Geochronology/Cenozoic

Cenozoic geochronology is the science of applying dates in the past to apparently Cenozoic rocks.
The cliffs at Stevns, in the image at the top of this page, have the highest iridium occurrence in the Alvarez analysis.
Notations[edit | edit source]
Let
- ALMA represent the Asian Land Mammal Age,
- b2k represent before AD 2000,
- BP represent before present, as the chart is for 2008, this may require an added -8 for b2k,
- ELMMZ represent the European Land Mammal Mega Zone,
- FAD represent first appearance datum,
- GICC05 represent Greenland Ice Core Chronology 2005,
- GRIP represent Greenland Ice Core Project,
- GSSP represent Global Stratotype Section and Point,
- ICS represent the International Commission on Stratigraphy,
- IUGS represent the International Union of Geological Sciences,
- LAD represent last appearance datum,
- Ma represent Megaannum, or million years ago, or -106 b2k,
- NALMA represent the North American Land Mammal Age,
- NGRIP represent North Greenland Ice Core Project, and
- SALMA represent South American Land Mammal Age.
"The term b2 k [b2k] refers to the ice-core zero age of AD 2000; note that this is 50 years different from the zero yr for radiocarbon, which is AD 1950 [...]."[1]
Cenozoic time frames[edit | edit source]
Name (English)[2] | base/start (Ma)[3] | top/end (Ma)[3] | status | subdivision of | usage | named after | author, year |
---|---|---|---|---|---|---|---|
Aftonian | 0.6 | 0.48 | age | Pleistocene | North America | ||
Agenian | 23 | 20.4 | ELMMZ | Miocene | Europe | Agen (France) | |
Aldingan | 36 | 30 | age | Eocene | Australia | ||
Allerød | 13,350 BP | 12,700 BP | chronozone | Weichselian | Northern Europe | Allerød (Denmark) | |
Altonian | 19.0 | 15.9 | age | Miocene | New Zealand | Alton | |
Amstelian | 2.588 | 2.40 | super-age | Pleistocene | Netherlands | river Amstel | Harmer, 1896 |
Anglian | 0.465 | 0.418 | age | Pleistocene | Great Britain | East Anglia | |
Antian | ~2.12 | ~2.0 | age | Pleistocene | Great Britain | River Ant (England) | |
Antwerpian | 19 | 18.4 | age | Miocene | Belgium (obsolete) | Antwerp | Gogels, 1879 |
Aquatraversian | 2.588 | 2.4 | age | Pleistocene | Italy | ||
Aquitanian | 23.03 | 20.43 | age | Miocene | ICS | Aquitaine | |
Arikareean | 30.6 | 20.8 | super-age | Oligocene-Miocene | North America | ||
Arnold | 43.0 | 34.3 | epoch | Paleogene | New Zealand | Arnold River | |
Arshantan | 52.1 | 46.2 | ALMA | Eocene | Asia | ||
Astaracian | 15 | 11.1 | ELMMZ | Miocene | Europe | The Astarac (France) | |
Atlantic | 5,660 BP | 9,220 BP | chronozone | Holocene | Northern Europe | the Atlantic Ocean | Blytt, 1876 |
Avernian | 29.2 | 23.03 | ELMMZ | Oligocene | Europe | ||
Awamoan | 20.0 | 17.5 | age | Miocene | New Zealand | ||
Badenian | 16.3 | 12.8 | age | Miocene | Paratethys | Baden (Austria) | Papp & Cicha, 1968 |
Bairnsdalian | 15.0 | 10.5 | age | Miocene | Australia | ||
Balcombian | 15.5 | 15.0 | age | Miocene | Australia | ||
Barstovian | 16.3 | 13.6 | age | Miocene | North America | ||
Bartonian | 37.2 ± 0.1 | 40.4 ± 0.2 | age | Eocene | ICS | Barton-on-Sea (South England) | Mayer-Eymar, 1857 |
Batesfordian | 16.5 | 15.5 | age | Miocene | Australia | ||
Bavel Interglacial | 1.03 | 0.96 | age | Pleistocene | Netherlands | Bavel | |
Bavelian | 1.03 | 0.85 | super-age | Pleistocene | Netherlands | Bavel | |
Baventian | ~2.0 | ~1.87 | age | Pleistocene | Great Britain | Easton Bavents (England) | West, 1961 |
Beestonian | 1.77 | ~0.8 | age | Pleistocene | Great Britain | Beeston, Norfolk (England) | |
Belvédère Interglacial | 0.338 | 0.324 | age | Pleistocene | Netherlands | quarry "Belvédère" (Maastricht) | |
Biber Glacial | ~2.5 | 2.35 | age | Pleistocene | Alps | river Biber (Germany) | |
Biber-Donau | age | Pleistocene | Alps | ||||
Blancan | 4.9 | 1.8 | age | Pliocene-Pleistocene | North America | ||
Bolderian | <23.03 | ≥19 | age | Miocene | Belgium | Bolderberg | Dumont, 1850 |
Bølling | 13,730 BP | 13,480 BP | chronozone | Weichselian | Northern Europe | Bølling Sø (Denmark) | |
Boreal | 10,640 BP | 9,220 BP | chronozone | Holocene | Northern Europe | boreal zone in ecology | Blytt, 1876 |
Bortonian | 43.0 | 37.0 | age | Eocene | New Zealand | Bortons | |
Bramertonian | ~2.12 | ~2.0 | age | Pleistocene | Great Britain | Bramerton Pits (England) | Funnell, Norton, West and Mayhew, 1979 |
Bridgerian | 50.3 | 46.2 | age | Eocene | North America | ||
Brüggenian | 2.588 | 2.4 | chronozone | Pleistocene | Northwest Europe | ||
Brunssumian | 5.3 | 3.6 | chronozone | Pliocene | Northwest Europe | Brunssum (The Netherlands) | |
Bulitian | 55.8 | 53 | age | Eocene | California | ||
Bumbanian | 55.7 | 52.1 | ALMA | Eocene | Asia | ||
Burdigalian | 20.43 | 15.97 | age | Miocene | ICS | Latin: Burdigala = Bordeaux (France) | Depéret, 1892 |
Calabrian | 1.806 | 0.781 | age | Pleistocene | Southern Europe | Calabria | |
Casamajoran | 54 | 48 | age | Eocene | South America | ||
Cassian | 1.1 | 0.8 | age | Pleistocene | Italy | ||
Castlecliffian | 1.63 | 0.34 | age | Pleistocene | New Zealand | Castlecliff | |
Cenozoic | 65.5 ± 0.3 | present | era | Phanerozoic | ICS | new life | Phillips, 1847 |
Cernaysian | 55.9 | 55.0 | ELMMZ | Paleocene | Europe | ||
Chadronian | 38.0 | 33.9 | age | Eocene | North America | ||
Chapadmalalan | 4.0 | 3.0 | age | Pliocene | South America | ||
Chasicoan | 10.0 | 9.0 | age | Miocene | South America | ||
Chattian | 28.4 ± 0.1 | 23.03 | age | Oligocene | ICS | Chatti (ancient Germanic tribe) | Fuchs, 1894 |
Cheltenhamian | 5.0 | 4.3 | age | Pliocene | Australia | ||
Clarendonian | 13.6 | 10.3 | age | Miocene | North America | ||
Clarkforkian | 56.8 | 55.4 | age | Paleocene-Eocene | North America | ||
Clifdenian | 15.9 | 15.1 | age | Miocene | New Zealand | Clifden | |
Colhuehuapian | 21.0 | 17.5 | age | Miocene | South America | ||
Colloncurian | 15.5 | 12.0 | age | Miocene | South America | ||
Cromerian | 0.85 | 0.465 | super-age/age | Pleistocene | Netherlands, Great Britain | Cromer (England) | |
Dacian | 5.332 ± 0.005 | 3.600 ± 0.005 | age | Pliocene | Paratethys | Dacia (Roman province) | |
Danian | 65.5 ± 0.3 | 61.7 ± 0.2 | age | Paleocene | ICS | Denmark | Desor, 1847 |
Dannevirke | 65.0 | 43.0 | epoch | Paleogene | New Zealand | Dannevirke | |
Delmontian | 7.5 | 2.9 | age | Pliocene-Miocene | California | ||
Deseadan | 29.0 | 21.0 | age | Oligocene-Miocene | South America | ||
Deurnian | age | Miocene | Belgium (obsolete) | Deurne | de Heinzelin (1955) | ||
Devensian | 0.116 | 0.0115 | age | Pleistocene | Great Britain | Devenses, Celtic tribe by the Deva (England and Wales) | |
Divisaderan | 42 | 36 | age | Eocene | South America | ||
Donau Glacial | 1.7 | 1.35 | age | Pleistocene | Alps | river Danube | |
Donau-Günz | >2.35 | age | Pleistocene | Alps | |||
Drenthian | 0.238 | 0.17 | chronozone | Pleistocene | Northwest Europe | Drenthe | |
Duchesnean | 42.0 | 38.0 | age | Eocene | North America | ||
Duntroonian | 27.3 | 25.2 | age | Oligocene | New Zealand | Duntroon | |
Early Pleistocene | 1.6 | 0.73 | age | Pleistocene | |||
Eburonian | 1.80 | 1.45 | super-age | Pleistocene | Netherlands | Eburones, Germanic tribe | |
Eemian | 0.130 | 0.116 | age | Pleistocene | Northern Europe | river Eem (Netherlands) | Harting, 1875 |
Egerian | 25.8 | 20.3 | age | Oligocene-Miocene | Paratethys | Eger (Hungary) | Báldi & Seneš, 1968 |
Eggenburgian | 20.8 | 18.3 | age | Miocene | Paratethys | Eggenburg (Austria) | Steininger & Seneš, 1968 |
Elsterian | 0.465 | 0.418 | age | Pleistocene | Northern Europe | river Weißen Elster (Germany) | |
Emilian | 1.5 | 0.781 | sub-age | Pleistocene | Italy | ||
Ensenadan | 1.2 | 0.8 | SALMA | Pleistocene | South America | ||
Eocene | 55.8 ± 0.2 | 33.9 ± 0.1 | epoch | Paleogene | ICS | earliest recent | Lyell, 1847 |
Ergilian | 35.1 | 33.8 | ALMA | Oligocene-Eocene | Asia | ||
Flaminian | 0.5 | 0.3 | age | Pleistocene | Italy | ||
Flandrian | 0.01 | present | age | Holocene | Western Europe (obsolete) | Flanders | Rutot & Van den Broeck, 1885 |
Friasian | 16.3 | 15.5 | age | Miocene | South America | ||
Fujian | 11.1 | 9.5 | age | Miocene | Japan | ||
Gashatan | 56.9 | 55.7 | ALMA | Paleocene-Eocene | Asia | ||
Geiseltalian | 48.5 | 42.7 | ELMMZ | Eocene | Europe | ||
Gelasian | 2.588 | 1.806 | age | Pleistocene | ICS | Gela (Italy) | Rio et al., 1998 |
Geringian | 30.8 | 26.3 | age | Oligocene | North America | ||
Gramian | 10.3 | 8.5 | age | Miocene | Northern Germany | ||
Grauvian | 50.8 | 48.5 | ELMMZ | Eocene | Europe | ||
Greenlandian | 11.7 | 8.2 | Holocene | Greenland | |||
Günz Glacial | 2.35 | age | Pleistocene | Alps | river Günz (Germany) | ||
Günz-Mindel | age | Pleistocene | Alps | ||||
Hallian | ~10.000 | 0 | age | Holocene | California | ||
Haranoyan | 18.2 | 15.97 | age | Miocene | Japan | ||
Harrisonian | 24.8 | 20.6 | age | Oligocene-Miocene | North America | ||
Hautawan | 3.1 | 2.2 | sub-age | Pliocene-Pleistocene | New Zealand | ||
Haweran | 0.34 | present | age | Holocene | New Zealand | ||
Headonian | 37.2 | 33.8 | ELMMZ | Oligocene-Eocene | Europe | ||
Hemingfordian | 20.6 | 16.3 | age | Miocene | North America | ||
Hemmoorian | age | Miocene | Northern Germany | ||||
Hemphillian | 10.3 | 4.9 | age | Miocene-Pliocene | North America | ||
Heretaungan | 49.5 | 46.2 | age | Eocene | New Zealand | ||
Holocene | 11,800 BP | present | epoch | Quaternary | ICS | Greek: totally new | Gervais, 1867 |
Holsteinian | 0.418 | 0.386 | age | Pleistocene | Northern Europe | Holstein (Germany) | |
Houldjinian | 37.2 | 33.9 | ALMA | Asia | |||
Houthalenian | <21 | >16 | age | Miocene | Belgium (obsolete) | Houthalen | Hirsch, 1952 |
Hoxnian | 0.418 | 0.386 | age | Pleistocene | Great Britain | Hoxne (Suffolk) | West & Donner, 1956 |
Hsandgolian | 33.8 | 24.0 | ALMA | Oligocene | Asia | ||
Huayquerian | 9.0 | 6.8 | age | Miocene | South America | ||
Hutchinsonian | 21 | 20 | age | Miocene | New Zealand | ||
Icenian | 2.4 | ~2 | age | Pleistocene | Netherlands, England (obsolete) | Iceni, ancient tribe (England) | Pannekoek, 1956 |
Ilfordian | age | Pleistocene | British Isles | Ilford (England) | |||
Illinoian | 0.17 | 0.125 | age | Pleistocene | North America | ||
Ionian | 0.781 | 0.126 | age | Pleistocene | Southern Europe | Ionian Sea (between Greece and Italy) | |
Ipswichian | 0.130 | 0.116 | age | Pleistocene | Great Britain | Ipswich (England) | West, 1957 |
Irdinmanhan | 46.2 | 40.4 | ALMA | Eocene | Asia | ||
Irvingtonian | 1.8 | NALMA | Pleistocene | North America | Irvington | ||
Itaboraian | 59 | 57 | age | Paleocene | South America | ||
Jacksonian | [4] | age | Eocene | southern US | |||
Janjukian | 30.0 | 27.5 | age | Oligocene | Australia | ||
Johannian | 48 | 35 | age | Eocene | Australia | ||
Kaburan | 13.5 | 11.1 | age | Miocene | Japan | ||
Kaiatan | 37.0 | 36.0 | age | Eocene | New Zealand | ||
Kalimnan | 4.3 | 3.4 | age | Pliocene | Australia | ||
Kansan | 0.48 | 0.26 | age | Pleistocene | North America | ||
Kapitean | 6.5 | 5.0 | age | Miocene | New Zealand | ||
Karpatian | 17.0 | 16.0 | age | Miocene | Paratethys | the Carpathian Mountains | Cicha et al., 1967 |
Kasterlian | ~4.7 | ~3.6 | age | Pliocene | Belgium (obsolete) | Kasterlee | Dumont, 1882 |
Kattendijkian | ~5 | ~3.6 | age | Pliocene | Belgium (obsolete) | Kattendijke | Glibert & de Heinzelin, 1957 |
Kechienjian | 1.9 | 1.5 | age | Pleistocene | Japan | ||
Kiscellian | 25.8 | age | Oligocene | Paratethys | |||
Kryzhanovan | 1.9 | 1.2 | age | Pleistocene | Eastern Europe | ||
Landenian | <60 | >55 | age | Paleocene | Western Europe (obsolete) | Landen (Belgium) | Dumont, 1839 |
Landon | 34.3 | 21.7 | epoch | Paleogene-Neogene | New Zealand | ||
Langenfeldian | age | Miocene | Northern Germany | ||||
Langhian | 15.97 | 13.65 | age | Miocene | ICS | Serravalle Langhe (Italy) | Pareto, 1864 |
Latdorfian | age | Oligocene | Germany | ||||
Late Pleistocene | age | Pleistocene | |||||
Laventan | 13.8 | 12.0 | age | Miocene | South America | ||
Likhvinian | 0.3 | 0.18 | age | Pleistocene | Eastern Europe | ||
Lillburnian | 15.1 | 12.7 | age | Miocene | New Zealand | ||
Lishihhuangtuan | 1.2 | 0.1 | age | Pleistocene | China | ||
Longfordian | 27.5 | 16.5 | age | Oligocene-Miocene | Australia | ||
Ludhamian | ~2.52 | ~2.25 | age | Pleistocene | Great Britain | Ludham (England) | |
Ludian | age | Eocene | western Europe | de Lapparent, 1893 | |||
Luisian | 15.5 | 13.5 | age | Miocene | California | ||
Lujanian | 0.8 | 0.3 | age | Pleistocene | South America | ||
Lutetian | 48.6 ± 0.2 | 40.4 ± 0.2 | age | Eocene | ICS | Latin: Lutetia=Paris (France) | de Lapparent, 1883 |
Malanghuangtuan | 0.1 | 0.01 | age | Pleistocene | China | ||
Mangaorapan | 53.0 | 49.5 | age | Eocene | New Zealand | ||
Mangapanian | 3.00 | 2.40 | age | Pliocene-Pleistocene | New Zealand | ||
Marahuan | 2.2 | 1.8 | sub-age | Pleistocene | New Zealand | ||
Mayoian | 12.0 | 10.0 | age | Miocene | South America | ||
Meghalayan | 4.2 | 0.0 | age | Quaternary | India | ||
Menapian | 1.03 | super-age | Pleistocene | Netherlands | Menapii, Germanic tribe | ||
Merksemian | ~2.5 | ~2 | age | Pleistocene | Belgium (obsolete) | Merksem | de Heinzelin, 1958 |
Mesozoic | 251.0 ± 0.7 | 65.5 ± 0.3 | era | ICS | middle life | ||
Messinian | 7.246 | 5.332 | age | Miocene | ICS | Messina (Italy) | Mayer-Eymar, 1867 |
Middle Miocene | 15.97 | 11.608 | age | Miocene | ICS | ||
Middle Pleistocene | 0.73 | 0.15 | age | Pleistocene | ICS | ||
Mindel | 0.85 | 0.465 | age | Pleistocene | Alps | river Mindel (Germany) | |
Mindel-Riss | 0.465 | 0.238 | age | Pleistocene | Alps | ||
Miocene | 23.03 | 5.332 | epoch | Neogene | ICS | Greek: less recent | Lyell, 1847 |
Mitchellian | 10.5 | 5.0 | age | Pliocene-Miocene | Australia | ||
Mohnian | 13.5 | 7.5 | age | Miocene | California | ||
Monroecreekian | 26.3 | 24.8 | age | Oligocene | North America | Monroe Creek | |
Montehermosan | 6.8 | 4.0 | age | Pliocene-Miocene | South America | ||
Montian | ~65 | ~61 | age | Paleocene | Europe (obsolete) | Mons (Belgium) | Dewalque, 1868 |
Morozovan | 0.8 | 0.5 | age | Pleistocene | Eastern Europe | ||
Mustersan | 48 | 42 | age | Eocene | South America | ||
Nanzian | 48 | 35 | age | Eocene | California | ||
Nebraskan | 0.93 | 0.6 | age | Pleistocene | North America (obsolete) | ||
Needian | 0.42 | 0.38 | age | Pleistocene | Netherlands (obsolete) | Neede | |
Neocomian | 145.5 | 125.0/130.0 | epoch | obsolete | Neocomium, Latin name for Neuchâtel | ||
Neogene | 23.0 | 2.588 | period | Cenozoic | ICS | Hoernes, 1856 | |
Neporatan | 2.5 | 1.7 | age | Pleistocene | Eastern Europe | ||
Neustrian | 55.0 | 50.8 | ELMMZ | Paleocene-Eocene | Europe | ||
Nomentanan | 0.24 | 0.13 | age | Pleistocene | Italy | ||
Nongshanian | 62.9 | 56.9 | ALMA | Paleocene | Asia | ||
Northgrippian | 8.236 | 4.2 | Holocene | Greenland | |||
Nukumaruan | 3.1 | 1.8 | age | Pleistocene | New Zealand | ||
Odessan | 1.2 | 0.8 | age | Pleistocene | Eastern Europe | ||
Okehuan | 1.1 | 0.37 | age | Pleistocene | New Zealand | ||
Older Dryas | 13,480 BP | 13,350 BP | chron | Weichselian | Europe | Dryas octopetala (plant) | |
Oldest Dryas | 13,860 | 13,780 | chron | Weichselian | Europe | Dryas octopetala (plant) | |
Oligocene | 33.9 ± 0.1 | 23.03 | epoch | Paleogene | ICS | "not so recent" | Beyrich, 1857 |
Oostermeer Interglacial | 0.243 | 0.238 | age | Pleistocene | Netherlands | Oostermeer | |
Opoitian | 5.0 | 3.8 | age | Pliocene | New Zealand | ||
Orellan | 33.9 | 33.3 | age | Oligocene | North America | ||
Orleanian | 20.4 | 15 | ELMMZ | Miocene | Europe | Orléans (France) | |
Otaian | 21.7 | 19.0 | age | Miocene | New Zealand | ||
Ottnangian | 18.3 | 17.0 | age | Miocene | Paratethys | Ottnang am Hausruck (Austria) | Papp & Rögl, 1967 |
Paleocene | 65.5 ± 0.3 | 55.8 ± 0.2 | epoch | Paleogene | ICS | oldest recent | Schimper, 1847 |
Paleophytic | ~450 | ~270 | era | paleobotany | old flora | ||
Paleogene | 65.5 ± 0.3 | 23.0 | period | Cenozoic | ICS | Hoernes, 1856 | |
Paleozoic | 542.0 ± 1.0 | 251.0 ± 0.7 | era | Phanerozoic | ICS | old life | |
Pannonian | 11.608 ± 0.005 | 7.246 ± 0.005 | age | Miocene | Paratethys | Pannonia (Roman province) | Roth von Telegd, 1879 |
Pareora | 21.7 | 15.9 | epoch | Neogene | New Zealand | ||
Pastonian | ~1.87 | 1.77 | age | Pleistocene | Great Britain | Paston, Norfolk (England) | |
Peligran | 62.5 | 59 | age | Paleocene | South America | ||
Penutian | 53 | 51 | age | Eocene | California | ||
Phanerozoic | 542.0 ± 1.0 | present | eon | ICS | visible life | ||
Piacenzian | 3.600 | 2.588 | age | Pliocene | ICS | Piacenza (Italy) | Mayer-Eymar, 1858 |
Pleistocene | 2.588 | 0.0117 | epoch | Quaternary | ICS | youngest recent | |
Pleniglacial | 73,000 BP | 14,500 BP | sub-age | Pleistocene | Northern Europe | ||
Pliocene | 5.332 | 2.588 | epoch | Neogene | ICS | newer recent | Lyell, 1847 |
Poederlian | ~3.5 | ~2.5 | age | Pliocene | Belgium (obsolete) | Poederlee | Vincent, 1889 |
Pontian | 7.246 ± 0.005 | 5.332 ± 0.005 | epoch | Miocene | Paratethys | Pontus Euxinus, Latin name for the Black Sea | Le Play, 1842 |
Pontinian | 0.1 | 0.01 | age | Pleistocene | Italy | ||
Porangan | 46.2 | 43.0 | age | Eocene | New Zealand | ||
Preboreal | 11,560 BP | 10,640 BP | chron | Northern Europe | before the Boreal | ||
Precambrian | none | 542.0 ± 1.0 | none (before: eon) | worldwide | before the Cambrian | ||
Pre-Illinoian | age | Pleistocene | North America | before the Illinoian | |||
Preludhamian | ~2.52 | ~2.61 | age | Pliocene-Pleistocene | Great Britain | before the Ludhamian | |
Prepastonian | ~2.0 | ~1.87 | age | Pleistocene | Great Britain | before the Pastonian | |
Pretiglian | 2.588 | 2.40 | super-age | Pleistocene | Netherlands | before the Tiglian Tegelen (The Netherlands) | Van der Vlerk, 1948 |
Priabonian | 37.2 ± 0.1 | 33.9 ± 0.1 | age | Eocene | ICS | Priabona (Italy) | Munier-Chalmas & De Lapparent, 1893 |
Proterozoic | 2,500 | 542.0 ± 1.0 | eon | ICS | |||
Puercan | 65.5 | 63.3 | age | Paleocene-Cretaceous | North America | ||
Putikian | 0.37 | 0.01 | age | Pleistocene | New Zealand | ||
Quaternary | 2.588 | present | period | Cenozoic | ICS | fourth part | Arduino, 1760 |
Rancholabrean | NALMA | Pleistocene | North America | ||||
Refugian | 35.0 | 33.5 | age | Oligocene-Eocene | California | ||
Reinbekian | age | Miocene | Northern Germany | ||||
Relizian | 16.5 | 13.5 | age | Miocene | California | ||
Repettian | 2.9 | 2.2 | age | Pliocene-Pleistocene | California | ||
Reuverian | 3.5 | 2.558 | chronozone | Pliocene | Northwest Europe | Reuver (The Netherlands) | |
Riochican | 57 | 54 | age | Eocene-Paleocene | South America | ||
Riss Glacial | 0.238 | 0.128 | age | Pleistocene | Alps | river Riß (Germany) | |
Riss-Würm Interglacial | 0.128 | 0.116 | age | Pleistocene | Alps | ||
Robiacian | 42.7 | 37.2 | ELMMZ | Miocene | Europe | ||
Romanian | 3.6 | 1.8 | age | Pliocene-Pleistocene | Paratethys | ||
Runangan | 36.0 | 34.3 | age | Eocene-Oligocene | New Zealand | ||
Rupelian | 33.9 ± 0.1 | 28.4 ± 0.1 | age | Oligocene | ICS | river Rupel (Belgium) | Dumont, 1850 |
Ruscinian | 4.9 | 3.5 | ELMMZ | Pliocene | Europe | Ruscino, Latin for the Roussillon (France) | Kretzoi, 1962 |
Saalian | 0.238 | 0.128 | age | Pleistocene | Northern Europe | river Saale (Germany) | |
Sangamonian | 0.125 | 0.075 | age | Pleistocene | North America | ||
Santacrucian | 17.5 | 16.3 | age | Miocene | South America | ||
Santomian | 1.81 | 1.5 | sub-age | Pleistocene | Italy | ||
Sarmatian | 12.7 | 11.6 | age | Miocene | Paratethys | Sarmatians (ancient people) | Suess, 1866 |
Saucesian | 22.0 | 16.5 | age | Miocene | California | ||
Scaldisian | ~4 | ~2.5 | age | Pliocene | Belgium (obsolete) | Scaldus, Latin name for the river Scheldt | Dumont, 1850 |
Scythian | 251 ± 0.2 | 245 ± 1.5 | Epoch | Early Triassic | Europe | Scythia | |
Selandian | 61.7 ± 0.2 | 58.7 ± 0.2 | age | Paleocene | ICS | Seeland (Denmark) | Rosenkrantz, 1924 |
Serravallian | 13.65 | 11.608 | age | Miocene | ICS | Serravalle Scrivia (Italy) | Pareto, 1864 |
Shanghuan | 65.5 | 62.9 | ALMA | Paleocene | Asia | ||
Sharamurunian | 40.4 | 37.2 | ALMA | Eocene | Asia | ||
Sicilian | 0.781 | 0.260 | sub-age | Pleistocene | Italy | Sicily | |
Southland | 15.9 | 10.9 | epoch | Neogene | New Zealand | ||
Stampian | age | Oligocene | western Europe | Étampes (France) | d'Orbigny, 1852 | ||
Subatlantic | 2400 BP | 0 | chron | Holocene | Northern Europe | ||
Subboreal | 5660 BP | 2400 BP | chron | Holocene | Northern Europe | ||
Suchian | 3.0 | 1.9 | age | Pliocene-Pleistocene | Japan | ||
Suevian | 33.8 | 29.2 | ELMMZ | Oligocene | Europe | ||
Susterian | 8.5 | 5.3 | chronozone | Miocene | Northwest Europe | Susteren (The Netherlands) | |
Syltian | age | Miocene | Northern Germany | ||||
Tabenbulakian | 24.0 | 23.03 | ALMA | Oligocene | Asia | ||
Taranaki | 10.9 | 5.28 | epoch | Neogene | New Zealand | ||
Tarantian | 0.15 | 0.0115 | age | Pleistocene | Southern Europe | Tarento (Italy) | |
Taxandrian | 1.80 | 0.418 | super-age | Pleistocene | Netherlands (obsolete) | ||
Tertiary | 65.5 ± 0.3 | 2.588 | sub-era | Cenozoic[5] | worldwide | third part | Arduino, 1760 |
Teurian | 65.0 | 55.5 | age | Paleocene | New Zealand | ||
Thanetian | 58.7 ± 0.2 | 55.8 ± 0.2 | age | Paleocene | ICS | Isle of Thanet (England) | Renevier, 1874 |
Thurnian | ~2.25 | ~2.12 | age | Pleistocene | Great Britain | River Thurne (England} | West, 1961 |
Tiffanian | 60.2 | 56.8 | age | Paleocene | North America | ||
Tiglian | 2.40 | 1.80 | super-age | Pleistocene | Netherlands | Tegelen (The Netherlands) | |
Tinguirirican | 36 | 29 | age | Oligocene-Eocene | South America | ||
Tiupampan | 64.5 | 62.5 | age | Paleocene | South America | Tiupampa | Marshall & de Muizon, 1988 |
Tongaporutuan | 10.9 | 6.5 | age | Miocene | New Zealand | ||
Tongrian | age | Oligocene | western Europe | ||||
Torrejonian | 63.3 | 60.2 | age | Paleocene | North America | ||
Tortonian | 11.608 | 7.246 | age | Miocene | ICS | Tortona (Italy) | Mayer-Eymar, 1858 |
Totomian | 3.6 | 3.0 | age | Pliocene | Japan | ||
Tozawan | 15.97 | 13.5 | age | Miocene | Japan | ||
Treenean | 0.15 | 0.17 | chronozone | Pleistocene | Northwest Europe | ||
Tubantian | 0.116 | 0.0115 | age | Pleistocene | Netherlands (obsolete) | Van der Vlerk & Florschütz, 1950 | |
Turolian | 8.7 | 4.9 | ELMMZ | Miocene-Pliocene | Europe | Turolium, Latin for Teruel (Spain) | Crusafont, 1965 |
Tyrrhenian | 0.26 | 0.01143 | sub-age | Pleistocene | Italy | Tyrrhenian Sea | Issel, 1914 |
Uintan | 46.2 | 42.0 | age | Eocene | North America | ||
Ulangochuian | 37.2 | 35.1 | ALMA | Eocene | Asia | ||
Ulatisian | 51 | 48 | age | Eocene | California | ||
Uquian | 3.0 | 1.2 | age | Pliocene-Pleistocene | South America | Uquia (Argentina) | Castellanos, 1923 |
Vallesian | 11.1 | 8.7 | ELMMZ | Miocene | Europe | The Vallès (Spain) | Crusafont, 1950 |
Venturian | 2.2 | 1.9 | age | Pleistocene | California | ||
Vierlandian | age | Miocene | Northern Germany | ||||
Vicksburgian | age | Oligocene | southern US | ||||
Villafranchian | 3.5 | 1.1 | ELMMZ | Pliocene-Pleistocene | Europe | ||
Waalian | 1.45 | 1.20 | super-age | Pleistocene | Netherlands | river Waal (river)|Waal | |
Waiauan | 12.7 | 10.9 | age | Miocene | New Zealand | ||
Waipawan | 55.5 | 53.0 | age | Eocene | New Zealand | ||
Waipipian | 3.60 | 3.00 | age | Pliocene | New Zealand | ||
Waitakian | 25.2 | 21.7 | age | Oligocene-Miocene | New Zealand | ||
Waitotaran | 3.8 | 3.1 | sub-age | Pliocene | New Zealand | ||
Waltonian | ~2.52 | age | Pliocene | Great Britain | Walton-on-the-Naze | Harmer, | |
Wanganui | 5.28 | present | epoch | Neogene-Quaternary | New Zealand | Wanganui | |
Wangerripian | age | Paleocene-Eocene | Australia | ||||
Warthian | 0.15 | 0.13 | chronozone | Pleistocene | Northwest Europe | ||
Wasatchian | 55.4 | 50.3 | age | Eocene | North America | ||
Weichselian | 0.116 | 0.0115 | age | Northern Europe | Weichsel, German name for the river Vistula (Poland) | ||
Werrikooian | 1.00 | 1.806 | age | Pleistocene | Australia | ||
Whaingaroan | 34.3 | 27.3 | age | Oligocene | New Zealand | ||
Wheelerian | 1.9 | 0.01143 | age | Pleistocene | California | ||
Whitneyan | 33.3 | 30.8 | age | Oligocene | North America | ||
Wisconsinan | 0.075 | 0.01 | age | Pleistocene | North America | ||
Wolstonian | 0.238 | 0.128 | age | Pleistocene | Great Britain | Wolston (England) | |
Wuchenghuangtuan | 2.4 | 1.2 | age | Pleistocene | China | ||
Würm Glacial | 0.116 | 0.0115 | age | Pleistocene | Alps | river Würm (Germany) | |
Yarmouthian | 0.26 | 0.17 | age | Pleistocene | North America | Aegean Sea | |
Yatalan | 3.4 | 2.0 | age | Pliocene-Pleistocene | Australia | ||
Younger Dryas | 12,700 BP | 11,560 BP | chron | Weichselian | Northern Europe | Dryas octopetala (plant) | |
Ypresian | 55.8 ± 0.2 | 48.6 ± 0.2 | age | Eocene | ICS | Ypres, French name for Ieper (Ieper) in Belgium |
Dumont, 1849 |
Ynezian | 61.5 | 55.8 | age | Paleocene | California | ||
Yuian | 9.5 | 3.6 | age | Pliocene-Miocene | Japan | ||
Yuzanjian | 1.5 | 0.75 | age | Pleistocene | Japan | ||
Zanclean | 5.332 | 3.60 | age | Pliocene | ICS | Zancla, old name for Messina (Italy) | Sequenza, 1868 |
Zemorrian | 33.5 | 22.0 | age | Oligocene-Miocene | California |
Cenozoic[edit | edit source]
"Our assessment of published radiometric dates suggests the following best biochronologic age estimates for Cenozoic Epoch boundaries:"[6]
- Pliocene/Pleistocene: <2 Ma;
- Miocene/Pliocene: ~5 Ma;
- Oligocene/Miocene: ~23.5 Ma;
- Eocene/Oligocene: ~37 Ma;
- Paleocene/Eocene: ~56.5 Ma;
- Cretaceous/Tertiary: ~66 Ma.
"The radiometric data on which these age estimates are based, especially in the Paleogene, are biased toward those obtained from high-temperature minerals; age estimates based on radiometric dates from glauconites tend to be younger, particularly in the Paleogene (for example, Odin and others, 1982)."[6]
Quaternary[edit | edit source]


Quaternary is the current and most recent of the three periods of the Cenozoic Era in the geologic time scale of the International Commission on Stratigraphy (ICS).[7] It follows the Neogene Period and spans from 2.588 ± 0.005 million years ago to the present.[7] The Quaternary Period is divided into two epochs: the Pleistocene (2.588 million years ago to 11.7 thousand years ago) and the Holocene (11.7 thousand years ago to today, although a third epoch, the Anthropocene, has been proposed but is not yet officially recognized by the ICS).[7] The informal term "Late Quaternary" refers to the past 0.5–1.0 million years.[8]
The Quaternary Period is typically defined by the cyclic growth and decay of continental ice sheets related to the Milankovitch cycles and the associated climate and environmental changes that they caused.[9][10]
In 2009, it was decided to make the Quaternary the youngest period of the Cenozoic Era with its base at 2.588 Mya and including the Gelasian stage, which was formerly considered part of the Neogene Period and Pliocene Epoch.[11]
The Anthropocene has been proposed as a third epoch as a mark of the anthropogenic impact on the global environment starting with the Industrial Revolution, or about 200 years ago.[12] The Anthropocene is not officially designated by the ICS, but a working group has been working on a proposal for the creation of an epoch or sub-period.[13]
The 2.6 million years of the Quaternary represents the time during which recognizable humans existed.[14] Over this geologically short time period there has been relatively little change in the distribution of the continents due to plate tectonics.
The major geographical changes during this time period included the emergence of the Strait of Bosphorus and Skagerrak during glacial epochs, which respectively turned the Black Sea and Baltic Sea into fresh water lakes, followed by their flooding (and return to salt water) by rising sea level;[15] the periodic filling of the English Channel, forming a land bridge between Britain and the European mainland; the periodic closing of the Bering Strait, forming Beringia, the land bridge between Asia and North America; and the periodic flash flooding of Scablands of the American Northwest by glacial water.[16]
The current extent of Hudson Bay, the Great Lakes and other major lakes of North America are a consequence of the Canadian Shield's readjustment since the last ice age; different shorelines have existed over the course of Quaternary time.[17]
The climate was one of periodic glaciations with continental glaciers moving as far from the poles as 40 degrees latitude. There was a major extinction of large mammals in Northern areas at the end of the Pleistocene Epoch. Many forms such as saber-toothed cats, mammoths, mastodons, glyptodonts, etc., became extinct worldwide. Others, including horses, Camelops and American cheetahs became extinct in North America.[18][19]
The "whole change elapsed just opposite the course of events that characterized the great glacial oscillations with sudden warming followed by slow cooling. Therefore, the two phenomena hardly have the same cause."[20]
"In the Greenland ice cores, the Pleistocene–Holocene transition is chronologically constrained between two clearly defined tephra horizons: the Saksunarvatn tephra (1409.83 m depth) and the Vedde Ash (1506.14 m depth). These are dated at 10 347 yr b2 k (counting uncertainty 89 yr) and 12 171 yr (counting uncertainty 114 yr) b2 k, respectively."[1]
Meghalayan[edit | edit source]
The Meghalayan is the latest age or uppermost stage of the Quaternary.[21] It is also the upper, or latest, of three subdivisions of the Holocene epoch or series.[22][23] It was officially ratified by the International Commission on Stratigraphy in June 2018 along with the earlier Greenlandian and Northgrippian ages/stages.[21] Its Global Boundary Stratotype Section and Point (GSSP) is a Krem Mawmluh Cave formation in Meghalaya, a state in Northeast India.[24] Mawmluh cave is one of the longest and deepest caves in India, and conditions here were suitable for preserving chemical signs of the transition in ages.[25] The global auxiliary stratotype is an ice core from Mount Logan in Canada.[26]
The Meghalayan begins 4,200 years Before Present (BP), i.e., before 1950 ca 2250 BCE or 7750 Holocene calendar (HE),[27] leaving open room for the possible creation of the Anthropocene from 1950 forward.[28][29] Helama & Oinonen (2019) dated the start of the Meghalayan to 2190-1990 BCE.[30] The age began with a 4.2 kiloyear event (200-year drought that impacted human civilizations) in the Eastern Mediterranean, Mesopotamia, the Indus Valley and the Yangtze civilization (Yangtze River Valley).[27] "The fact that the beginning of this age coincides with a cultural shift caused by a global climate event makes it unique," according to Stanley Finney, Secretary General of the International Union of Geological Sciences.[29]
Northgrippian[edit | edit source]
The Northgrippian is the middle one of three ages or stages of the Holocene Epoch or series.[31][32][33][34] It was officially ratified by the International Commission on Stratigraphy in June 2018 along with the earlier Greenlandian and later Meghalayan ages/stages. The age takes its name from the North Greenland Ice Core Project (NorthGRIP).[32] The age began 8,236 years before the year 2000, 6236 BCE or 3764 Holocene calendar (HE), near the 8.2 kiloyear event, and goes up to the start of the Meghalayan, which began 4,200 years before the year 1950 (2250 BCE or 7750 HE), near the 4.2 kiloyear event.[35]
Holocene[edit | edit source]

The Holocene starts at ~11,700 b2k and extends to the present.
"A timescale based on multi-parameter annual layer counting provides an age of 11 700 calendar yr b2k (before AD 2000) for the base of the Holocene, with a maximum counting error of 99 yr."[1]
"The base of the Holocene Series/Epoch is defined in the NGRIP ice-core record [above] at the horizon which shows the clearest signal of climatic warming, an event that marks the end of the last cold episode (Younger Dryas Stadial/Greenland Stadial 1) of the Pleistocene [...]."[1]
Subatlantic period[edit | edit source]
The "calibration of radiocarbon dates at approximately 2500-2450 BP [2500-2450 b2k] is problematic due to a "plateau" (known as the "Hallstatt-plateau") in the calibration curve [...] A decrease in solar activity caused an increase in production of 14C, and thus a sharp rise in Δ 14C, beginning at approximately 850 cal (calendar years) BC [...] Between approximately 760 and 420 cal BC (corresponding to 2500-2425 BP [2500-2425 b2k]), the concentration of 14C returned to "normal" values."[36]
Subboreal period[edit | edit source]
The "period around 850-760 BC, [2850-2760 b2k, is] characterised by a decrease in solar activity and a sharp increase of Δ 14C [...] the local vegetation succession, in relation to the changes in atmospheric radiocarbon content, shows additional evidence for solar forcing of climate change at the Subboreal - Subatlantic transition."[36]
The "Holocene climatic optimum in this interior part of Asia [Lake Baikal] corresponds to the Subboreal period 2.5–4.5 ka".[37]
Bronze Age[edit | edit source]
Atlantic[edit | edit source]
The "Atlantic period [is from] 4.6–6 ka [6,000 to 4,600 b2k]."[37]
Use of flax steadily spread, reaching as far as Switzerland and Germany by 5,000 years ago.[38] In China and India, domesticated flax was cultivated at least 5,000 years ago.[39]
"The last remains of the American ice sheet disappeared about 6000 years ago [6,000 b2k]".[20]
Beginning with the temperatures, as derivable from Greenland ice core data, it is possible to define an 'Early' or 'Pre-Atlantic' period at around 8040 BC, where the 18O isotope line remains above 33 ppm in the combined curve after Rasmussen et al. (2006),[40] which then would end at the well-known 6.2 ka BC (8.2 ka calBP)-cold-event.
Boreal transition[edit | edit source]
Evidence exists of a domesticated oilseed flax with increased seed-size from Tell Ramad in Syria[41] and flax fabric fragments from Çatalhöyük in Turkey[42] by circa 9,000 years ago.
"In recent years, the German oak chronology has been extended to 7938 BC [9938 b2k]. For earlier intervals, tree-ring chronologies must be based on pine, because oak re-emigrated to central Europe at the Preboreal/Boreal transition, at about 8000 BC [10,000 b2k]."[43]
"The age range, 7145-7875 BC [9145-9875 b2k], is represented by the oak chronology, 'Main9'."[43]
"The age range, 7833-9439 BC [9833-11439 b2k], is covered by the 1784-yr pine chronology."[43]
Pre-Boreal transition[edit | edit source]
The last glaciation appears to have a gradual decline ending about 12,000 b2k. This may have been the end of the Pre-Boreal transition.
Mesolithic[edit | edit source]
Late Pleistocene[edit | edit source]
Late Pleistocene spans ca. 11,000-150,000 yr BP.[44]
The Late Pleistocene is an unofficial age in the international geologic timescale in chronostratigraphy, also known as Upper Pleistocene from a stratigraphic perspective. It is intended to be the fourth division of the Pleistocene Epoch within the ongoing Quaternary Period. It is currently estimated to span the time between c. 129,000 and c. 11,700 years ago. The Late Pleistocene equates to the proposed Tarantian Age of the geologic time scale, preceded by the officially ratified Chibanian (formerly known as Middle Pleistocene) and succeeded by the officially ratified Greenlandian.[45] The estimated beginning of the Tarantian is the start of the Eemian interglacial period (Marine Isotope Stage 5).[46] It is held to end with the termination of the Younger Dryas, some 11,700 years ago when the Holocene Epoch began.[47]
The term Upper Pleistocene is currently in use as a provisional or "quasi-formal" designation by the International Union of Geological Sciences (IUGS). Although the three oldest ages of the Pleistocene (the Gelasian, the Calabrian and the Chibanian) have been officially defined, the Late Pleistocene has yet to be formally defined, along with consideration of a proposed Anthropocene sub-division of the Holocene.[48]
The main feature of the Late Pleistocene was glaciation, for example the Würm glaciation in the Alps of Europe, to 14 ka, and the subsequent Younger Dryas. Many megafauna became Quaternary extinction event during this age, a trend that continued into the Holocene. In palaeoanthropology, the Late Pleistocene contains the Upper Palaeolithic stage of human development, including many of the early human migrations and the extinction of the last remaining archaic human species.
The proposed beginning of the Late Pleistocene is the end of the Penultimate Glacial Period (PGP) 126 ka when the Riß glaciation (Alpine) was being succeeded by the Eemian (Riß-Würm) interglacial period.[49] The Riß-Würm ended 115 ka with the onset of the Last Glacial Period (LGP) which is known in Europe as the Würm glaciation (Alpine) or Devensian glaciation (Great Britain) or Weichselian glaciation (northern Europe); these are broadly equated with the Wisconsin glaciation (North America), though technically that began much later.[49]
The Last Glacial Maximum was reached during the later millennia of the Würm/Weichselian, estimated between 26 ka and 19 ka when deglaciation began in the Northern Hemisphere. The Würm/Weichselian endured until 16 ka with Northern Europe, including most of Great Britain, covered by an ice sheet. The glaciers reached the Great Lakes in North America.[47] Sea levels fell and two land bridges were temporarily in existence that had significance for human migration: Doggerland, which connected Great Britain to mainland Europe; and the Bering land bridge which joined Alaska to Siberia.[50][51]
The Last Ice Age was followed by the Late Glacial Interstadial, a period of global warming to 12.9 ka, and the Younger Dryas, a return to glacial conditions until 11.7 ka. Palaeoclimatology holds that there was a sequence of stadials and interstadials from about 16 ka until the end of the Pleistocene. These were the Oldest Dryas (stadial), the Bølling oscillation (interstadial), the Older Dryas (stadial), the Allerød oscillation (interstadial) and finally the Younger Dryas.[52]
The end of the Younger Dryas marks the boundary between the Pleistocene and Holocene Epochs. Man in all parts of the world was still culturally and technologically in the Palaeolithic (Old Stone) Age. Tools and weapons were basic stone or wooden implements. Nomadic tribes followed moving herds. Non-nomadics acquired their food by gathering and hunting.[53]
In Egypt, the Late (or Upper) Palaeolithic began sometime after 30,000 BC. People in North Africa had relocated to the Nile Valley as the Sahara was transformed from grassland to desert.[54] The Nazlet Khater skeleton was found in 1980 and has been radiocarbon dated to between 30,360 and 35,100 years ago.[55][56]
Flandrian interglacial[edit | edit source]
The first part of the Flandrian, known as the Younger Atlantic, was a period of fairly rapid sea level rise,[57] known as the Flandrian transgression and associated with the melting of the Fenno-Scandian, Scottish, Laurentide and Cordilleran Cordilleran glaciers.
Fjords were formed during the Flandrian transgression when U-shaped glaciated valleys were inundated with water.[58]
The Flandrian began as the relatively short-lived Younger Dryas climate downturn came to an end. This formed the last gasp of the Devensian glaciation.
Younger Dryas[edit | edit source]
The "Alleröd/Younger Dryas transition [occurred] some 11,000 years ago [11,000 b2k]."[59]
"The Younger Dryas interval during the Last Glacial Termination was an abrupt return to glacial-like conditions punctuating the transition to a warmer, interglacial climate."[60]
"From former cirque glaciers in western Norway, it is calculated that the summer (1.May to 30.September) temperature dropped 5-6°C during less than two centuries, probably within decades, at the Alleröd/Younger Dryas transition, some 11,000 years ago."[59]
Greenlandian[edit | edit source]
The 'Greenlandian is the earliest age or lowest stage of the Holocene epoch or series, part of the Quaternary.[45][61] Beginning in 9700 BCE (300 HE, 11700 b2k) and ending 6236 BCE (3764 HE, 8236 b2k),[62] it is the earliest of three sub-divisions of the Holocene, and was officially ratified by the International Commission on Stratigraphy in June 2018 with the later Northgrippian and Meghalayan ages/stages.[61] The lower boundary of the Greenlandian Age is the Global Boundary Stratotype Section and Point (GSSP) sample from the North Greenland Ice Core Project in central Greenland (75.1000°N 42.3200°W).[63] The Greenlandian GSSP has been correlated with the end of Younger Dryas (from near-glacial to interglacial) and a "shift in deuterium excess values".[63]
Allerød Oscillation[edit | edit source]
The "Allerød Chronozone, 11,800 to 11,000 years ago".[59]
"During the Allerød Chronozone, 11,800 to 11,000 years ago, western Europe approached the present day environmental and climatic situation, after having suffered the last glacial maximum some 20,000 to 18,000 years ago. However, the climatic deterioration 11,000 years ago led to nearly fully glacial conditions on this continent for some few hundreds of years during the Younger Dryas. This change is completely out of phase with the Milankovitch (orbital) forcing as this is understood today, and therefore its cause is of major interest."[59]
"Excess 14C in Cariaco Basin sediments indicates a slowing in thermohaline circulation and heat transport to the North Atlantic at that time, and both marine and terrestrial paleoclimate proxy records around the North Atlantic show a short-lived (<400 yr) cold event (Intra-Allerød cold period) that began ca. 13,350 yr B.P."[64]
Pleistocene[edit | edit source]
The Pleistocene dates from 2.588 x 106 to 11,700 b2k.
Older Dryas[edit | edit source]

"Older Dryas [...] events [occurred about 13,400 b2k]".[65]
"The most negative δ 18O excursions seen in the GRIP record lasted approximately 131 and 21 years for the [inter-Allerød cold period] IACP and [Older Dryas] OD, respectively. The comparable events in the Cariaco basin were of similar duration, 127 and 21 years. In addition to the chronological agreement, there is also considerable similarity in the decade-scale patterns of variability in both records. Given the geographical distance separating central Greenland from the southern Caribbean Sea, the close match of the pattern and duration of decadal events between the two records is striking."[65]
In the figures on the right, especially b, is a detailed "comparison of δ 18O from the GRIP ice core24 with changes in a continuous sequence of light-lamina thickness measurements from core PL07-57PC. Both records are constrained by annual chronologies, although neither record is sampled at annual resolution. The interval of comparison includes the inter-Allerød cold period (12.9-13 cal. kyr BP) and Older Dryas (13.4 cal. kyr BP) events (IABP and OD from a). The durations of the two events, measured independently in both records, are very similar, as is the detailed pattern of variability at the decadal timescale."[65]
Marine Isotope Stage 1[edit | edit source]
The Earth is currently experiencing an interglacial period (warming) during the present Quaternary Ice Age, identified as the "Marine Isotope Stage 1" (MIS1) in the Holocene epoch (or recently the Anthropocene epoch).
Dansgaard–Oeschger events are considered switches between states of the climate system.[66]
The Holocene period began around 11,700 years ago and continues to the present.[67] Identified with the current warm period, known as "Marine Isotope Stage 1", or MIS 1, the Holocene is considered an interglacial period in the Quaternary glaciation or current Ice Age.
Bølling Oscillation[edit | edit source]

The "intra-Bølling cold period [IBCP is a century-scale cold event and the] Bølling warming [occurs] at 14600 cal [calendar years] BP (12700 14C BP)".[68]
The "Bølling was originally defined as starting from 13000 14C BP (calibrated to ~15650 cal BP; Stuiver et al., 1998). [...] independent annual chronology indicate a much later onset of the Bølling (e.g., 14600 cal BP".[68]
"During the IBCP and perhaps also IACP, δ 18O values inversely correlate with δ 13C, but during the OD δ 18O shows positive correlation with δ 13C, suggesting dry conditions with high evaporation, as well as cold."[68]
Bølling interstadial[edit | edit source]
The Bølling interstadial corresponds to GIS 1 as shown in the diagram on the right.[69]
MIS Boundary 1/2 is at 14 ka.[70]
Marine Isotope Stage 2[edit | edit source]
Termination I, also known as the Last Glacial Termination, is the end of Marine isotope stage 2.
Oldest Dryas[edit | edit source]
"During the Late Weichselian glacial maximum (20-15 ka BP) the overriding of ice streams eventually lead to strong glaciotectonic displacement of Late Pleistocene and pre-Quaternary deposits and to deposition of till."[71]
The "minimum point of GIS-2b (Greenland Stadial sub-event b) [is] identified by Bjork et al. 1998 [at] 17.687 ka BP".[72]
"The synchronous and nearly uniform lowering of snowlines in Southern Hemisphere middle-latitude mountains compared with Northern Hemisphere values suggests global cooling of about the same magnitude in both hemispheres at the [Last Glacial Maximum] LGM. When compared with paleoclimate records from the North Atlantic region, the middle-latitude Southern Hemisphere terrestrial data imply interhemispheric symmetry of the structure and timing of the last glacial/interglacial transition. In both regions atmospheric warming pulses are implicated near the beginning of Oldest Dryas time (~14,600 14C yr BP) and near the Oldest Dryas/Bølling transition (~12,700-13,000 14C yr BP). The second of these warming pulses was coincident with resumption of North Atlantic thermohaline circulation similar to that of the modern mode, with strong formation of Lower North Atlantic Deep Water in the Nordic Seas. In both regions, the maximum Bølling-age warmth was achieved at 12,200-12,500 14C yr BP, and was followed by a reversal in climate trend. In the North Atlantic region, and possibly in middle latitudes of the Southern Hemisphere, this reversal culminated in a Younger-Dryas-age cold pulse."[73]
Meiendorf Interstadial[edit | edit source]

The period spans starting at the far right of the image on the right from Lascaux interstadial to Heinrich event H1, and to Meiendorf/Bölling warm stage, and Alleröd warm stage, to Younger dryas and early holocene.
The Dansgaard-Oeschger event 1 corresponds to the Meiendorf Interstadial.
The beginning of the Meiendorf Interstadial is around 14,700 b2k.
Heinrich event H1[edit | edit source]
This stadial starts about 17.5 ka, extends to about 15.5 ka and is followed after a brief warming by H1.
Dolní Veštonice cold event 6-7[edit | edit source]
This stadial occurred at 16 ka.
The Dolní Veštonice (DV) are cold events marked by ice wedges in the upper loess of Dolní Veštonice.
Altonian[edit | edit source]
The Altonian began about 19.0-18.7 Ma and lasted to 15.9 Ma.
Lascaux interstadial[edit | edit source]
The Lascaux interstadial begins about 21 ka and extends to about 18 ka.
Jylland stade[edit | edit source]
"After c. 22 ka BP during the Jylland stade (Houmark-Nielsen 1989), Late Weichselian glaciers of the Main Weichselain advance overrode Southeast Denmark from the northeast and later the Young Baltic ice invaded from southeasterly directions. Traces of the Northeast-ice are apparently absent in the Klintholm sections, although large scale glaciotectonic structures and till deposits from this advance are found in Hjelm Bugt and Møns Klint (Aber 1979; Berthelsen 1981, 1986). At Klintholm, the younger phase of glaciotectonic deformation from the southeast and south and deposition of the discordant till (unit 9) were most probably associated with recessional phases of the Young Baltic glaciation. In several cliff sections, well preserved Late Glacial (c. 14-10 ka BP) lacustrine sequences are present (Kolstrup 1982, Heiberg 1991)."[71]
The Jylland stadial corresponds to Dolní Veštonice cold event 3-4 at about 21.2 ka.
Laugerie Interstadial[edit | edit source]

The weak interstadial corresponding to GIS 2 (Dansgaard-Oeschger event 2) occurred about 23.2 kyr B.P.[69]
The δ18O values from GISP-2 follow the diagram of Wolfgang Weißmüller. The positions of the Dansgaard-Oeschger events DO1 to DO4 and the Heinrich events H1 to H3 are also indicated. DV 3-4 and DV 6-7 are cold events marked by ice wedges in the upper loess of Dolní Veštonice.
Letzteiszeitliches Maximum[edit | edit source]
This glacial advance begins about 26 ka and ends abruptly at about 23.4 ka.
Dansgaard-Oeschger event 3[edit | edit source]
The stronger GIS 3 interstadial occurred about 27.6 kyr B.P.[69]
It begins abruptly at 29 ka and ends about 26 ka.
"GIS 3 (start) 25.571 [to] GIS 3 (end) 25.337 ka BP".[72]
Stadial[edit | edit source]
Heinrich Event 3 (H3) "occurs at 26.74 ka BP, coincident with the start of the transition into GIS 4."[72]
MIS Boundary 2/3 is at 29 ka.[70]
"Stadial duration 0.768 ka".[72]
Møn interstadial[edit | edit source]
The Møn interstadial (Dansgaard-Oeschger event 4) corresponds to GIS 4 at 29 ka.[69]
Klintholm advance[edit | edit source]
This advance occurred after the Møn and ended with GIS 6.[69]
"Stadial duration 2.899 ka".[72]
GIS 5[edit | edit source]
GIS 5 interstadial occurred during the Klintholm advance about 33.5 kyr B.P.[69]
"GIS 5 (start) 30.013 [to] GIS 5 (end) 29.526 ka BP".[72]
The earliest evidence of humans using wild flax as a textile comes from the present-day Republic of Georgia, where spun, dyed, and knotted wild flax fibers found in Dzudzuana Cave date to the Upper Paleolithic, 30,000 years ago.[74][75][76] Humans first domesticated flax in the Fertile Crescent region.[41]
Stadial[edit | edit source]
Stadial duration 0.836 ka""[72]
Ålesund Interstadial[edit | edit source]
The Ålesund interstadial began with GIS 6 and ended after GIS 8.[69]
"GIS 6 (start) 31.218 [to] GIS 6 (end) 30.849 ka BP".[72]
Stadial[edit | edit source]
"Stadial duration 0.932 ka".[72]
GIS 7 interstadial[edit | edit source]
"GIS 7 (start) 32.896 [to] GIS 7 (end) 32.15 ka BP".[72]
Stadial[edit | edit source]
"Stadial duration 0.642 ka".[72]
Huneborg interstadial[edit | edit source]
The Huneborg interstadial is a Greenland interstadial dating 36.5-38.5 kyr B.P. GIS 8.[69]
The Denekamp interstadial corresponds to the Huneborg interstadial.
"GIS 8 (start) 35.716 [to] GIS 8 (end) 33.977 ka BP".[72]
Heinrich Event 4[edit | edit source]
Heinrich Event 4 "33-39.93 ka BP".[72]
Hengelo interstadial[edit | edit source]
The Hengelo interstadial [is] > 35 ka BP".[71]
The "Hengelo Interstadial [is] (38–36 ka ago)."[77]
"An evolution with the coldest phases (coarsest grains) between 27,000 and 10,000 years B.P., 52,000 and 34,000 years B.P., and 76,000 and 60,000 years B.P. and relatively warmer intervals (finer grain size) in between is obvious. Apparently, they reflect a 21,000-year periodicity. This trend is superposed by much shorter oscillations of a duration of one to a few thousand years. Their duration is similar to the Dansgaard-Oeschger oscillations in the ice-core records. Some well-defined stadials and interstadials from the terrestrial records show also such a duration: for instance, the Hengelo interstadial around 37-38,500 14C years B.P. (Zagwijn, 1974; Kasse et al., 1995) and the preceding Hasselo stadial at approximately 40-38,500 14C years B.P. (Van Huissteden, 1990)."[78]
Hasselo stadial[edit | edit source]

The "Hasselo stadial [is] at approximately 40-38,500 14C years B.P. (Van Huissteden, 1990)."[78]
The "Hasselo Stadial [is a glacial advance] (44–39 ka ago)".[77]
The Hasselo stadial corresponds to the Skjonghelleren stadial in Norway but to the Sejrø interstadial in Denmark.[69]
"Paleomagnetic samples were obtained from cores taken during the drilling of a research well along Coyote Creek in San Jose, California, in order to use the geomagnetic field behavior recorded in those samples to provide age constraints for the sediment encountered. The well reached a depth of 308 meters and material apparently was deposited largely (entirely?) during the Brunhes Normal Polarity Chron, which lasted from 780 ka to the present time."[79]
"Three episodes of anomalous magnetic inclinations were recorded in parts of the sedimentary sequence; the uppermost two we correlate to the Mono Lake (~30 ka) geomagnetic excursion and 6 cm lower, tentatively to the Laschamp (~45 ka) excursion."[79]
"Some 41,000 years ago, a complete and rapid reversal of the geomagnetic field occured. Magnetic studies on sediment cores from the Black Sea show that during this period, during the last ice age, a compass at the Black Sea would have pointed to the south instead of north."[80]
"[A]dditional data from other studies in the North Atlantic, the South Pacific and Hawaii, prove that this polarity reversal was a global event."[80]
"The field geometry of reversed polarity, with field lines pointing into the opposite direction when compared to today's configuration, lasted for only about 440 years, and it was associated with a field strength that was only one quarter of today's field."[80]
"The actual polarity changes lasted only 250 years. In terms of geological time scales, that is very fast."[80]
"During this period, the field was even weaker, with only 5% of today's field strength. As a consequence, Earth nearly completely lost its protection shield against hard cosmic rays, leading to a significantly increased radiation exposure."[80]
"This is documented by peaks of radioactive beryllium (10Be) in ice cores from this time, recovered from the Greenland ice sheet. 10Be as well as radioactive carbon (14C) is caused by the collision of high-energy protons from space with atoms of the atmosphere."[80]
"The polarity reversal [...] has already been known for 45 years. It was first discovered after the analysis of the magnetisation of several lava flows near the village Laschamp near Clermont-Ferrand in the Massif Central, which differed significantly from today's direction of the geomagnetic field. Since then, this geomagnetic feature is known as the 'Laschamp event'."[80]
The "new data from the Black Sea give a complete image of geomagnetic field variability at a high temporal resolution."[80]
Moershoofd interstadial[edit | edit source]

The Moershoofd interstadial has a 14C date of 44-46 kyr B.P. and corresponds to GIS 12 at 45-47 kyr B.P.[69]
Marine Isotope Stage 3[edit | edit source]
Inca Huasi was a paleolake in the Andes named by a research team in 2006.[81]
It existed about 46,000 years ago in the Salar de Uyuni basin.[81] Water levels during this episode rose by about 10 metres (33 ft). Overall, this lake cycle was short and not deep,[81] with water levels reaching a height of 3,670 metres (12,040 ft). The lake would have had a surface of 21,000 square kilometres (8,100 sq mi).[82] Most water was contributed to it by the Uyuni-Coipasa drainage basin, with only minimal contributions from Lake Titicaca.[83] Changes in the South American monsoon may have triggered its formation.[84]
Radiocarbon dates on tufa which formed in Lake Inca Huasi were dated at 45,760 ± 440 years ago.[81] Uranium-thorium dating has yielded ages between 45,760 and 47,160 years.[81] Overall the lake existed between 46,000 and 47,000 years ago.[82] The Inca Huasi cycle coincides with the marine isotope stage 3,[85] the formation of a deep lake in the Laguna Pozuelos basin and the expansion of glaciers in several parts of South America.[86]
This lake cycle took part during a glacial epoch, along with the Sajsi lake cycles.[81] A more humid climate in northeastern Argentina and elsewhere in subtropical South America has been linked to the Inca Huasi phase.[84] However, rainfall might not have increased by much on the Altiplano during the Inca Huasi cycle.[82]
Other paleolakes are Coipasa, Ouki, Minchin, Sajsi, Salinas and Tauca.[81] Research made in 2006 attributed the "Lake Minchin" to this lake phase.[84]
The site was dated to Marine Isotope Stage 3 using Optically Stimulated Luminescence dating of the sand from the two layers of deposits within the channel.[87]
Eruptions occurred at Monte Burney (a volcano in southern Chile, part of its Austral Volcanic Zone which consists of six volcanoes with activity during the Quaternary) during the Pleistocene. Two eruptions around 49,000 ± 500 and 48,000 ± 500 years before present deposited tephra in Laguna Potrok Aike,[88] a lake approximately 300 kilometres (190 mi) east of Monte Burney;[89] there they reach thicknesses of 48 centimetres (19 in) and 8 centimetres (3.1 in) respectively.[88] Other Pleistocene eruptions are recorded there at 26,200 and 31,000 years ago,[90] with additional eruptions having occurred during marine isotope stage 3.[90]
Glinde interstadial[edit | edit source]
The Glinde interstadial has a 14C date of 48-50 kyr B.P. and corresponds to GIS ?13/14 with a GIS age of 49-54.5 kyr B.P.[69]
Ebersdorf Stadial[edit | edit source]
This corresponds to the Skjonghelleren Glaciation of Scandinavia where ice crosses the North Sea between 50-40 ka BP.
Oerel interstadial[edit | edit source]
The Oerel interstadial has a 14C date of 53-58 kyr B.P. and corresponds to GIS 15/16 with a GIS age of 56-59 kyr B.P.[69]
MIS Boundary 3/4 is at 57 ka.[70]
Karmøy stadial[edit | edit source]
The Karmøy stadial begins in the high mountains of Norway about 58 kyr B.P. and expands to the outer coast by 60 kyr B.P.[69]
The Schalkholz Stadial in North Germany is equivalent.
Odderade interstadial[edit | edit source]
The Odderade interstadial has a 14C date of 61-72 kyr B.P. and corresponds to GIS 21.[69]
MIS Boundary 4/5 is at 71 ka.[70]
Marine Isotope Stage 4[edit | edit source]
"The glacial episode of Marine Isotope Stage 4 [was] about 57-71,000 years ago".[91]
Ognon III interstadial[edit | edit source]
Ognon II interstadial[edit | edit source]
"The overlying loess, colluvial sediments and humiferous soils that end the palaeosol succession belong to the Ognon II and III interstadials; they record the onset of the early Pleniglacial (MIS stage 4)".[92]
Wisconsinian glacial[edit | edit source]
Wisconsinian glacial began at 80,000 yr BP.[44]
Rederstall Stadial[edit | edit source]
MIS Boundary 5.1 (Ognon I, peak) is at 82 ka.[70]
MIS Boundary 5.2 (Melisey II, Saint-Germain II, peak) is at 87 ka.[70]
MIS Boundary 5.3 (Saint-Germain I) is at 96 ka.[70]
Brørup interstadial[edit | edit source]
The "Brørup interstade [is about] 100 ka BP".[71] It corresponds to GIS 23/24.[69]
MIS Boundary 5.4 (peak) is at 109 ka.[70]
Herning Stadial[edit | edit source]
MIS Boundary 5.5 (peak) is at 123 ka.[70]
Eemian interglacial[edit | edit source]
The "controversially split Eemian period, the predecessor of our own warm period about 125,000 years ago."[20]
"The Eem interglaciation […] lasted from 131 to 117 kyr B.P."[20]
The Eemian is MIS substage 5e.
Late Pleistocene[edit | edit source]
Late Pleistocene spans ca. 11,000-150,000 yr BP.[44]
MIS Boundary 5/6 is at 130 ka.[70]
Haweran[edit | edit source]
The Haweran began 0.34 Ma and extends to the present.
Sangamon Episode interglacial[edit | edit source]
"OSL dates also suggest that last interglacial (MIS 5; Sangamon Ep.) fluvial deposits are preserved locally."[93]
Age "assignment of Sangamonian (sense alto = 80,000-ca. 220,000 yr BP) [is] to Illinoian (ca. 220,000-430,000 yr BP)".[44]
MIS Boundary 6/7 is at 191 ka.[70]
Middle Pleistocene[edit | edit source]
Middle Pleistocene spans ca. 150,000-730,000 yr BP.[44]
Illinois Episode glaciation[edit | edit source]
"Ages of sediments immediately beneath the oldest till (Kellerville Mbr.) in the bedrock valley average 160 ka and provide direct confirmation that Illinois Episode (IE) glaciation began in its type area during marine isotope stage (MIS) 6. The oldest deposits found are 190 ka fluvial sands on bedrock in the deepest part of the valley. These correlate to earliest MIS 6. We now correlate the lowest deposits to the IE (Pearl Fm.)."[93]
The "last two glacial cycles [span] MIS 6 through 2".[93]
"Illinoian [is] (ca. 220,000-430,000 yr BP)".[44]
MIS Boundary 10/11 is at 374 ka.[70]
MIS Boundary 9/10 is at 337 ka.[70]
MIS Boundary 8/9 is at 300 ka.[70]
MIS Boundary 7/8 is at 243 ka.[70]
Yarmouthian interglacial[edit | edit source]
"Clay deposition in the Piauí River floodplain around 436 ± 51.5 ka occurred during a warmer period of the [Yarmouthian interglaciation] Aftonian interglaciation, corresponding to isotope stage 12 (Ericson and Wollin, 1968)."[94]
Yarmouthian spans 420,000-500,000 yr BP.[44]
MIS Boundary 12/13 is at 478 ka.[70]
MIS Boundary 11/12 is at 424 ka.[70]
Anglian[edit | edit source]
The Anglian Stage is the name used in the British Isles for a middle Pleistocene glaciation that precedes the Hoxnian Stage and follows the Cromerian Stage, is correlated to Marine Isotope Stage 12 (MIS 12),[95][96][97] which started about 478,000 years ago and ended about 424,000 years ago.[70][98]
The Anglian stage has often been correlated to the Elsterian Stage of northern Continental Europe and the Mindel Stage in the Alps; however, there is ambiguity regarding the correlation of these two glacials to either MIS 12 or MIS 10.[99]
The Anglian was the most extreme glaciation during the last 2 million years, where the ice sheet reached the Isles of Scilly and the Western Approaches, the furthest south the ice reached in any Pleistocene ice age.[100] In the south-east of England it diverted the River Thames from its old course through the Vale of St Albans south to its present position.[101]
This stage had been equated to the Kansan Stage in North America; however, the terms Kansan Stage, along with Yarmouth, Nebraskan, and Aftonian stages, have been abandoned by North American Quaternary geologists and merged into the Pre-Illinoian stage.[102][103] The Anglian Stage is now correlated with the period of time which includes the Pre-Illinoian B glaciation of North America.[97][103]
Kansan glacial[edit | edit source]
Kansan glacial spans 500,000-600,000 yr BP.[44]
MIS Boundary 14/15 is at 563 ka.[70]
MIS Boundary 13/14 is at 533 ka.[70]
Aftonian interglacial[edit | edit source]

Aftonian interglacial spans ca. 600,000-650,000 yr BP.[44]
"N tills [...] show the greatest amount of feldspar and carbonate minerals in the silt fraction. This group includes at least one till unit overlain by the 0.6 Ma Lava Creek ash, thus suggesting that some of these units were deposited between 0.8 and 0.6 Ma, but also later, as indicated by two sites with a till overlying the 0.6 Ma ash (Boellstorff, 1973). The N till group is considered to include the A1, A2, and A3 tills of Boellstorff (1973, 1978b)."[104]
Lava Creek B ash is dated at 602 ka.[104]
MIS Boundary 15/16 is at 621 ka.[70]
The Yellowstone Lava Creek B ash is dated at 639 ± 2 ka ka.[105]
"The Lava Creek B ash bed (0.64 Ma) originated from one of several Yellowstone Plateau plinian eruptions that produced extensive ashfall over much of the west-central United States (Izett and Wilcox, 1982)."[105]
"The second, and geochemically analyzed, occurrence of Lava Creek B ash is in Kelso Gulch, along sloping hillsides slightly above the valley floor (Fig. 2). The tephra layer intermittently follows the contour of the hillslope at an elevation of 1,591 m. It is variably cemented with calcite and up to 5 cm thick. At this locality, geochemical confirmation of the Lava Creek B ash by co-author Wan (Table 1) comes from sample K06CO3, collected from an indurated, ca. 5-cm-thick ash bed exposed on a hillside (Fig. 2). This ash bed is thinly mantled by slope-wash."[105]
"Processing, petrographic analysis, and geochemical fingerprinting of tephra sample K06CO3 and its identification as the Lava Creek B ash was performed at the USGS Tephrochronology Laboratory and the Electron Microprobe Laboratory in Menlo Park, California."[105]
"Examples of pre–Illinoian sections [are in the images on the right]. (A) Two till units with paleosols separated by nonglacial silt and clay unit at site 19 (blow-up of units to left). (B) Lava Creek B ash (0.602 Ma) cropping out near site 4. (C) Two-till unit sequence capped by loess deposits at site 15. Lower till is truncated by sand and gravel unit whereas upper till is affected by paleosol development. Sandy diamicton is present between lower till and bedrock."[104]
Chibanian[edit | edit source]
The Chibanin ends with the onset of the Eemian interglacial period (Marine Isotope Stage 5).[46]
The beginning of the Chibanian is the Brunhes–Matuyama reversal, when the Earth's magnetic field last underwent reversal.[106]
Nebraskan glacial[edit | edit source]
Nebraskan glacial spans ca. 650,000-1,000,000 yr BP.[44]
The magnetic field reversal to the present geomagnetic poles (Brunhes chron) occurred at 780,000 yr BP.
"The R1-till group includes two till units that overlie the 1.3 Ma Mesa Falls ash, thus indicating at least two glaciations between 1.3 Ma and 0.8 Ma."[104]
The magnetic field reversal to the opposite geomagnetic poles (subchron) occurred at 900,000 yr BP.
MIS Boundary 27/28 is at 982 ka.[70]
MIS Boundary 26/27 is at 970 ka.[70]
MIS Boundary 25/26 is at 959 ka.[70]
MIS Boundary 24/25 is at 936 ka.[70]
MIS Boundary 23/24 is at 917 ka.[70]
MIS Boundary 22/23 is at 900 ka.[70]
MIS Boundary 21/22 is at 866 ka.[70]
MIS Boundary 20/21 is at 814 ka.[70]
MIS Boundary 19/20 is at 790 ka.[70]
MIS Boundary 18/19 is at 761 ka.[70]
MIS Boundary 17/18 is at 712 ka.[70]
MIS Boundary 16/17 is at 676 ka.[70]
Early Pleistocene[edit | edit source]
Early Pleistocene spans ca. 730,000-1,600,000 yr BP.[44]
Mesa Falls ash is dated at 1293 ka.[104]
Castlecliffian[edit | edit source]
The Castlecliffian began 1.63 Ma and last until 0.34 Ma.
Calabrian[edit | edit source]


The magnetic field reversal to the present geomagnetic poles (Jaramillo subchron) occurred at 1,060,000 yr BP.
The magnetic field reversal to the opposite, back to the present, then opposite geomagnetic poles (Cobb Mountain subchron) occurred at 1,190,000 yr BP.
The magnetic field reversal to the opposite geomagnetic poles (Olduvai subchron) occurred at 1,780,000 yr BP.
"The [Calabrian] GSSP occurs at the base of the marine claystone conformably overlying sapropelic bed ‘e’ within Segment B in the Vrica section. This lithological level represents the primary marker for the recognition of the boundary, and is assigned an astronomical age of 1.80 Ma on the basis of sapropel calibration."[107]
MIS Boundary 63/64 is at 1782 ka.[70]
MIS Boundary 62/63 is at 1758 ka.[70]
MIS Boundary 61/62 is at 1743 ka.[70]
MIS Boundary 60/61 is at 1715 ka.[70]
MIS Boundary 59/60 is at 1697.5 ka.[70]
MIS Boundary 58/59 is at 1670 ka.[70]
MIS Boundary 57/58 is at 1642.5 ka.[70]
MIS Boundary 56/57 is at 1628.5 ka.[70]
In the image on the right, the Vrica section includes specifically the GSSP of the Calabrian Stage fixed at the top of layer 'e'.
Antian[edit | edit source]
The Antian began ~2.12 Ma and ended ~2.0 Ma.
Bramertonian[edit | edit source]
The Bramertonian Stage is the name for an early Pleistocene biostratigraphic stage in the British Isles that precedes the Pre-Pastonian Stage (Baventian Stage), derives its name from Bramerton Pits in Norfolk, where the deposits can be found on the surface, but the exact timing of the beginning and end of the Bramertonian Stage is currently unknown, it is equivalent to the Tiglian C1-4b Stage of Europe and early Pre-Illinoian Stage of North America, and lies somewhere in time between Marine Oxygen Isotope stages 65 to 95 and somewhere between 1.816 and 2.427 Ma.[95][97][70][98] The Bramertonian is correlated with the Antian stage identified from pollen assemblages in the Ludham borehole.[108][109]
Gelasian[edit | edit source]

Some number of N tills occurred during the Olduvai subchron.[104]
The magnetic field reversal to the present geomagnetic poles (Olduvai subchron) occurred at 2,000,000 yr BP.
The oldest till group, R2 tills, consists of till units with a reversed polarity and >77% of sedimentary clasts. Low amounts of expandable clays, substantial amounts of kaolinite, and the absence of chlorite characterize the clay mineralogy of R2 tills. The mineralogy of the silt fraction of R2 tills is rich in quartz and depleted in calcite, dolomite, and feldspar. This till group includes a till unit that underlies the 2.0-Ma Huckleberry Ridge ash, thus indicating deposition sometime between ~2.5 Ma (onset of Northern Hemisphere glaciations) (Mix et al., 1995) and 2.0 Ma.[104]
The magnetic field reversal to the present geomagnetic poles (Reunion subchron) and back occurred at 2,080,000 yr BP.
The magnetic field reversal to the present geomagnetic poles (Reunion subchron) and back occurred at 2,140,000 yr BP.
"The base of the Quaternary System [shown in the image on the right] is defined by the Global Stratotype Section and Point (GSSP) of the Gelasian Stage at Monte San Nicola in Sicily, Italy, currently dated at 2.58 Ma."[110]
"The astrochronological age of sapropel MPRS 250 (mid-point), corresponding to precessional cycle 250 from the present, is 2.588 Ma (Lourens et al., 1996), which can be assumed as the age of the boundary."[111]
MIS Boundary 102/103 is at 2575 ka.[70]
MIS Boundary 101/102 is at 2554 ka.[70]
MIS Boundary 100/101 is at 2540 ka.[70]
MIS Boundary 99/100 is at 2510 ka.[70]
MIS Boundary 98/99 is at 2494 ka.[70]
MIS Boundary 97/98 is at 2477 ka.[70]
MIS Boundary 96/97 is at 2452 ka.[70]
MIS Boundary 95/96 is at 2427 ka.[70]
MIS Boundary 94/95 is at 2407 ka.[70]
MIS Boundary 93/94 is at 2387 ka.[70]
MIS Boundary 92/93 is at 2373 ka.[70]
MIS Boundary 91/92 is at 2350 ka.[70]
MIS Boundary 90/91 is at 2333 ka.[70]
MIS Boundary 89/90 is at 2309 ka.[70]
MIS Boundary 88/89 is at 2291 ka.[70]
MIS Boundary 87/88 is at 2273 ka.[70]
MIS Boundary 86/87 is at 2250 ka.[70]
MIS Boundary 85/86 is at 2236 ka.[70]
MIS Boundary 84/85 is at 2207.5 ka.[70]
MIS Boundary 83/84 is at 2192 ka.[70]
MIS Boundary 82/83 is at 2168 ka.[70]
MIS Boundary 81/82 is at 2146 ka.[70]
MIS Boundary 80/81 is at 2125 ka.[70]
MIS Boundary 79/80 is at 2103 ka.[70]
MIS Boundary 78/79 is at 2088 ka.[70]
MIS Boundary 77/78 is at 2043 ka.[70]
MIS Boundary 76/77 is at 2017 ka.[70]
Huckleberry Ridge ash is dated at 2003 ka.[104]
MIS Boundary 75/76 is at 1990 ka.[70]
MIS Boundary 74/75 is at 1965 ka.[70]
MIS Boundary 73/74 is at 1941 ka.[70]
MIS Boundary 72/73 is at 1915 ka.[70]
MIS Boundary 71/72 is at 1898 ka.[70]
MIS Boundary 70/71 is at 1875 ka.[70]
MIS Boundary 69/70 is at 1859.5 ka.[70]
MIS Boundary 68/69 is at 1849 ka.[70]
MIS Boundary 67/68 is at 1832.5 ka.[70]
MIS Boundary 66/67 is at 1826 ka.[70]
MIS Boundary 65/66 is at 1816 ka.[70]
MIS Boundary 64/65 is at 1802.5 ka.[70]
Amstelian[edit | edit source]
The Amstelian began 2.588 Ma and ended 2.40 Ma.
Aquatraversian[edit | edit source]
The Aquatraversian began 2.588 Ma and ended 2.4 Ma.
Mangapanian[edit | edit source]
The Mangapanian began 3.00 Ma and ended 2.40 Ma.
Nukumaruan[edit | edit source]
The Nukumaruan began 3.1 Ma and ended 1.8 Ma.
Tertiary[edit | edit source]
The Tertiary Period extends from 65.5 ± 0.3 to 2.588 x 106 b2k.
Neogene[edit | edit source]
The Neogene dates from 23.03 x 106 to 2.58 x 106 b2k.
Pliocene[edit | edit source]
The Pliocene ranges from 5.332 x 106 to 2.588 x 106 b2k.
"The base of the Quaternary System is defined by the Global Stratotype Section and Point (GSSP) of the Gelasian Stage at Monte San Nicola in Sicily, Italy, currently dated at 2.58 Ma."[48]
Piacenzian[edit | edit source]

The magnetic field reversal to the present geomagnetic poles (Matuyama chron) occurred at 2,590,000 yr BP.
MIS Boundary MG12/Gi1 is at 3592 ka.[70]
MIS Boundary MG11/MG12 is at 3578 ka.[70]
MIS Boundary MG10/MG11 is at 3566 ka.[70]
MIS Boundary MG9/MG10 is at 3546 ka.[70]
MIS Boundary MG8/MG9 is at 3532 ka.[70]
MIS Boundary MG7/MG8 is at 3517 ka.[70]
MIS Boundary MG6/MG7 is at 3471 ka.[70]
MIS Boundary MG5/MG6 is at 3444 ka.[70]
MIS Boundary MG4/MG5 is at 3387 ka.[70]
MIS Boundary MG3/MG4 is at 3372 ka.[70]
MIS Boundary MG2/MG3 is at 3347 ka.[70]
MIS Boundary MG1/MG2 is at 3332 ka.[70]
MIS Boundary M2/MG1 is at 3312 ka.[70]
MIS Boundary M1/M2 is at 3264 ka.[70]
MIS Boundary KM6/M1 is at 3238 ka.[70]
MIS Boundary KM5/KM6 is at 3212 ka.[70]
MIS Boundary KM4/KM5 is at 3184 ka.[70]
MIS Boundary KM3/KM4 is at 3167 ka.[70]
MIS Boundary KM2/KM3 is at 3150 ka.[70]
MIS Boundary KM1/KM2 is at 3119 ka.[70]
MIS Boundary K2/KM1 is at 3097 ka.[70]
MIS Boundary K1/K2 is at 3087 ka.[70]
MIS Boundary G22/K1 is at 3055 ka.[70]
MIS Boundary G21/G22 is at 3039 ka.[70]
MIS Boundary G20/6G21 is at 3025 ka.[70]
MIS Boundary G19/G20 is at 2999 ka.[70]
MIS Boundary G18/G19 is at 2982.5 ka.[70]
MIS Boundary G17/G18 is at 2966 ka.[70]
MIS Boundary G16/G17 is at 2937 ka.[70]
MIS Boundary G15/G16 is at 2913 ka.[70]
MIS Boundary G14/G15 is at 2893 ka.[70]
MIS Boundary G13/G14 is at 2876 ka.[70]
MIS Boundary G12/G13 is at 2858 ka.[70]
MIS Boundary G11/G12 is at 2838 ka.[70]
MIS Boundary G10/G11 is at 2820 ka.[70]
MIS Boundary G9/G10 is at 2798 ka.[70]
MIS Boundary G8/G9 is at 2777 ka.[70]
MIS Boundary G7/G8 is at 2759 ka.[70]
MIS Boundary G6/G7 is at 2730 ka.[70]
MIS Boundary G5/G6 is at 2704 ka.[70]
MIS Boundary G4/G5 is at 2690 ka.[70]
MIS Boundary G3/G4 is at 2681 ka.[70]
MIS Boundary G2/G3 is at 2652 ka.[70]
MIS Boundary G1/G2 is at 2638 ka.[70]
MIS Boundary 104/G1 is at 2614 ka.[70]
MIS Boundary 103/105 is at 2595 ka.[70]
"The base of the beige marl bed of the small-scale carbonate cycle 77 (sensu Hilgen, 1991b) is the approved base of the Piacenzian Stage (that is the Lower Pliocene-Middle Pliocene boundary). It corresponds to precessional excursion 347 as numbered from the present with an astrochronological age estimate of 3.600 Ma (Lourens et al., 1996a)."[112]
Waipipian[edit | edit source]
The Waipipian began 3.60 Ma and lasted to 3.00 Ma.
Opoitian[edit | edit source]
The Opoitian began 5.0 Ma and ended 3.8 Ma.
Wanganui[edit | edit source]
The Wanganui epoch began 5.33-5.28 Ma and extends into the present.
Zanclean[edit | edit source]

"The boundary-stratotype of the stage is located in the Eraclea Minoa section on the southern coast of Sicily (Italy), at the base of the Trubi Formation. The age of the Zanclean and Pliocene GSSP at the base of the stage is 5.33 Ma in the orbitally calibrated time scale, and lies within the lowermost reversed episode of the Gilbert Chron (C3n.4r), below the Thvera normal subchron."[113]
In the chronostratigraphic correlation in the Piacenzian section, the base of the Zanclean is marked as the '0' point.
MIS Boundary TG5/TG6 is at 5315 ka.[70]
MIS Boundary TG4/TG5 is at 5301 ka.[70]
MIS Boundary TG3/TG4 is at 5289 ka.[70]
MIS Boundary TG2/TG3 is at 5266 ka.[70]
MIS Boundary TG1/TG2 is at 5241 ka.[70]
MIS Boundary T8/TG1 is at 5188 ka.[70]
MIS Boundary T7/T8 is at 5165 ka.[70]
MIS Boundary T6/T7 is at 5116 ka.[70]
MIS Boundary T5/T6 is at 5094 ka.[70]
MIS Boundary T4/T5 is at 5070 ka.[70]
MIS Boundary T3/T4 is at 5038 ka.[70]
MIS Boundary T2/T3 is at 5016 ka.[70]
MIS Boundary T1/T2 is at 5002 ka.[70]
MIS Boundary ST4/T1 is at 4985 ka.[70]
MIS Boundary ST3/ST4 is at 4976 ka.[70]
MIS Boundary ST2/ST3 is at 4952.5 ka.[70]
MIS Boundary ST1/ST2 is at 4931 ka.[70]
MIS Boundary Si6/ST1 is at 4904 ka.[70]
MIS Boundary Si5/Si6 is at 4883 ka.[70]
MIS Boundary Si4/Si5 is at 4860 ka.[70]
MIS Boundary Si3/Si4 is at 4840 ka.[70]
MIS Boundary Si2/Si3 is at 4821 ka.[70]
MIS Boundary Si1/Si2 is at 4807 ka.[70]
MIS Boundary NS6/Si1 is at 4778 ka.[70]
MIS Boundary NS5/NS6 is at 4766 ka.[70]
MIS Boundary NS4/NS5 is at 4737 ka.[70]
MIS Boundary NS3/NS4 is at 4722.5 ka.[70]
MIS Boundary NS2/NS3 is at 4702.5 ka.[70]
MIS Boundary NS1/NS2 is at 4684 ka.[70]
MIS Boundary N10/NS1 is at 4658 ka.[70]
MIS Boundary N9/N10 is at 4648 ka.[70]
MIS Boundary N8/N9 is at 4622 ka.[70]
MIS Boundary N7/N8 is at 4603 ka.[70]
MIS Boundary N6/N7 is at 45887 ka.[70]
MIS Boundary N5/N6 is at 4570 ka.[70]
MIS Boundary N4/N5 is at 4538 ka.[70]
MIS Boundary N3/N4 is at 4523 ka.[70]
MIS Boundary N2/N3 is at 4508 ka.[70]
MIS Boundary N1/N2 is at 4487 ka.[70]
MIS Boundary CN8/N1 is at 4457 ka.[70]
MIS Boundary CN7/CN8 is at 4446 ka.[70]
MIS Boundary CN6/CN7 is at 4420 ka.[70]
MIS Boundary CN5/CN6 is at 4395 ka.[70]
MIS Boundary CN4/CN5 is at 4371 ka.[70]
MIS Boundary CN3/CN4 is at 4356 ka.[70]
MIS Boundary CN2/CN3 is at 4335 ka.[70]
MIS Boundary CN1/CN2 is at 4327 ka.[70]
MIS Boundary Co4/CN1 is at 4303 ka.[70]
MIS Boundary Co3/Co4 is at 4286 ka.[70]
MIS Boundary Co2/Co3 is at 4259 ka.[70]
MIS Boundary Co1/Co2 is at 4232 ka.[70]
MIS Boundary Gi28/Co1 is at 4211 ka.[70]
MIS Boundary Gi27/Gi28 is at 4192 ka.[70]
MIS Boundary Gi26/Gi27 is at 4175 ka.[70]
MIS Boundary Gi25/Gi26 is at 4146 ka.[70]
MIS Boundary Gi24/Gi25 is at 4098 ka.[70]
MIS Boundary Gi23/Gi24 is at 4085 ka.[70]
MIS Boundary Gi22/Gi23 is at 4048 ka.[70]
MIS Boundary Gi21/Gi22 is at 4029 ka.[70]
MIS Boundary Gi20/Gi21 is at 4007 ka.[70]
MIS Boundary Gi19/Gi20 is at 3978 ka.[70]
MIS Boundary Gi18/Gi19 is at 3952 ka.[70]
MIS Boundary Gi17/Gi18 is at 3939 ka.[70]
MIS Boundary Gi16/Gi17 is at 3923 ka.[70]
MIS Boundary Gi15/Gi16 is at 3912 ka.[70]
MIS Boundary Gi14/Gi15 is at 3879 ka.[70]
MIS Boundary Gi13/Gi14 is at 3862 ka.[70]
MIS Boundary Gi12/Gi13 is at 3835 ka.[70]
MIS Boundary Gi11/Gi12 is at 3822 ka.[70]
MIS Boundary Gi10/Gi11 is at 3798 ka.[70]
MIS Boundary Gi9/Gi10 is at 3768 ka.[70]
MIS Boundary Gi8/Gi9 is at 3752 ka.[70]
MIS Boundary Gi7/Gi8 is at 3742 ka.[70]
MIS Boundary Gi6/Gi7 is at 3719 ka.[70]
MIS Boundary Gi5/Gi6 is at 3705 ka.[70]
MIS Boundary Gi4/Gi5 is at 3676 ka.[70]
MIS Boundary Gi3/Gi4 is at 3660 ka.[70]
MIS Boundary Gi2/Gi3 is at 3637 ka.[70]
MIS Boundary Gi1/Gi2 is at 3619 ka.[70]
Kapitean[edit | edit source]
The Kapitean began 6.5 Ma and ended 5.0 Ma.
Miocene[edit | edit source]
The Miocene dates from 23.03 x 106 to 5.332 x 106 b2k.
Messinian[edit | edit source]
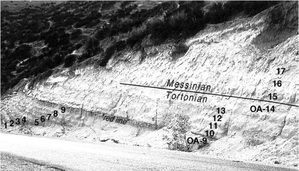

"The GSSP of the Messinian Stage, which per definition marks the base of the Messinian and, hence, the boundary between the Tortonian and Messinian Stages of the Upper Miocene Subseries, is Oued Akrech (Morocco) where the Messinian GSSP is now formally designated at the base of the reddish layer of sedimentary cycle no. 15. This point coincides closely with the first regular occurrence (FRO) of the planktonic foraminiferal Globorotalia miotumida group and the first occurrence (FO) of the calcareous nannofossil Amaurolithus delicatus, and falls within the interval of reversed polarity that corresponds to C3Br.1r. The base of the reddish layer and, thus, the Messinian GSSP has been assigned an astronomical age of 7.251 Ma."[114]
"The correlation of characteristic sedimentary cycle patterns to the astronomical record resulted in an astronomical age of 7.24 Ma (Hilgen et al., 1995), in good agreement with the radiometric age estimates of Vai et al. (1993) and Laurenzi et al. (1997)."[114]
The integrated magnetostratigraphy, calcareous plankton biostratigraphy and cyclostratigraphy of section Oued Akrech is diagrammed on the left.
Taranaki[edit | edit source]
The Taranaki began 10.9 Ma and ended 5.28 Ma in New Zealand.
Tortonian[edit | edit source]
The Tortonian lasted from 11.63 Ma to 7.246 Ma.
Middle Miocene[edit | edit source]
The sub-epoch lasted from 15.97 ± 0.05 Ma to 11.608 ± 0.005 Ma (million years ago). During this period, a sharp drop in global temperatures took place. This event is known as the Middle Miocene Climate Transition.
Serravallian[edit | edit source]
The Serravallian is in the middle Miocene and spans the time between 13.82 Ma and 11.63 Ma (million years ago), follows the Langhian and is followed by the Tortonian.[115]
Bairnsdalian[edit | edit source]
The Bairnsdalian began 15.0 Ma and ended 10.5 Ma.
Lillburnian[edit | edit source]
The Lillburnian began 15.1 Ma and ended 12.7 Ma.
Clifdenian[edit | edit source]
The Clifdenian began 15.9 Ma and lasted to 15.1 Ma.
Southland[edit | edit source]
The Southland began 15.9 Ma and lasted to 10.9 Ma.
Langhian[edit | edit source]
The Langhian is, in the International Commission on Stratigraphy (ICS) geologic timescale, an age or stage in the middle Miocene series, spanning the time between 15.97 ± 0.05 Ma and 13.65 ± 0.05 Ma.[116]
The Langhian was a continuing warming period.[117] defined by Lorenzo Pareto in 1865, it was originally established in the Langhe area north of Ceva in northern Italy, hence the name. The Langhian is preceded by the Burdigalian and followed by the Serravallian stage.
Astaracian[edit | edit source]
The Astaracian age is a period of geologic time (16.0-11.6 Ma), equivalent with the Middle Miocene and used more specifically with European Land Mammal Ages, precedes the Vallesian age and follows the Orleanian age, and overlaps the Langhian and Serravallian ages.[118]
Badenian[edit | edit source]
The Badenian began 16.3 Ma and ended 12.8 Ma.[119]
Antwerpian[edit | edit source]
"The deposits indicated with the stage Antwerpian are nowadays called the Berchem Formation, which is subdivided in the Edegem Sands, Kiel Sands and Antwerpen Sands members (De Meuter & Laga, 1976). Recent micropalaeontological studies revealed the presence of a well-preserved dinoflagellate assemblage: a (latest Aquitanian?) Burdigalian to middle Serravallian age is attributed to the Berchem Formation (Louwye et al., 2000)."[120]
"De Heinzelin (1955) suggested a late Middle Miocene age for the Antwerpian and an early Middle Miocene age for the Bolderian (= “Houthaléen”)."[120]
"The new Sr-dating of dinoflagellate cyst bioevents [...] allows to constrain the age of the Edegem Member [Houthalen Member] between 19 Ma and 18.4 Ma (early Burdigalian)."[121]
Orleanian[edit | edit source]
The Orleanian age is a period of geologic time, MN (Mammal Neogene) zonation (MN) 3–5, 20.0 - 16.0 Ma, within the Miocene and used more specifically with European Land Mammal Ages, preceding the Astaracian age and follows the Agenian age.[122]
Awamoan[edit | edit source]
The Awamoan began 20.0 Ma and ended at 17.5 Ma, occurred during the Miocene of New Zealand.
Burdigalian[edit | edit source]
The Burdigalian is, in the geologic timescale, an age or stage in the early Miocene that spans the time between 20.43 ± 0.05 Ma and 15.97 ± 0.05 Ma, preceded by the Aquitanian, the Burdigalian was the first and longest warming period of the Miocene[123] and is succeeded by the Langhian.
Otaian[edit | edit source]
The Otaian began 21.7 Ma and ended 19.1 Ma.
Pareora[edit | edit source]
The Pareora began 21.7 Ma and ended 15.9 Ma.
Bolderian[edit | edit source]
The Elsloo gravel dates from 23.03 Ma to 19 Ma of the Houthalen Member based on the presence of reworked fossils and the Houthalen Member above. "As is the case for the Edegem Member, the Kiel Member contains glauconite which, based on radiometric dating and grain-size distribution curves, are presumed to be reworked (Odin et al., 1974; Vandenberghe et al., 2014; Adriaens, 2015). The radiometric datings further show divergence between K-Ar ages (23 to 25.3 Ma; Chattian) and Rb-Sr ages (30 Ma; Rupelian) (Odin & Kreuzer, 1988)."[121]
Aquitanian[edit | edit source]
The Aquitanian is, in the International Commission on Stratigraphy (ICS) geologic timescale, the oldest age or lowest stage in the Miocene, that spans the time between 23.03 ± 0.05 Ma and 20.43 ± 0.05 Ma during the Early Miocene, a dry, cooling period.[123]
Paleogene[edit | edit source]
The Paleogene Period extends from 65.5 ± 0.3 to 23.03 ± 0.05 x 106 b2k.[124]
Agenian[edit | edit source]
The Agenian age is a period of geologic time (23.8–20 Ma) within the Miocene used more specifically with European Land Mammal Ages, and follows the Orleanian age and overlaps the Aquitanian and Burdigalian ages.[125]
Oligocene[edit | edit source]
The Oligocene dates from 33.9 ± 0.1 x 106 to 23.03 x 106 b2k.
Waitakian[edit | edit source]
The Waitakian began 25.2 Ma and ended 21.7 Ma.
Duntroonian[edit | edit source]
The Duntroonian began 27.3 Ma and ended 25.2 Ma.
Chattian[edit | edit source]
The Chattian began 27.82 Ma and ended 23.03 Ma.[126]
During the Chattian the largest known single-event volcanic eruption occurred: the Fish Canyon Tuff (Fish Canyon eruption) of the La Garita Caldera with a magnitude of 9.2 and VEI of 8.[127]
It has been dated to 27.51 Ma ago.[128]
Avernian[edit | edit source]
The Avernian began 29.2 Ma and ended 23.03 Ma.
Arikareean[edit | edit source]
The Arikareean North American Stage on the geologic timescale is the North American faunal stage according to the North American Land Mammal Ages chronology (NALMA), typically set from 30,600,000 to 20,800,000 years BP, a period of 30.6-20.8 Ma.[129] It is usually considered to overlap the Oligocene and Miocene epochs. The Arikareean is preceded by the Whitneyan and followed by the Hemingfordian NALMA stages.
The Arikareean can be further divided into the substages of:
- late Late Arikareean: Lower boundary source, base of Geringian (approximate)[130]
- early Late Arikareean: base of Geringian (approximate). Upper boundary source: base of Hemingfordian (approximate).
- late Early Arikareean: Lower boundary source of base of Geringian (approximate). Upper boundary source of base of Hemingfordian (approximate).
- early Early Arikareean (shares lower boundary): Upper boundary source of base of Hemingfordian (approximate).
Rupelian[edit | edit source]
The Rupelian began 33.9 Ma and ended 27.82 Ma.[131]
Eocene[edit | edit source]
The Eocene dates from 55.8 ± 0.2 x 106 to 33.9 ± 0.1 x 106 b2k.
The Eocene is conventionally divided into early (56–47.8 million years ago), middle (47.8–38m), and late (38–33.9m) subdivisions.[132] The corresponding rocks are referred to as lower, middle, and upper Eocene. The Ypresian Stage constitutes the lower, the Priabonian Stage the upper; and the Lutetian and Bartonian Stages are united as the middle Eocene.
Whaingaroan[edit | edit source]
The Whaingaroan began 34.3 Ma and ended 27.3 Ma.
Landon[edit | edit source]
The Landon began 34.3 Ma and ended 21.7 Ma.
Refugian[edit | edit source]
The Refugian began 35.0 Ma and ended 33.5 Ma.
Aldingan[edit | edit source]
The Aldingan began 36 Ma and ended 30 Ma.
Runangan[edit | edit source]
The Runangan began 36.0 Ma and ended 34.3 Ma.
Jacksonian[edit | edit source]

"Por primera vez en la Cuenca de Burgos se utilizan isótopos 87
Sr/86
Sr para obtener una edad geocronológica de 36.75 (+2.65/-2.05) Ma, para la Formación Jackson inferior."[4] In English, "For the first time in the Burgos Basin, 87
Sr/86
Sr isotopes are used to obtain a geochronological age of Ma, for the lower Jackson Formation."[4]
Priabonian[edit | edit source]
The Priabonian began 37.8 Ma and ended 33.9 Ma.[133]
Bartonian[edit | edit source]
The Bartonian began 41.2 Ma and ended 37.8 Ma.[134]
Bortonian[edit | edit source]
The Bortonian began 43.0 Ma and ended 37.0 Ma.
Arnold[edit | edit source]
The Arnold Epoch began 43.0 Ma and ended 34.3 Ma.
Porangan[edit | edit source]
The Porangan began 46.2 Ma and ended 43.0 Ma.
Lutetian[edit | edit source]
The Lutetian began 47.8 Ma and ended 41.2 Ma.[135]
Johannian[edit | edit source]
The Johannian began 48 Ma and ended 35 Ma.
Nanzian[edit | edit source]
The Nanzian began 48 Ma and ended 35 Ma.
Arshantan[edit | edit source]
The Arshantan age is a period of geologic time (48.6–37.2 Ma) within the Early Eocene epoch of the Paleogene used more specifically with Asian Land Mammal Ages, following the Bumbanian age and preceding the Irdinmanhan age, where the upper boundary layer of the Arshantan can be the lower boundary of the Priabonian[136]
Mangaorapan[edit | edit source]
The Mangaorapan began 53.0 Ma and ended 49.5 Ma.
Waipawan[edit | edit source]
The Waipawan began 55.5 Ma and ended 53.0 Ma.
Ypresian[edit | edit source]
The Ypresian began 56.0 Ma and ended 47.8 Ma.[137]
Paleocene[edit | edit source]
The Paleocene dates from 65.5 ± 0.3 x 106 to 55.8 ± 0.2 x 106 b2k.
Thanetian[edit | edit source]
The Thanetian began 59.2 Ma and ended 56.0 Ma.[138]
Selandian[edit | edit source]
The Selandian began 61.6 Ma and ended at 59.2 Ma.[139]
Dannevirke[edit | edit source]
The Dannevirke began 65.0 Ma and ended 43.0 Ma.
Teurian[edit | edit source]
The Teurian began 65.0 Ma or 65.5 Ma and ended 55.5 Ma.
Danian[edit | edit source]


The Danian is the oldest age or lowest stage of the Paleocene Epoch or Series, of the Paleogene Period or System, and of the Cenozoic Era or Erathem: the beginning of the Danian Age (and the end of the preceding Maastrichtian Age) is at the Cretaceous–Paleogene extinction event 66 Ma, ending 61.6 Ma, being followed by the Selandian Age.[140]
"Many correlation criteria are present at the GSSP of which the most useful are the meteorite impact evidence (iridium anomaly, Ni-rich spinel, etc.) and the mass extinction of plankic micro- and nannofossils."[141]
The "GSSP of the K/Pg boundary [is defined] at the base of the boundary clay at the section near El Kef, Tunisia."[141]
"The section [specifically shown in a closeup on the right] contains marine sediments and sedimentation was as continuous as it could be at a K/Pg boundary. There is a facies change from a grey marl to a black clay (Boundary Clay), at the base of which is a thin rusty layer. This is the fingerprint of continuous sedimentation over the K/Pg boundary interval."[141]
"Neither magnetostratigraphy nor geochronometry are available at the section near El Kef."[141]
"The GSSP section near El Kef contains one main feature that allows for a direct correlation of this marine section with continental sections: the Ir anomaly at the base of the Boundary Clay."[141]
The Global Boundary Stratotype Section and Point for the base of the Danian Stage is also the base GSSP for the Paleocene, Paleogene, "Tertiary", and Cenozoic at El Kef, Tunisia.
Hypotheses[edit | edit source]
- Each time frame or span of time in the geochronology of the Cenozoic has at least one dating technique.
See also[edit | edit source]
- Genetics/Ammonoids
- Genetics/Human DNA
- Genetics/Human teeth
- Geochronology/Archaeology
- Geochronology/Cenozoic
- Geochronology/Dates
- Geochronology/Dendrochronology
- Geochronology/Dye 3
- Geochronology/Geomagnetic Polarity Time Scale
- Geochronology/Ice cores
- Geochronology/Ice cores/Black ice
- Geochronology/Ice cores/Brittle ice
- Geochronology/Ice cores/Clear ice
- Geochronology/Ice cores/Firns
- Geochronology/Ice cores/Sea ice
- Geochronology/Medieval Warm Period
- Geochronology/Mesozoic
- Geochronology/Middle Ages
- Geochronology/Neoglaciations
- Geochronology/Optically stimulated luminescence
- Geochronology/Paleontology
- Geochronology/Paleozoic
- Geochronology/Palynology
- Geochronology/Precambrian
- Geochronology/Radiocarbon dating
- Geochronology/Stratigraphy
- Geochronology/Tephra layers
- Geochronology/Uranium-thorium dating
- Paleanthropology
References[edit | edit source]
- ↑ 1.0 1.1 1.2 1.3 Mike Walker; Sigfus Johnsen; Sune Olander Rasmussen; Trevor Popp; Jørgen-Peder Steffensen; Phil Gibbard; Wim Hoek; John Lowe et al. (2009). "Formal definition and dating of the GSSP (Global Stratotype Section and Point) for the base of the Holocene using the Greenland NGRIP ice core, and selected auxiliary records". Journal of Quaternary Science 24 (1): 3-17. doi:10.1002/jqs.1227. http://www.stratigraphy.org/GSSP/Holocene.pdf. Retrieved 2015-01-18.
- ↑ Names from local versions of the geologic timescale can often be found in the local language. The English name is usually found by replacing the suffix in the local language for -an or -ian. Examples for "local" suffices are -en (French), -ano (Spanish), -ium (German), -aidd (Welsh) or -aan (Flemish Dutch). The English name "Norian", for example, becomes Noriano in Spanish, Norium in German, Noraidd in Welsh or Norien in French.
- ↑ 3.0 3.1 Time is given in Megaannum (million years BP, unless other units are given in the table. BP stands for "years before present". For ICS-units the absolute ages are taken from Gradstein et al. (2004).
- ↑ 4.0 4.1 4.2 Eguiluz y de Antuñano, Samuel, Solís Pichardo, Gabriela, Vega, Francisco J. (2020). "Contribución al conocimiento paleontológico, geocronológico y paleoambiental de la Formación Jackson inferior (Eoceno Superior), en la Cuenca de Burgos, Tamaulipas, México". Paleontología Mexicana 9 (2): 121-134. http://www.ojs-igl.unam.mx/index.php/Paleontologia/article/viewFile/642/563. Retrieved 18 October 2021.
- ↑ The status of the Tertiary is not yet decided. The ICS will probably make a decision in 2009.
- ↑ 6.0 6.1 William A. Berggren; Dennis V. Kent; John J. Flynn; John A. Van Couvering (November 1985). "Cenozoic geochronology". Geological Society of America Bulletin 96 (11): 1407-18. doi:10.1130/0016-7606(1985)96<1407:CG>2.0.CO;2. http://academiccommons.columbia.edu/download/fedora_content/download/ac:143432/CONTENT/gsab.96.11.1407.full.pdf. Retrieved 2015-09-16.
- ↑ 7.0 7.1 7.2 Cohen, K.M.; Finney, S.C.; Gibbard, P.L.; Fan, J.-X. "International Chronostratigraphic Chart 2013" (PDF). stratigraphy.org. ICS. Retrieved 15 June 2014.
- ↑ Earthquake Glossary - Late Quaternary U.S. Geological Survey
- ↑ Denton, G.H.; Anderson, R.F.; Toggweiler, J.R.; Edwards, R.L.; Schaefer, J.M.; Putnam, A.E. (2010). "The Last Glacial Termination". Science 328 (5986): 1652–1656. doi:10.1126/science.1184119. PMID 20576882.
- ↑ Lowe, J.J.; Walker, M.J.C. (1997). Reconstructing Quaternary Environments. Routledge. ISBN 978-0582101661.
- ↑ See the 2009 version of the ICS geologic time scale
- ↑ Zalasiewicz, J.; Williams, M.; Haywood, A.; Ellis, M. (2011). "The Anthropocene: a new epoch of geological time?". Philosophical Transactions of the Royal Society A 369 (1938): 835–841. doi:10.1098/rsta.2010.0339. PMID 21282149. http://nora.nerc.ac.uk/id/eprint/14867/1/Anthropocene_overview.pdf.
- ↑ "Working Group on the 'Anthropocene'". Subcomission on Quaternary Stratigraphy. Retrieved 16 June 2014.
- ↑ Ghosh, Pallab (March 4, 2015). "'First human' discovered in Ethiopia". BBC News. London. Retrieved 2015-04-19.
- ↑ Ryan, William B.F.; Pitman, Walter C.; Major, Candace O.; Shimkus, Kazimieras; Moskalenko, Vladamir; Jones, Glenn A.; Dimitrov, Petko; Gorür, Naci et al. (April 1997). "An abrupt drowning of the Black Sea shelf". Marine Geology 138 (1–2): 119–126. doi:10.1016/s0025-3227(97)00007-8.
- ↑ Balbas, A.M., Barth, A.M., Clark, P.U., Clark, J., Caffee, M., O'Connor, J., Baker, V.R., Konrad, K. and Bjornstad, B., 2017. 10Be dating of late Pleistocene megafloods and Cordilleran Ice Sheet retreat in the northwestern United States. Geology, 45(7), pp. 583-586.
- ↑ Dyke, Arthur S. (2004). "An outline of North American deglaciation with emphasis on central and northern Canada". Developments in Quaternary Sciences 2: 373–424. doi:10.1016/S1571-0866(04)80209-4.
- ↑ Haynes. "Stanford Camelops" (PDF).
{{cite web}}
:|archive-date=
requires|archive-url=
(help) - ↑ "Extinct American Cheetah Fact Sheet". Retrieved 2015-12-10.
{{cite web}}
:|archive-date=
requires|archive-url=
(help) - ↑ 20.0 20.1 20.2 20.3 Willi Dansgaard (2005). The Department of Geophysics of The Niels Bohr Institute for Astronomy Physics and Geophysics at The University of Copenhagen Denmark. ed. Frozen Annals Greenland Ice Cap Research. Copenhagen, Denmark: Niels Bohr Institute. pp. 123. ISBN 87-990078-0-0. http://www.iceandclimate.nbi.ku.dk/publications/FrozenAnnals.pdf/. Retrieved 2014-10-05.
- ↑ 21.0 21.1 Cohen, K. M.; Finney, S. C.; Gibbard, P. L.; Fan, J-X. (January 2020). "International Chronostratigraphic Chart" (PDF). International Commission on Stratigraphy. Retrieved 8 January 2021.
- ↑ Radha-Udayakumar, Ganesh (19 July 2018). "Scientists call our era the Meghalayan Age. Here's what the world was like when it began". India Today. New Delhi: Living Media India Limited. Retrieved 3 January 2021.
- ↑ Scroll Staff. "'Meghalayan Age': Latest phase in Earth's history named after Indian state, began 4,200 years ago". Scroll.in.
- ↑ International Commission on Stratigraphy. "ICS chart containing the Quaternary and Cambrian GSSPs and new stages (v 2018/07) is now released!". Retrieved 15 July 2018.
{{cite web}}
:|archive-date=
requires|archive-url=
(help) - ↑ "'Meghalayan Age' makes the state a part of geologic history". Hindustan Times. 18 July 2018. Retrieved 26 July 2018.
- ↑ "Formal subdivision of the Holocene Series/Epoch" (PDF).
- ↑ 27.0 27.1 Jonathan Amos (18 July 2018). "Welcome to the Meghalayan Age – a new phase in history". BBC. Retrieved 19 July 2018.
- ↑ International Commission on Stratigraphy. "Collapse of civilizations worldwide defines youngest unit of the Geologic Time Scale". News and Meetings. Retrieved 15 July 2018.
- ↑ 29.0 29.1 Michael Irving (19 July 2018). "Time for the Meghalayan: A new geological age has officially been declared". Retrieved 19 July 2018.
{{cite web}}
:|archive-date=
requires|archive-url=
(help) - ↑ "Exact dating of the Meghalayan lower boundary based on high-latitude tree-ring isotope chronology". Quaternary Science Reviews 214: 178–184. 2019-06-15. doi:10.1016/j.quascirev.2019.04.013. ISSN 0277-3791. https://www.sciencedirect.com/science/article/abs/pii/S0277379118310345.
- ↑ Cohen, K. M.; Finney, S. C.; Gibbard, P. L.; Fan, J-X. (January 2020). "International Chronostratigraphic Chart" (PDF). International Commission on Stratigraphy. Retrieved 8 January 2021.
- ↑ 32.0 32.1 International Commission on Stratigraphy. "ICS chart containing the Quaternary and Cambrian GSSPs and new stages (v 2018/07) is now released!". Retrieved 19 July 2018.
- ↑ Radha-Udayakumar, Ganesh (19 July 2018). "Scientists call our era the Meghalayan Age. Here's what the world was like when it began". India Today. New Delhi: Living Media India Limited. Retrieved 3 January 2021.
- ↑ Scroll Staff. "'Meghalayan Age': Latest phase in Earth's history named after Indian state, began 4,200 years ago". Scroll.in.
- ↑ Amos, Jonathan (18 July 2018). "Welcome to the Meghalayan Age – a new phase in history". BBC News. Retrieved 19 July 2018.
- ↑ 36.0 36.1 A. Speranza; J. van der Plicht; B. van Geel (November 2000). "Improving the time control of the Subboreal/Subatlantic transition in a Czech peat sequence by 14C wiggle-matching". Quaternary Science Reviews 19 (16): 1589-1604. doi:10.1016/S0277-3791(99)00108-0. http://www.researchgate.net/publication/30494985_Improving_the_time_control_of_the_SubborealSubatlantic_transition_in_a_Czech_peat_sequence_by_14C_wiggle-matching/file/60b7d51c350cf2efa0.pdf. Retrieved 2014-11-04.
- ↑ 37.0 37.1 E.B. Karabanov; A.A. Prokopenko; D.F. Williams; G.K. Khursevich (March 2000). "A new record of Holocene climate change from the bottom sediments of Lake Baikal". Palaeogeography, Palaeoclimatology, Palaeoecology 156 (3-4): 211–24. doi:10.1016/S0031-0182(99)00141-8. http://www.sciencedirect.com/science/article/pii/S0031018299001418. Retrieved 2014-11-04.
- ↑ Barber E. (1991) "Prehistoric Textiles: The Development of Cloth in the Neolithic and Bronze Ages with Special Reference to the Aegean". Princeton University Press, p.12
- ↑ Cullis, Chris A. (2007). "Flax". In Kole, Chittaranjan. Oilseeds. Springer. p. 275.
- ↑ S.O. Rasmussen, B.M. Vinther, H.B. Clausen, and K.K. Andersen. Greenland Ice Core Chronology 2005 (GICC05) Early Holocene section. IGBP PAGES/World Data Center for Paleoclimatology Data Contribution Series # 2006-119. NOAA/NCDC Paleoclimatology Program, Boulder CO, USA.
- ↑ 41.0 41.1 Fu, Y.-B. (2011). "Genetic evidence for early flax domestication with capsular dehiscence". Genetic Resources and Crop Evolution 58 (8): 1119–1128. doi:10.1007/s10722-010-9650-9.
- ↑ Çatalhöyük 2013 Archive Report (Report).
- ↑ 43.0 43.1 43.2 Bernd Kromer; Bernd Becker (1993). "German Oak and Pine 14C Calibration, 7200-9439 BC". Radiocarbon 35 (1): 125-135. https://journals.uair.arizona.edu/index.php/radiocarbon/article/download/18069/17799#page=130. Retrieved 2017-10-13.
- ↑ 44.00 44.01 44.02 44.03 44.04 44.05 44.06 44.07 44.08 44.09 44.10 Sam L. VanLandingham (May 2010). "Use of diatoms in determining age and paleoenvironment of the Valsequillo (Hueyatiaco) early man site, Puebla, Mexsico, with corroboration by Chrysophyta cysts for a maximum Yarmouthian (430,000-500,00yr BP) age of the artifacts". Nova Hedwigia 136: 127-38. http://www.pleistocenecoalition.com/vanlandingham/VanLandingham_2010b.pdf. Retrieved 2017-06-11.
- ↑ 45.0 45.1 Cohen, K. M.; Finney, S. C.; Gibbard, P. L.; Fan, J-X. (January 2020). "International Chronostratigraphic Chart" (PDF). International Commission on Stratigraphy. Retrieved 8 January 2021.
- ↑ 46.0 46.1 D. Dahl-Jensen & others (2013). "Eemian interglacial reconstructed from a Greenland folded ice core". Nature 493 (7433): 489–494. doi:10.1038/nature11789. PMID 23344358. http://nora.nerc.ac.uk/id/eprint/500331/1/2012-07-09846-NEEM_revised.pdf. Cite error: Invalid
<ref>
tag; name "Eemian" defined multiple times with different content - ↑ 47.0 47.1 Mike Walker; et al. (December 2018). "Formal ratification of the subdivision of the Holocene Series/Epoch (Quaternary System/Period)" (PDF). Episodes. Subcommission on Quaternary Stratigraphy (SQS). 41 (4): 213–223. doi:10.18814/epiiugs/2018/018016. Retrieved 11 November 2019.
- ↑ 48.0 48.1 P. L. Gibbard (2015). "The Quaternary System/Period and its major subdivisions". Russian Geology and Geophysics 56 (4): 686–688. doi:10.1016/j.rgg.2015.03.015.
- ↑ 49.0 49.1 D. Dahl-Jensen & others (2013). "Eemian interglacial reconstructed from a Greenland folded ice core". Nature 493 (7433): 489–94. doi:10.1038/nature11789. PMID 23344358. http://nora.nerc.ac.uk/id/eprint/500331/1/2012-07-09846-NEEM_revised.pdf.
- ↑ Lane, Megan (15 February 2011). "The moment Great Britain became an island". BBC News. BBC. Retrieved 5 November 2019.
- ↑ Winter, Barbara. "Bering Land Bridge". SFU Museum of Archaeology and Ethnology. Retrieved 2 March 2019.
{{cite web}}
:|archive-date=
requires|archive-url=
(help) - ↑ Carlson, A. E. (2013). The Younger Dryas Climate Event. 3. Elsevier. pp. 126–134. http://people.oregonstate.edu/~carlsand/carlson_encyclopedia_Quat_2013_YD.pdf.
- ↑ Bronowski, Jacob (1973). The Ascent of Man. London: BBC. ISBN 978-1-849-90115-4.
- ↑ "Ancient Egyptian Culture: Palaeolithic Egypt". Emuseum. Minnesota State University. 2002. Retrieved 18 November 2019.
{{cite web}}
:|archive-date=
requires|archive-url=
(help) - ↑ Willoughby, Pamela R. (2007). The Evolution of Modern Humans in Africa: A Comprehensive Guide. Rowman Altamira. pp. 181–182. ISBN 978-0759101197.
- ↑ Bouchneba, L.; Crevecoeur, I. (2009). "The inner ear of Nazlet Khater 2 (Upper Palaeolithic, Egypt)". Journal of Human Evolution 56 (3): 257–262. doi:10.1016/j.jhevol.2008.12.003. PMID 19144388.
- ↑ Tooley, M. J. (1979) Sea-level Changes: North-West England During the Flandrian Stage Clarendon Press, Oxford, England, ISBN 978-0-19-823228-5
- ↑ Stoker, Martyn S. (2010) "Late glacial ice-cap dynamics in NW Scotland: evidence from the fjords of the Summer Isles region" Quaternary Science Reviews 28(27/28): pp. 3161–3184, doi: 10.1016/j.quascirev.2009.09.012
- ↑ 59.0 59.1 59.2 59.3 Jan Mangerud (1987). W. H. Berger and L. D. Labeyrie. ed. The Alleröd/Younger Dryas Boundary, In: Abrupt Climatic Change. D. Reidel Publishing Company. pp. 163-71. http://folk.uib.no/ngljm/PDF_files/Mangerud%201987,YD%20boundary.PDF. Retrieved 2014-11-03.
- ↑ R. Muscheler; B. Kromer; S. Björck; A. Svensson; M. Friedrich; K. F. Kaiser; J. Southon (2008). "Tree rings and ice cores reveal 14C calibration uncertainties during the Younger Dryas". Nature Geoscience 1 (4): 263-7. doi:10.1038/ngeo128. http://www.nature.com/ngeo/journal/v1/n4/full/ngeo128.html. Retrieved 2014-10-09.
- ↑ 61.0 61.1 International Commission on Stratigraphy. "ICS chart containing the Quaternary and Cambrian GSSPs and new stages (v 2018/07) is now released!". Retrieved 15 July 2018.
- ↑ Amos, Jonathan (18 July 2018). "Welcome to the Meghalayan Age – a new phase in history". BBC News. Retrieved 19 July 2018.
- ↑ 63.0 63.1 International Commission on Stratigraphy. "GSSP Table – All Periods". GSSPs. Retrieved 15 July 2018.
- ↑ Jeffrey P. Donnelly; Neal W. Driscoll; Elazar Uchupi; Lloyd D. Keigwin; William C. Schwab; E. Robert Thieler; Stephen A. Swift (February 2005). "Catastrophic meltwater discharge down the Hudson Valley: A potential trigger for the Intra-Allerød cold period". Geology 33 (2): 89-92. doi:10.1130/G21043.1. http://geology.geoscienceworld.org/content/33/2/89.abstract. Retrieved 2014-11-04.
- ↑ 65.0 65.1 65.2 Konrad A. Hughes; Jonathan T. Overpeck; Larry C. Peterson; Susan Trumbore (7 March 1996). Rapid climate changes in the tropical Atlantic region during the last deglaciation. 380. pp. 51-4. http://www.diagonalarida.cl/SemV/Hughen_etal_1996_tropicalAtlantic.pdf. Retrieved 2014-11-05.
- ↑ V. N. Livina; F. Kwasniok; T. M. Lenton (2009). "Potential analysis reveals changing number of climate states during the last 60 kyr". Climate of the Past (European Geosciences Union) 5: 2223–2237. doi:10.5194/cpd-5-2223-2009. http://www.clim-past.net/6/77/2010/cp-6-77-2010.pdf.
- ↑ "International Stratigraphic Chart". International Commission on Stratigraphy. 2010. Retrieved 24 February 2012.
- ↑ 68.0 68.1 68.2 Zicheng Yu; Ulrich Eicher (2001). "Three Amphi-Atlantic Century-Scale Cold Events during the Bølling-Allerød Warm Period". Géographie physique et Quaternaire 55 (2): 171-9. doi:10.7202/008301ar. http://www.lehigh.edu/~ziy2/pubs/YuGpQPreprint.pdf. Retrieved 2014-11-04.
- ↑ 69.00 69.01 69.02 69.03 69.04 69.05 69.06 69.07 69.08 69.09 69.10 69.11 69.12 69.13 69.14 Barbara Wohlfarth (April 2010). "Ice-free conditions in Sweden during Marine Oxygen Isotope Stage 3?". Boreas 39: 377-98. doi:10.1111/j.1502-3885.2009.00137.x. http://people.su.se/~wohlf/pdf/Wohlfarth%20Boreas%202010.pdf. Retrieved 2014-11-06.
- ↑ 70.000 70.001 70.002 70.003 70.004 70.005 70.006 70.007 70.008 70.009 70.010 70.011 70.012 70.013 70.014 70.015 70.016 70.017 70.018 70.019 70.020 70.021 70.022 70.023 70.024 70.025 70.026 70.027 70.028 70.029 70.030 70.031 70.032 70.033 70.034 70.035 70.036 70.037 70.038 70.039 70.040 70.041 70.042 70.043 70.044 70.045 70.046 70.047 70.048 70.049 70.050 70.051 70.052 70.053 70.054 70.055 70.056 70.057 70.058 70.059 70.060 70.061 70.062 70.063 70.064 70.065 70.066 70.067 70.068 70.069 70.070 70.071 70.072 70.073 70.074 70.075 70.076 70.077 70.078 70.079 70.080 70.081 70.082 70.083 70.084 70.085 70.086 70.087 70.088 70.089 70.090 70.091 70.092 70.093 70.094 70.095 70.096 70.097 70.098 70.099 70.100 70.101 70.102 70.103 70.104 70.105 70.106 70.107 70.108 70.109 70.110 70.111 70.112 70.113 70.114 70.115 70.116 70.117 70.118 70.119 70.120 70.121 70.122 70.123 70.124 70.125 70.126 70.127 70.128 70.129 70.130 70.131 70.132 70.133 70.134 70.135 70.136 70.137 70.138 70.139 70.140 70.141 70.142 70.143 70.144 70.145 70.146 70.147 70.148 70.149 70.150 70.151 70.152 70.153 70.154 70.155 70.156 70.157 70.158 70.159 70.160 70.161 70.162 70.163 70.164 70.165 70.166 70.167 70.168 70.169 70.170 70.171 70.172 70.173 70.174 70.175 70.176 70.177 70.178 70.179 70.180 70.181 70.182 70.183 70.184 70.185 70.186 70.187 70.188 70.189 70.190 70.191 70.192 70.193 70.194 70.195 70.196 70.197 70.198 70.199 70.200 70.201 70.202 70.203 70.204 70.205 Lisiecki, L.E., 2005, Ages of MIS boundaries. LR04 Benthic Stack Boston University, Boston, MA
- ↑ 71.0 71.1 71.2 71.3 Michael Houmark-Nielsen, (30 November 1994). "Late Pleistocene stratigraphy, glaciation chronology and Middle Weichselian environmental history from Klintholm, Møn, Denmark". Bulletin of the Geological Society of Denmark 41 (2): 181-202. http://2dgf.dk/xpdf/bull41-02-181-202.pdf. Retrieved 2014-11-03.
- ↑ 72.00 72.01 72.02 72.03 72.04 72.05 72.06 72.07 72.08 72.09 72.10 72.11 72.12 Sasha Naomi Bharier Leigh (2007). A STUDY OF THE DYNAMICS OF THE BRITISH ICE SHEET DURING MARINE ISOTOPE STAGES 2 AND 3, FOCUSING ON HEINRICH EVENTS 2 AND 4 AND THEIR RELATIONSHIP TO THE NORTH ATLANTIC GLACIOLOGICAL AND CLIMATOLOGICAL CONDITIONS. St Andrews, Scotland: University of St Andrews. pp. 219. https://research-repository.st-andrews.ac.uk/bitstream/handle/10023/525/Sasha%20Leigh%20MPhil%20thesis.pdf?sequence=1. Retrieved 2017-02-16.
- ↑ George H. Denton; Thomas V. Lowell; Calvin J. Heusser; Patricio I. Moreno; Bjørn G. Andersen; Linda E. Heusser; Christian Schlüchter; David R. Marchant (1999). "Interhemispheric Linkage of Paleoclimate during the Last Glaciation". Geografiska Annaler. Series A, Physical Geography 81A (2): 107-53. http://people.bu.edu/marchant/Dave_FullText_Papers/Denton_GA_1999.pdf. Retrieved 2014-11-05.
- ↑ "These Vintage Threads Are 30,000 Years Old". NPR. Retrieved 2010-11-13.
{{cite web}}
:|archive-date=
requires|archive-url=
(help) - ↑ Balter, M (2009). "Clothes make the (Hu) Man". Science 325 (5946): 1329. doi:10.1126/science.325_1329a. PMID 19745126.
- ↑ Kvavadze, E; Bar-Yosef, O; Belfer-Cohen, A; Boaretto, E; Jakeli, N; Matskevich, Z; Meshveliani, T (2009). "30,000-Year-Old Wild Flax Fibers". Science 325 (5946): 1359. doi:10.1126/science.1175404. PMID 19745144. http://nrs.harvard.edu/urn-3:HUL.InstRepos:4270521.
- ↑ 77.0 77.1 A.A. Nikonov; M.M. Shakhnovich; J. van der Plicht (2011). "Age of Mammoth Remains from the Submoraine Sediments of the Kola Peninsula and Karelia". Doklady Earth Sciences 436 (2): 308-10. http://cio.eldoc.ub.rug.nl/FILES/root/2011/DoklEarthSciNikonov/2011DoklEarthSciNikonov.pdf?origin=publication_detail. Retrieved 2014-11-06.
- ↑ 78.0 78.1 J. Vandenberghe; G. Nugteren (2001). "Rapid climatic changes recorded in loess successions". Global and Planetary Change 28 (1-9): 222-30. http://shixi.bnu.edu.cn/field-trips/cooperation/ChinaSweden/the%20link/1.1.4.pdf. Retrieved 2014-11-06.
- ↑ 79.0 79.1 Edward A. Mankinen; Carl M. Wentworth (10 June 2003). Preliminary Paleomagnetic Results from the Coyote Creek Outdoor Classroom Drill Hole, Santa Clara Valley, California. U.S. Geological Survey. http://geopubs.wr.usgs.gov/open-file/of03-187/. Retrieved 2016-11-04.
- ↑ 80.0 80.1 80.2 80.3 80.4 80.5 80.6 80.7 Norbert R. Nowaczyk; Helge Arz (16 October 2012). Ice age polarity reversal was global event: Extremely brief reversal of geomagnetic field, climate variability, and super volcano. ScienceDaily: Helmholtz Centre Potsdam - GFZ German Research Centre for Geosciences. https://www.sciencedaily.com/releases/2012/10/121016084936.htm. Retrieved 2016-11-04.
- ↑ 81.0 81.1 81.2 81.3 81.4 81.5 81.6 Placzek, C.; Quade, J.; Patchett, P. J. (8 May 2006). "Geochronology and stratigraphy of late Pleistocene lake cycles on the southern Bolivian Altiplano: Implications for causes of tropical climate change". Geological Society of America Bulletin 118 (5-6): 515–532. doi:10.1130/B25770.1.
- ↑ 82.0 82.1 82.2 Placzek, C.J.; Quade, J.; Patchett, P.J. (February 2013). "A 130ka reconstruction of rainfall on the Bolivian Altiplano". Earth and Planetary Science Letters 363: 97–108. doi:10.1016/j.epsl.2012.12.017.
- ↑ Placzek, Christa J.; Quade, Jay; Patchett, P. Jonathan (January 2011). "Isotopic tracers of paleohydrologic change in large lakes of the Bolivian Altiplano". Quaternary Research 75 (1): 239. doi:10.1016/j.yqres.2010.08.004.
- ↑ 84.0 84.1 84.2 Zech, Michael; Zech, Roland; Morrás, Héctor; Moretti, Lucas; Glaser, Bruno; Zech, Wolfgang (March 2009). "Late Quaternary environmental changes in Misiones, subtropical NE Argentina, deduced from multi-proxy geochemical analyses in a palaeosol-sediment sequence". Quaternary International 196 (1-2). doi:10.1016/j.quaint.2008.06.006.
- ↑ Zech, Michael; Glaser, Bruno (30 January 2008). "Improved compound-specificδ13C analysis of n-alkanes for application in palaeoenvironmental studies". Rapid Communications in Mass Spectrometry 22 (2): 136. doi:10.1002/rcm.3342.
- ↑ Ward, D.; Thornton, R.; Cesta, J. (15 September 2017). "Across the Arid Diagonal: deglaciation of the western Andean Cordillera in southwest Bolivia and northern Chile". Cuadernos de Investigación Geográfica 43 (2): 689. doi:10.18172/cig.3209. ISSN 1697-9540. https://publicaciones.unirioja.es/ojs/index.php/cig/article/view/3209.
- ↑ Boismier, B. (2002). "Lynford Quarry, A Neanderthal butchery site". Current Archaeology 16 (182): 53-58.
- ↑ 88.0 88.1 Kliem, P.; Buylaert, J. P.; Hahn, A.; Mayr, C.; Murray, A. S.; Ohlendorf, C.; Veres, D.; Wastegård, S. et al. (2013-07-01). "Magnitude, geomorphologic response and climate links of lake level oscillations at Laguna Potrok Aike, Patagonian steppe (Argentina)". Quaternary Science Reviews. Potrok Aike Maar Lake Sediment Archive Drilling Project (PASADO) 71: 131–146. doi:10.1016/j.quascirev.2012.08.023. http://www.sciencedirect.com/science/article/pii/S0277379112003411.
- ↑ Anselmetti, Flavio S.; Ariztegui, Daniel; De Batist, Marc; Gebhardt, Catalina A.; Haberzettl, Torsten; Niessen, Frank; Ohlendorf, Christian; Zolitschka, Bernd (2009-06-01). "Environmental history of southern Patagonia unravelled by the seismic stratigraphy of Laguna Potrok Aike". Sedimentology 56 (4). doi:10.1111/j.1365-3091.2008.01002.x/abstract. ISSN 1365-3091. http://onlinelibrary.wiley.com/wol1/doi/10.1111/j.1365-3091.2008.01002.x/abstract.
- ↑ 90.0 90.1 Wastegård, S.; Veres, D.; Kliem, P.; Hahn, A.; Ohlendorf, C.; Zolitschka, B. (2013-07-01). "Towards a late Quaternary tephrochronological framework for the southernmost part of South America – the Laguna Potrok Aike tephra record". Quaternary Science Reviews. Potrok Aike Maar Lake Sediment Archive Drilling Project (PASADO) 71: 81–90. doi:10.1016/j.quascirev.2012.10.019. http://www.sciencedirect.com/science/article/pii/S0277379112004155.
- ↑ ROCEEH (1 July 2010). File:Motm 2010 07 Howiesons Poort.pdf. Wikimedia. https://commons.wikimedia.org/wiki/File:Motm_2010_07_Howiesons_Poort.pdf. Retrieved 11 July 2018.
- ↑ Paul Haesaerts & Hans Mestdagh (30 March 2000). "Pedosedimentary evolution of the last interglacial and early glacial sequence in the European loess belt from Belgium to central Russia". Netherlands Journal of Geosciences 79 (2/3): 313-324. https://www.cambridge.org/core/services/aop-cambridge-core/content/view/D5638FD7EEC7F7425B6FD6F2688A5A8D/S001677460002179Xa.pdf/pedosedimentary_evolution_of_the_last_interglacial_and_early_glacial_sequence_in_the_european_loess_belt_from_belgium_to_central_russia.pdf. Retrieved 16 October 2021.
- ↑ 93.0 93.1 93.2 E. Donald McKay III (24-25 April 2008). "Optical Ages Spanning Two Glacial-Interglacial Cycles from Deposits of the Ancient Mississippi River, North-Central Illinois". Geological Society of America Abstracts with Programs 40 (5): 78. https://gsa.confex.com/gsa/2008NC/finalprogram/abstract_137641.htm. Retrieved 2017-06-11.
- ↑ Janaina C. Santos; Alcina Magnólia Franca BarretoII; Kenitiro Suguio (16 August 2012). "Quaternary deposits in the Serra da Capivara National Park and surrounding area, Southeastern Piauí state, Brazil". Geologia USP. Série Científica 12 (3). doi:10.5327/Z1519-874X2012000300008. http://ppegeo.igc.usp.br/scielo.php?pid=S1519-874X2012000300009&script=sci_arttext. Retrieved 2015-01-20.
- ↑ 95.0 95.1 McMillan, A.A. (2005). "A provisional Quaternary and Neogene lithostratigraphic framework Great Britain". Netherland Journal of Geosciences 84 (2): 87–107. doi:10.1017/S0016774600022988.
- ↑ Walker, M. (2005). Quaternary Dating Methods. Chichester UK: Wiley. ISBN 0-470-86927-5.
- ↑ 97.0 97.1 97.2 Gibbard, P.L.; Boreham, S.; Cohen, K.M.; Moscariello, A. (2007). "Global chronostratigraphical correlation table for the last 2.7 million years". Cambridge UK: Subcommission on Quaternary Stratigraphy, Department of Geography, University of Cambridge.
{{cite web}}
:|archive-date=
requires|archive-url=
(help) - ↑ 98.0 98.1 Lisiecki, L.E.; Raymo, M.E. (2005). "A Pliocene-Pleistocene stack of 57 globally distributed benthic d18O records". Paleoceanography 20: PA1003. doi:10.1029/2004PA001071. http://www.lorraine-lisiecki.com/LisieckiRaymo2005.pdf.
- ↑ Böse et al. (2012), Quaternary Glaciations of Northern Europe, Quaternary Science Reviews 44, page 17-22.
- ↑ Scourse, JD (ed) (2006) The Isles of Scilly: Field Guide. Quaternary Research Association, London, 2006.
- ↑ "Greater London". Natural England. Retrieved 28 March 2013.
{{cite web}}
:|archive-date=
requires|archive-url=
(help) - ↑ Hallberg, G.R. (1986). "Pre-Wisconsin glacial stratigraphy of the Central Plains region in Iowa, Nebraska, Kansas, and Missouri". Quaternary Science Reviews 5: 11–15. doi:10.1016/0277-3791(86)90169-1.
- ↑ 103.0 103.1 Richmond, G.M.; Fullerton, D.S. (1986). "Summation of Quaternary glaciations in the United States of America". Quaternary Science Reviews 5: 183–196. doi:10.1016/0277-3791(86)90184-8.
- ↑ 104.0 104.1 104.2 104.3 104.4 104.5 104.6 104.7 M. Roy; P.U. Clark; R.W. Barendregt; J.R. Glasmann; R.J. Enkin (January/February 2004). "Glacial stratigraphy and paleomagnetism of late Cenozoic deposits of the north-central United States". Geological Society of America Bulletin 116 (1/2): 30-41. doi:10.1130/B25325.1. http://geo.oregonstate.edu/files/geo/Royetal-GSAB-2004.pdf. Retrieved 2017-06-11.
- ↑ 105.0 105.1 105.2 105.3 Andrew L. Darling; Karl E. Karlstrom; Andres Aslan; Rex Cole; Charles Betton; Elmira Wan (May 2009). "Quaternary incision rates and drainage evolution of the Uncompahgre and Gunnison Rivers, western Colorado, as calibrated by the Lava Creek B ash". Rocky Mountain Geology 44 (1): 71–83. doi:10.1130/B25325.1. https://www.researchgate.net/profile/Andrew_Darling/publication/250085188_Quaternary_incision_rates_and_drainage_evolution_of_the_Uncompahgre_and_Gunnison_Rivers_western_Colorado_as_calibrated_by_the_Lava_Creek_B_ash/links/5580978b08ae607ddc3226ca.pdf. Retrieved 2017-06-11.
- ↑ Gradstein, Felix M.; Ogg, James G.; Smith, Alan G., eds (2004). A Geological Time Scale 2004 (3rd ed.). Cambridge: Cambridge University Press. p. 28. ISBN 9780521786737. https://archive.org/details/geologictimescal2004grad.
- ↑ Maria Bianca Cita; Philip L. Gibbard; Martin J. Head; the ICS Subcommission on Quaternary Stratigraphy (September 2012). "Formal ratification of the GSSP for the base of the Calabrian Stage (second stage of the Pleistocene Series, Quaternary System)". Episodes 35 (3): 388-97. http://www.stratigraphy.org/GSSP/Calabrian2.pdf. Retrieved 2015-01-18.
- ↑ West, RG (1962). Vegetational history of the Early Pleistocene of the Royal Society Borehole at Ludham, Norfolk. Proceedings of the Royal Society of London, B155, pp.437-453
- ↑ Gibbard, PL, Zalasiewicz, JA & Mathers, SJ (1998). Stratigraphy of the marine Plio-Pleistocene crag deposits of East Anglia. In: van Kolfschoten, T & Gibbard, PL (eds). The Dawn of the Quaternary - proceedings of the SEQS-EuroMam Symposium : Kerkrade, 16-21 June 1996. Netherlands Institute of Applied Geoscience, 1998. isbn:9072869613 }}
- ↑ Philip L. Gibbard; Martin J. Head (September 2010). "The newly-ratified definition of the Quaternary System/Period and redefinition of the Pleistocene Series/Epoch, and comparison of proposals advanced prior to formal ratification". Episodes 33 (3): 152-8. http://www.stratigraphy.org/GSSP/Quaternary&Pleistocene.pdf. Retrieved 2015-01-20.
- ↑ D. Rio; R. Sprovieri; D. Castradori; E. Di Stefano (June 1998). "The Gelasian Stage (Upper Pliocene): A new unit of the global standard chronostratigraphic scale". Episodes 21 (2): 82-7. http://www.stratigraphy.org/GSSP/Gelasian.pdf. Retrieved 2015-01-20.
- ↑ D. Castradori; D. Rio; F. J. Hilgen; L. J. Lourens (June 1998). "The Global Standard Stratotype-section and Point (GSSP) of the Piacenzian Stage (Middle Pliocene)". Episodes 21 (2): 88-93. http://www.stratigraphy.org/GSSP/Piacenzian.pdf. Retrieved 2015-01-23.
- ↑ John A. Van Couvering; Davide Castradori; Maria Bianca Cita; Frederik J. Hilgen; Domenico Rio (September 2000). [http://www.stratigraphy.org/GSSP/Zanclean.pdf "The base of the Zanclean Stage and of the Pliocene Series"]. Episodes 23 (3): 179-87. http://www.stratigraphy.org/GSSP/Zanclean.pdf. Retrieved 2015-01-23.
- ↑ 114.0 114.1 F.J. Hilgen; S. Iaccarino; W. Krijgsman; G. Villa; C.G. Langereis (2000). "The Global Boundary Stratotype Section and Point (GSSP) of the Messinian Stage (uppermost Miocene)". Episodes 23 (3): 172-178. http://www.stratigraphy.org/GSSP/Messinian.pdf. Retrieved 2017-08-20.
- ↑ Felix M. Gradstein; Frits P. Agterberg; James G. Ogg; Jan Hardenbol; Paul Van Veen; Jacques Thierry; Zehui Huang (1995). A Triassic, Jurassic and Cretaceous Time Scale, In: Geochronology Time Scales and Global Stratigraphic Correlation. SEPM Special Publication No. 54. Society for Sedimentary Geology. doi:1-56576-024-7. http://archives.datapages.com/data/sepm_sp/SP54/A_Triassic_Jurassic_and_Cretaceous_Time_Scale.htm. Retrieved 2016-10-24.
- ↑ GeoWhen (2007)
- ↑ "Archived copy". Retrieved 2010-05-01.
{{cite web}}
:|archive-date=
requires|archive-url=
(help), Edward Petuch, Cenozoic Seas: The View From Eastern North America. isbn 0-8493-1632-4}} - ↑ Paleo Database: Astaracian
- ↑ Hohenegger, Johann; Ćorić, Stjepan; Wagreich, Michael (2014). "Timing of the Middle Miocene Badenian Stage of the Central Paratethys". Geologica Carpathica 65 (1). doi:10.2478/geoca-2014-0004. http://geologie.univie.ac.at/uploads/media/Hohenegger.etal.Badenian.age.2014.GC_1_.pdf.
- ↑ 120.0 120.1 Pieter Laga & Stephen Louwye (2006). "Disused Neogene and Quaternary regional stages from Belgium: Bolderian, Houthalenian, Antwerpian, Diestian, Deurnian, Kasterlian, Kattendijkian, Scaldisian, Poederlian, Merksemian and Flandrian". Geologica Belgica 9 (1-2): 215-224. https://popups.uliege.be/1374-8505/index.php?id=1226&file=1&pid=1220. Retrieved 19 October 2021.
- ↑ 121.0 121.1 Stephen Louwye, Jef Deckers, Jasper Verhaegen, Rieko Adriaens & Noël Vandenberghe (2020). "A review of the lower and middle Miocene of northern Belgium". Geologica Belgica 23 (3-4): 137-156. https://biblio.ugent.be/publication/8694505/file/8694508. Retrieved 19 October 2021.
- ↑ Paleo Database: Orleanian
- ↑ 123.0 123.1 Edward Petuch. "Edward Petuch". Retrieved 2010-05-01.
{{cite web}}
:|archive-date=
requires|archive-url=
(help) - ↑ Robert A. Rohde (18 January 2005). Paleogene Period. GeoWhen Database. http://www.stratigraphy.org/bak/geowhen/stages/Paleogene.html. Retrieved 2015-09-16.
- ↑ Paleo Database: Aegnian
- ↑ "ICS - Chart/Time Scale". www.stratigraphy.org.
- ↑ Mason, B.G.; Pyle, D.M. & Oppenheimer, C.; 2004: The size and frequency of the largest explosive eruptions on Earth, Bulletin of Volcanology 66(8), pp 735–748.
- ↑ Lanphere, M.A. & Baadsgaard, H.; 2001: Precise K–Ar, 40Ar/39Ar, Rb–Sr and U/Pb mineral ages from the 27.5 Ma Fish Canyon Tuff reference standard, Chemical Geology 175(3–4), pp 653–671.
- ↑ Paleobiology Database
- ↑ Paleobiology Database Late Arikareean
- ↑ "ICS - Chart/Time Scale". www.stratigraphy.org.
- ↑ Encyclopedia Britannica https://www.britannica.com/science/Eocene-Epoch
- ↑ "ICS - Chart/Time Scale". www.stratigraphy.org.
- ↑ "ICS - Chart/Time Scale". www.stratigraphy.org.
- ↑ "ICS - Chart/Time Scale". www.stratigraphy.org.
- ↑ Paleo Database: Arshantan
- ↑ "ICS - Chart/Time Scale". www.stratigraphy.org.
- ↑ "ICS - Chart/Time Scale". www.stratigraphy.org.
- ↑ "ICS - Chart/Time Scale". www.stratigraphy.org.
- ↑ International Commission on Stratigraphy 2017
- ↑ 141.0 141.1 141.2 141.3 141.4 Eustoquio Molina; Laia Alegret; Ignacio Arenillas; José A. Arz; Njoud Gallala; Jan Hardenbol; Katharina von Salis; Etienne Steurbaut et al. (December 2006). "The Global Boundary Stratotype Section and Point for the base of the Danian Stage (Paleocene, Paleogene, "Tertiary", Cenozoic) at El Kef, Tunisia - Original definition and revision". Episodes 29 (4): 263-73. http://www.stratigraphy.org/GSSP/Danian.pdf. Retrieved 2015-01-19.