxene Subgroup
- ↑ The word "coesite" is pronounced as "Coze-ite" after chemist Loring Coes Jr. Coes, L. Jr. (31 July 1953). "A New Dense Crystalline Silica". Science 118 (3057): 131–132. doi:10.1126/science.118.3057.131. PMID 17835139.
- ↑ Robert M. Hazen (22 July 1999). The Diamond Makers. Cambridge University Press. pp. 91–. ISBN 978-0-521-65474-6. https://books.google.com/books?id=fNJQok6N9_MC&pg=PA91. Retrieved 6 June 2012.
- ↑ Chao, E. C. T.; Shoemaker, E. M.; Madsen, B. M. (1960). "First Natural Occurrence of Coesite". Science 132 (3421): 220–2. doi:10.1126/science.132.3421.220. PMID 17748937.
- ↑ Smyth, Joseph R.; Hatton, C.J. (1977). "A coesite-sanidine grospydite from the Roberts Victor kimberlite". Earth and Planetary Science Letters 34 (2): 284. doi:10.1016/0012-821X(77)90012-7.
- ↑ 35.0 35.1 Chopin, Christian (1984). "Coesite and pure pyrope in high-grade blueschists of the Western Alps: a first record and some consequences". Contributions to Mineralogy and Petrology 86 (2): 107–118. doi:10.1007/BF00381838.
- ↑ Massonne, H.-J. (2001). "First find of coesite in the ultrahigh-pressure metamorphic area of the central Erzgebirge, Germany". European Journal of Mineralogy 13 (3): 565–570. doi:10.1127/0935-1221/2001/0013-0565.
- ↑ Ghiribelli, B.; Frezzotti, M.L.; Palmeri, R. (2002). "Coesite in eclogites of the Lanterman Range (Antarctica): Evidence from textural and Raman studies". European Journal of Mineralogy 14 (2): 355–360. doi:10.1127/0935-1221/2002/0014-0355.
- ↑ Korsakov, A.V.; Shatskiy, V. S.; Sobolev N.V. (1998). "Первая находка коэсита в эклогитах Кокчетавского массива (First occurrence of coesite in eclogites from the Kokchetav Massif)". Doklady Earth Sciences 359: 77–81.
- ↑ Smith, D.C. (1984). "Coesite in clinopyroxene in the Caledonides and its implications for geodynamics". Nature 310 (5979): 641–644. doi:10.1038/310641a0.
- ↑ Schertl, H.-P.; Okay, A.I. (1994). "A coesite inclusion in dolomite in Dabie Shan, China: petrological and rheological significance". Eur. J. Mineral. 6 (6): 995–1000. doi:10.1127/ejm/6/6/0995. http://eurjmin.geoscienceworld.org/content/6/6/995.short.
- ↑ O'Brien, P.J., N. Zotov, R. Law, M.A. Khan and M.Q. Jan (2001). "Coesite in Himalayan eclogite and implications for models of India-Asia collision". Geology 29 (5): 435–438. doi:10.1130/0091-7613(2001)029<0435:CIHEAI>2.0.CO;2.
- ↑ Joseph Gonzalez, Suzanne Baldwin, Jay B Thomas, William O Nachlas, Paul G Fitzgerald (2019). "First Discovery of Coesite in the Appalachians: Characterization of Prograde Metamorphism in a Taconic Metapelite". AGU Fall Meeting 2019: V51B–03. https://agu.confex.com/agu/fm19/meetingapp.cgi/Paper/552005.
- ↑ Joseph Gonzalez, Suzanne Baldwin, Jay B Thomas, William O Nachlas, Paul G Fitzgerald (2020). "Evidence for ultrahigh-pressure metamorphism discovered in the Appalachian orogen". Geology. doi:10.1130/G47507.1.
- ↑ Levien L.; Prewitt C.T. (1981). "High-pressure crystal structure and compressibility of coesite". American Mineralogist 66: 324–333. https://web.archive.org/web/20160604062422/http://www.minsocam.org/ammin/AM66/AM66_324.pdf. Retrieved 2009-12-15.
- ↑ 45.00 45.01 45.02 45.03 45.04 45.05 45.06 45.07 45.08 45.09 https://www.mindat.org/min-1384.html
- ↑ Leonard James (1911). "Enstatite". In Chisholm, Hugh (ed.). Encyclopædia Britannica. Vol. 9 (11th ed.). Cambridge University Press. p. 654.
- ↑ Michael J. Gaffey; Jeffrey F. Bell; R. Hamilton Brown; Thomas H. Burbine; Jennifer L. Piatek; Kevin L. Reed; Damon A. Chaky (December 1993). "Mineralogical variations within the S-type asteroid class". Icarus 106 (2): 573-602. http://www.mtholyoke.edu/~tburbine/gaffey.icarus.1993.pdf. Retrieved 2015-09-03.
- ↑ 48.0 48.1 Anthony J. Irving; Frederick A. Frey (June 1978). "Distribution of trace elements between garnet megacrysts and host volcanic liquids of kimberlitic to rhyolitic composition". Geochimica et Cosmochimica Acta 42 (6): 771-87. doi:10.1016/0016-7037(78)90092-3. http://www.sciencedirect.com/science/article/pii/0016703778900923. Retrieved 2016-02-12.
- ↑ Willard Lincoln Roberts; George Robert Rapp Jr.; Julius Weber (1974). Encyclopedia of Minerals. New York, New York, USA: Van Nostrand Reinhold Company. pp. 693. ISBN 0-442-26820-3.
- ↑ "garnet". San Francisco, California: Wikimedia Foundation, Inc. 21 January 2017. Retrieved 2017-02-21.
- ↑ "olivine". San Francisco, California: Wikimedia Foundation, Inc. August 30, 2012. Retrieved 2012-10-23.
- ↑ 52.0 52.1 52.2 "Forsterite". San Francisco, California: Wikimedia Foundation, Inc. October 24, 2012. Retrieved 2013-01-22.
- ↑ Ds. Lauretta; L.P. Keller; S. Messenger (2005). "Supernova olivine from cometary dust". Science 309 (5735): 737–741. doi:10.1126/science.1109602. PMID 15994379.
- ↑ Spitzer sees crystal 'rain' in outer clouds of infant star, Whitney Clavin and Trent Perrotto, Physorg.com, May 27, 2011 . Accessed May 2011
- ↑ [2]
- ↑ Nesse, William (2012). Introduction to Mineralogy (Second ed.). Oxford University Press. p. 300.
- ↑ http://www.mindat.org/min-3210.html Mindat.org
- ↑ Winchell, Alexander N. (1900). "Mineralogical and petrographic study of the gabbroid rocks of Minnesota, and more particularly, of the plagioclasytes". The American Geologist 26 (4): 197–245.
- ↑ M. Fattahi; S. Stokes (December 2000). "Extending the time range of luminescence dating using red TL (RTL) from volcanic quartz". Radiation Measurements 32 (5-6): 479-85. doi:10.1016/S1350-4487(00)00105-0. http://www.sciencedirect.com/science/article/pii/S1350448700001050. Retrieved 2016-02-12.
- ↑ Dmitry L. Lakshtanov "The post-stishovite phase transition in hydrous alumina-bearing SiO
2 in the lower mantle of the earth" PNAS 2007 104 (34) 13588-13590; doi:10.1073/pnas.0706113104. - ↑ 61.0 61.1 Fleischer, Michael (1962). "New mineral names". American Mineralogist (Mineralogical Society of America) 47 (2): 172–174. http://rruff.info/uploads/AM47_805.pdf.
- ↑ Ross, Nancy L. (1990). "High pressure crystal chemistry of stishovite". American Mineralogist (Mineralogical Society of America) 75 (7): 739–747. http://www.minsocam.org/ammin/AM75/AM75_739.pdf.
- ↑ Luo, Sheng-Nian; Swadener, J. G.; Ma, Chi; Tschauner, Oliver (2007). "Examining crystallographic orientation dependence of hardness of silica stishovite". Physica B: Condensed Matter 399 (2): 138. doi:10.1016/j.physb.2007.06.011. http://www.its.caltech.edu/~chima/publications/2007_PBCM_stishovite.pdf. and references therein
- ↑ He, Duanwei; Zhao, Yusheng; Daemen, L.; Qian, J.; Shen, T. D.; Zerda, T. W. (2002). "Boron suboxide: As hard as cubic boron nitride". Applied Physics Letters 81 (4): 643. doi:10.1063/1.1494860.
- ↑ 65.0 65.1 R Wirth; C. Vollmer; F. Brenker; S. Matsyuk; F. Kaminsky (2007). "Inclusions of nanocrystalline hydrous aluminium silicate "Phase Egg" in superdeep diamonds from Juina (Mato Grosso State, Brazil)". Earth and Planetary Science Letters 259 (3–4): 384. doi:10.1016/j.epsl.2007.04.041.
- ↑ Liu, L.; Zhang, J.; Greenii, H.; Jin, Z.; Bozhilov, K. (2007). "Evidence of former stishovite in metamorphosed sediments, implying subduction to >350 km". Earth and Planetary Science Letters 263 (3–4): 180. doi:10.1016/j.epsl.2007.08.010. https://web.archive.org/web/20100717031853/http://micron.ucr.edu/Public/KNB-papers/Liu-et-al-2007.pdf.
- ↑ J. M. Léger, J. Haines, M. Schmidt, J. P. Petitet, A. S. Pereira & J. A. H. da Jornada (1996). "Discovery of hardest known oxide". Nature 383 (6599): 401. doi:10.1038/383401a0.
- ↑ Smyth J. R.; Swope R. J.; Pawley A. R. (1995). "H in rutile-type compounds: II. Crystal chemistry of Al substitution in H-bearing stishovite". American Mineralogist 80 (5–6): 454–456. doi:10.2138/am-1995-5-605. http://rruff.geo.arizona.edu/doclib/am/vol80/AM80_454.pdf.
- ↑ "Stishovite". San Francisco, California: Wikimedia Foundation, Inc. October 24, 2012. Retrieved 2012-10-23.
- ↑ "Shocked quartz". San Francisco, California: Wikimedia Foundation, Inc. May 14, 2012. Retrieved 2012-10-23.