Gases/Gaseous objects/Earth
"When Hurricane Ida slammed into Louisiana as huge Category 4 storm on Sunday (Aug. 29), the tempest's sheer size was evident from nearly a million miles away."[1]
This "new photo [on the right] from NASA's Epic camera on the NOAA Deep Space Climate Observatory (DSCOVR) shows Hurricane Ida as it appeared from Lagrange point 1, a a point between the sun and Earth that's about 1 million miles (1.5 million kilometers) from our planet, just as it hit the U.S. Gulf Coast."[1]
"From about 1 million miles away, NASA's EPIC camera on NOAA's Deep Space Climate Observatory saw Hurricane Ida as it was approaching landfall in Louisiana yesterday."[2]
"Hurricane Ida made landfall near Port Fourchon, Louisiana as a terrifying Category 4 hurricane, with wind speeds of up to 150 mph (240 kph) and torrential rain. It made landfall in the state 16 years to the day of the devastating Hurricane Katrina in 2005. The storm knocked out power an estimated 1 million customers and at least two deaths have been attributed to the storm, according to the New York Times. Ida was also expected to cause flooding from storm surge and wind damage."[1]
"By 4 p.m. EDT (20:00 GMT) Monday, Ida was downgraded to a tropical depression located about 20 miles (35 km) north-northwest Jackson, Mississippi and dropping heavy rainfall across parts of southeast Louisiana, Mississippi and western Alabama, according to the National Hurricane Center."[1]
"Initial assessments from the rideout crew at NASA's Michoud Assembly Facility report all personnel onsite are accounted for and there are no injuries. Michoud remains closed and is operating on generator power. There is no significant flooding at the facility. At this time, no damage to flight hardware has been observed and NASA personnel will be conducting detailed damage assessments today."[1]
Absorptions
[edit | edit source]
"[P]referential absorption of sunlight by ozone over long horizon paths gives the zenith sky its blueness when the sun is near the horizon".[3]
Clouds
[edit | edit source]

Def. a "large white puffy cloud"[4] is called a cumulus cloud.
Def. "[a] visible mass of
- water droplets suspended in the air ...
- dust,
- steam ...
- smoke ...
- a group or swarm"[5] is called a cloud.
Cumulus clouds look white because the water droplets reflect and scatter the sunlight without absorbing other colors.
"On any given day, about half of Earth is covered by clouds, which reflect more sunlight than land and water. Clouds keep Earth cool by reflecting sunlight, but they can also serve as blankets to trap warmth."[6]
Aerometeors
[edit | edit source]"Several times a year an atmospheric river [shown in the image on the right forming over Hawai'i]—a long, narrow conveyor belt of storms that stream in relentlessly from the Pacific Ocean—drops inches of rain or feet of snow on the U.S. west coast. Such a system triggered floods and mudslides in central and southern California this past weekend [2-3 February 2019]."[7]
"Atmospheric rivers flow through the sky about a mile above the ocean surface, and may extend across a thousand miles of ocean to the coast. Some bring routine rain but the more intense systems can carry as much water as 15 Mississippi Rivers. The series of storms striking land can arrive for days or, occasionally, weeks on end. They hit west-facing coastlines worldwide, although the U.S. experiences more than most other national coasts."[7]
The “atmospheric river scale” "ranks severity and impacts, from category 1 (weak) to category 5 (exceptional)."[7]
"Without a scale, we really had no way to objectively communicate what would be a strong storm or a weak one."[8]
"Scientists, the media and the public viewed atmospheric rivers as primarily a hazard, but the weaker ARs are quite beneficial. Water managers made it clear to us that a rating scale would be helpful."[8]
"The scale, published Tuesday in the Bulletin of the American Meteorological Society, ranks atmospheric rivers on five levels:"[7]
- Category 1: Weak—primarily beneficial
- Category 2: Moderate—mostly beneficial, but also somewhat hazardous
- Category 3: Strong—balance of beneficial and hazardous
- Category 4: Extreme—mostly hazardous, but also beneficial (if persistent drought)
- Category 5—Exceptional—primarily hazardous
Neutrals
[edit | edit source]"Energetic neutral atoms (ENA), emitted from the magnetosphere with energies of ∼50 keV, have been measured with solid-state detectors on the IMP 7/8 and ISEE 1 spacecraft. The ENA are produced when singly charged trapped ions collide with the exospheric neutral hydrogen geocorona and the energetic ions are neutralized by charge exchange."[9]
"The IMAGE mission ... High Energy Neutral Atom imager (HENA) ... images [ENAs] at energies between 10 and 60 keV/nucleon [to] reveal the distribution and the evolution of energetic [ions, including protons] as they are injected into the ring current during geomagnetic storms, drift about the Earth on both open and closed drift paths, and decay through charge exchange to pre‐storm levels."[10]
Cosmic rays
[edit | edit source]
When cosmic rays enter the Earth’s atmosphere they collide with molecules, mainly oxygen and nitrogen, to produce a cascade of billions of lighter particles, a so-called air shower.
An air shower is an extensive (many kilometres wide) cascade of ionized particles and electromagnetic radiation produced in the atmosphere when a primary cosmic ray (i.e. one of extraterrestrial origin) enters the atmosphere.
There is "a decrease in thunderstorms at the time of high cosmic rays and an increase in thunderstorms 2-4 days later."[11]
It is believed that proton energies exceeding 50 MeV in the lower belts at lower altitudes are the result of the beta decay of neutrons created by cosmic ray collisions with nuclei of the upper atmosphere. The source of lower energy protons is believed to be proton diffusion due to changes in the magnetic field during geomagnetic storms.[12]
The PAMELA experiment detected orders of magnitude higher levels of antiprotons than are expected from normal particle decays while passing through the SAA. This suggests the van Allen belts confine a significant flux of antiprotons produced by the interaction of the Earth's upper atmosphere with cosmic rays.[13] The energy of the antiprotons has been measured in the range from 60 - 750 MeV.
Atmospheric neutrinos result from the interaction of cosmic rays with atomic nuclei in the Earth's atmosphere, creating showers of particles, many of which are unstable and produce neutrinos when they decay. A collaboration of particle physicists from the Tata Institute of Fundamental Research (India), Osaka City University (Japan) and Durham University (UK) recorded the first cosmic ray neutrino interaction in an underground laboratory in Kolar Gold Fields in India in 1965.
"The major problems associated with the balloon borne positron measurements are (i) the unique identification against a vast background of protons, and (ii) corrections for the positrons produced in the residual atmosphere."[14]
"[T]o account for the atmospheric corrections ... first [use] the instrument to determine the negative muon spectrum at float altitude. ... [Use this] spectrum ... to normalize the analytically determined atmospheric electron-positron spectra. ... most of the atmospheric electrons and positrons at small atmospheric depths are produced from muon decay at [the energies from 0.85 to 14 GeV]."[14]
Protons
[edit | edit source]The Bastille Day Flare or Bastille Day Event was a powerful solar flare on July 14, 2000, occurring near the peak of the solar maximum in solar cycle 23.[15][16] [NOAA] Active region 9077 produced an X5.7-class flare, which caused an S3 radiation storm on Earth fifteen minutes later as energetic protons bombarded the ionosphere.[15][17] It was the biggest solar radiation event since 1989.[17] The proton event was four times more intense than any previously recorded since the launches of SOHO in 1995 and ACE in 1997.[15] The flare was followed by a full-halo coronal mass ejection[15] and a geomagnetic super storm on July 15-16. The extreme level, G5, was peaked in late hours of July 15.
Tauons
[edit | edit source]The "incoming neutrino flux [is disentangled] from the consequent τ air-shower physics. [To] establish the τ production rate we introduce an effective volume and mass for Earth-skimming τ’s, which is independent on any incoming neutrino flux [...]. This volume describes a strip within the Earth where neutrino/antineutrino-nucleon, ντ () − N, interactions may produce emerging τ−,τ+ leptons which then shower in air. [General] τ upward-going showers [can be compared] to detectors such as the ongoing photo-fluorescence ground-based observatory Auger".[18]
Muons
[edit | edit source]"Muons are produced, along with other particles, when cosmic rays (high-energy particles originating in outer space) interact with atomic nuclei in Earth’s atmosphere to produce ‘showers’ of secondary particles. The muons inherit the high energy of the parent cosmic rays, which enables them to penetrate and pass through the rock of the volcano and to be detected on the other side of the mountain. Because denser materials absorb more muons (just as dense materials such as bone absorb more X-rays), this provides a basis for producing shadow images of the volcano’s interior."[19]
"Muon radiography was first used in 1971 – not for volcanoes, but for investigating the interior of the pyramid of Chefren at Giza, Egypt. The Nobel-prize winning physicist Louis Alvarez placed a muon detector inside the pyramid to pick up changes in muon flux (rate of muon flow) that could indicate the presence of a hidden burial chamber. However, none was found."[19]
"In 2007, Hiroyuki Tanaka and collaborators from the University of Tokyo were the first to apply this technique to volcanoes. They carried out radiography of the top part of the Asama volcano in Honshu, Japan, which revealed a region with rock of low density under the bottom of the crater. The presence of low-density regions can be used in computer simulations that predict how possible eruptions could develop, indicating the most dangerous areas around the volcano. Their observations showed that muon radiography could indeed produce useful images of the internal structure of volcanoes."[19]
"The really important advantages of muon radiography of volcanoes are two-fold. First, whereas current indirect methods can provide information to a spatial resolution of some 100 m, muon radiography can be up to ten times more specific, mapping internal structures to a resolution of some 10 m. Second, muon radiography offers the possibility of continuous monitoring, thus potentially revealing the evolution of structures over time. The time resolution depends on the thickness of the rock traversed by the muons: the thicker it is, the fainter the muon flux and the longer it takes to accumulate enough muons for a picture. The time needed can thus be weeks, months or years."[19]
Vesuvius "is a special challenge, not only because it represents the highest volcanic risk in Europe, but also because of the mountain’s unusual structure. Vesuvius is in fact situated within the remnants of a much larger volcano, Mount Somma. Moreover, inside the summit of Vesuvius is a crater that is 500 m wide and 300 m deep: this means that, to look below the bottom of the crater, muons have to penetrate deep into the mountain, through almost two kilometres of rock, to reach the detector on the opposite side of the volcano. Only muons of very high energy travelling in a near-horizontal direction are able to pass through all this rock, so their flux at the detector is very low, making imaging extremely difficult."[19]
Beta particles
[edit | edit source]
"The Large Area Telescope (LAT) is a pair-conversion gamma-ray telescope onboard the Fermi Gamma-ray Space Telescope satellite. It has been used to measure the combined [cosmic-ray] CR electron and positron spectrum from 7 GeV to 1 TeV [20, 21]. The LAT does not have a magnet for charge separation. However, as pioneered by [22] and [23], the geomagnetic field can also be used to separate the two species without an onboard magnet. Müller and Tang [23] used the difference in geomagnetic cutoff for positrons and electrons from the east and west to determine the positron fraction between 10 GeV and 20 GeV. As reported below, we used the shadow imposed by the Earth and its offset direction for electrons and positrons due to the geomagnetic field, to separately measure the spectra of CR electrons and positrons from 20 GeV to 200 GeV. In this energy range, the 68% containment radius of the LAT point-spread function is 0.1° or better and the energy resolution is 8% or better."[20]
"The Large Area Telescope (LAT) detects individual gamma rays using technology similar to that used in terrestrial particle accelerators. Photons hit thin metal sheets, converting to electron-positron pairs, via a process known as pair production. These charged particles pass through interleaved layers of silicon microstrip detectors, causing ionization which produce detectable tiny pulses of electric charge. Researchers can combine information from several layers of this tracker to determine the path of the particles. After passing through the tracker, the particles enter the calorimeter, which consists of a stack of caesium iodide scintillator crystals to measure the total energy of the particles. The LAT's field of view is large, about 20% of the sky. The resolution of its images is modest by astronomical standards, a few arc minutes for the highest-energy photons and about 3 degrees at 100 MeV. The LAT is a bigger and better successor to the EGRET instrument on NASA's Compton Gamma Ray Observatory satellite in the 1990s. {{clear}]
Positrons
[edit | edit source]In 2009, the Fermi Gamma Ray Telescope in Earth orbit observed [an] intense burst of gamma rays corresponding to positron annihilations coming out of a storm formation. Scientists wouldn't have been surprised to see a few positrons accompanying any intense gamma ray burst, but the lightning flash detected by Fermi appeared to have produced about 100 trillion positrons. This has been reported by media in January 2011, it is an effect, never considered to happen before.[21]
"The Gamma-ray Burst Monitor (GBM) detects sudden flares of gamma-rays produced by gamma ray bursts and solar flares. Its scintillators are on the sides of the spacecraft to view all of the sky which is not blocked by the earth. The design is optimized for good resolution in time and photon energy. The Gamma-ray Burst Monitor has detected gamma rays from positrons generated in powerful thunderstorms.[22]
Electrons
[edit | edit source]


With respect to the rocky-object Earth, between the surface and various altitudes there is an electric field induced by the ionosphere. It changes with altitude from about 150 volts per meter at the suface to lower values at higher altitude. In fair weather, it is relatively constant, in turbulent weather it is accompanied by ions. At greater altitude these chemical species continue to increase in concentration. To dissipate the accumulation of greater charge differential between the surface and the ionosphere, the gases between suffer breakdown (ionization) that permits lightning to be either a draw of negative charge, usually electrons, upward from the surface or a transfer of positive charge to the ground.
"[L]ow-altitude regions of downward electric current on auroral magnetic field lines are sites of dramatic upward magnetic field-aligned electron acceleration that generates intense magnetic field-aligned electron beams within Earth’s equatorial middle magnetosphere."[24]
Gamma rays
[edit | edit source]The Earth's atmosphere is a relatively bright source of gamma rays produced in interactions of ordinary cosmic ray protons with air atoms. In gamma-ray and X-ray astronomy, Earth is a dwarf gaseous object.
A number of observations by space-based telescopes have revealed gamma ray emissions, specifically, terrestrial gamma-ray flashes (TGFs). These observations pose a challenge to current theories of lightning, especially with the discovery of the clear signatures of antimatter produced in lightning.[25]
A TGF has been linked to an individual lightning stroke occurring within 1.5 ms of the TGF event,[26] proving for the first time that the TGF was of atmospheric origin and associated with lightning strikes.
The Reuven Ramaty High Energy Solar Spectroscopic Imager (RHESSI) spacecraft "has been observing TGFs at a much higher rate, indicating that these occur about 50 times per day globally (still a very small fraction of the total lightning on the planet). The energy levels recorded exceed 20 MeV. [Apparently], the gamma radiation fountains upward from starting points at surprisingly low altitudes in thunderclouds."[27]
"These are higher energy gamma rays than come from the sun. And yet here they are coming from the kind of terrestrial thunderstorm that we see here all the time."[28]
In 2009, [the] Fermi Gamma Ray Telescope in Earth orbit observed [an] intense burst of gamma rays corresponding to positron annihilations coming out of a storm formation. Scientists wouldn't have been surprised to see a few positrons accompanying any intense gamma ray burst, but the lightning flash detected by Fermi appeared to have produced about 100 trillion positrons. This has been reported by media in January 2011, it is an effect, never considered to happen before.[29]
Airborne gamma-ray spectrometry is now the accepted leading technique for uranium prospecting with worldwide applications for geological mapping, mineral exploration & environmental monitoring.
X-rays
[edit | edit source]

The Earth is a known astronomical object. It is usually not thought of as an X-ray source.
At left is a composite image which contains the first picture of the Earth in X-rays, taken in March, 1996, with the orbiting Polar satellite. The area of brightest X-ray emission is red.
Energetic charged particles from the Sun energize electrons in the Earth's magnetosphere. These electrons move along the Earth's magnetic field and eventually strike the ionosphere, causing the X-ray emission.
X-ray observations offer the possibility to detect (X-ray dark) planets as they eclipse part of the corona of their parent star while in transit.
"Such methods are particularly promising for low-mass stars as a Jupiter-like planet could eclipse a rather significant coronal area."[30]
"Auroras are produced by solar storms that eject clouds of energetic charged particles. These particles are deflected when they encounter the Earth’s magnetic field, but in the process large electric voltages are created. Electrons trapped in the Earth’s magnetic field are accelerated by these voltages and spiral along the magnetic field into the polar regions. There they collide with atoms high in the atmosphere and emit X-rays".[31]
Ultraviolets
[edit | edit source]
"This unusual false-color image [at right] shows how the Earth glows in ultraviolet (UV) light. The Far UV Camera/Spectrograph deployed and left on the Moon by the crew of Apollo 16 captured this image. The part of the Earth facing the Sun reflects much UV light and bands of UV emission are also apparent on the side facing away from the Sun. These bands are the result of aurora caused by charged particles given off by the Sun. They spiral towards the Earth along Earth's magnetic field lines."[32]
In April 1972, the Apollo 16 mission recorded various astronomical photos and spectra in ultraviolet with the Far Ultraviolet Camera/Spectrograph.[33]
Archaeological "[a]erial survey ... employs ultraviolet, infrared, ground-penetrating radar wavelengths, LiDAR and thermography.[34]
Opticals
[edit | edit source]
"A NASA camera on the Deep Space Climate Observatory (DSCOVR) satellite has returned its first view of the entire sunlit side of Earth [on the right] from one million miles away."[35]
"The color images of Earth from NASA’s Earth Polychromatic Imaging Camera (EPIC) are generated by combining three separate images to create a photographic-quality image. The camera takes a series of 10 images using different narrowband filters -- from ultraviolet to near infrared -- to produce a variety of science products. The red, green and blue channel images are used in these Earth images."[35]
"This first DSCOVR image of our planet demonstrates the unique and important benefits of Earth observation from space. As a former astronaut who’s been privileged to view the Earth from orbit, I want everyone to be able to see and appreciate our planet as an integrated, interacting system. DSCOVR’s observations of Earth, as well as its measurements and early warnings of space weather events caused by the sun, will help every person to monitor the ever-changing Earth, and to understand how our planet fits into its neighborhood in the solar system.”[36]
"These initial Earth images show the effects of sunlight scattered by air molecules, giving the images a characteristic bluish tint."[35]
"The images clearly show [...] complex cloud patterns."[37]
"The primary objective of DSCOVR, a partnership between NASA, the National Oceanic and Atmospheric Administration (NOAA) and the U.S. Air Force, is to maintain the nation’s real-time solar wind monitoring capabilities, which are critical to the accuracy and lead time of space weather alerts and forecasts from NOAA."[35]
NASA's Earth Polychromatic Imaging Camera (EPIC) "is a four megapixel CCD camera and telescope. The color Earth images are created by combining three separate single-color images to create a photographic-quality imageequivalent to a 12-megapixel camera. The camera takes a series of 10 images using different narrowband filters -- from ultraviolet to near infrared -- to produce a variety of science products. The red, green and blue channel images are used to create the color images. Each image is about 3 megabytes in size."[35]
"The effective resolution of the DSCOVR EPIC camera is somewhere between 6.2 and 9.4 miles (10 and 15 kilometers)."[37]
Blues
[edit | edit source]
Def. "[t]he gases surrounding the Earth or any astronomical body"[38] is called an atmosphere.
Atmospheric gases scatter blue light more than other wavelengths, giving the Earth a blue halo when seen from space, as shown in the image at right.
Greens
[edit | edit source]
In the International Space Station image at right, you can "see green and yellow airglow paralleling the Earth’s horizon line (or limb) before it is overwhelmed by the light of the rising Sun. Airglow is the emission of light by atoms and molecules in the upper atmosphere after they are excited by ultraviolet radiation. ... Astronaut photograph ISS030-E-015491 was acquired on December 22, 2011, with a Nikon digital camera, and is provided by the ISS Crew Earth Observations experiment and Image Science & Analysis Laboratory, Johnson Space Center."[39]
Airglow (also called nightglow) is the very weak emission of light by a planetary atmosphere. In the case of Earth's atmosphere, this optical phenomenon causes the night sky to never be completely dark (even after the effects of starlight and diffused sunlight from the far side are removed).
Airglow is caused by various processes in the upper atmosphere, such as the recombination of ions which were photoionized by the sun during the day, luminescence caused by cosmic rays striking the upper atmosphere, and chemiluminescence caused mainly by oxygen and nitrogen reacting with hydroxyl ions at heights of a few hundred kilometers. It is not noticeable during the daytime because of the scattered light from the Sun.
Oranges
[edit | edit source]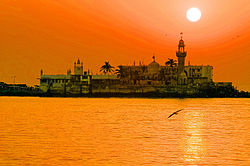

But truly, the now famous sun set at the peak where you will witness the panorama of rolling green hills and also a glimpse of Arabian sea and the bright orange sun going down the hills might force one to contemplate on the nature and its complex beauty.
Reds
[edit | edit source]



The Belt of Venus or Venus's Girdle is the Victorian-era name for an atmospheric phenomenon seen at sunrise and sunset. Shortly after sunset or shortly before sunrise, the observer is, or is very nearly, surrounded by a pinkish glow (or anti-twilight arch) that extends roughly 10°–20° above the horizon. Often, the glow is separated from the horizon by a dark layer, the Earth's shadow or "dark segment". The Arch's light rose (pink) color is due to backscattering of reddened light from the rising or setting Sun.
The first image at left shows the Belt of Venus from 42,000 feet altitude locally above the Earth's surface.
In the image at right is the Belt of Venus, a pink band that is visible above the dark blue of the Earth's shadow, in the same part of the sky. No defined line divides the Earth's shadow and the Belt of Venus; one colored band blends into the other in the sky.
In the image at second left, the Belt of Venus is shown at sunset, looking east from the Marin Headlands just north of San Francisco. There is a thin greyish cloud layer partially obscuring the horizon in this image.
The second image at right shows the full Moon rising as seen through the Belt of Venus.
In the right viewing conditions, a pink (or orange or purple) band is visible in the twilight sky just above the dark blue band of the Earth's shadow. This pink band is called the "anti-twilight arch" or "Belt of Venus". The name "Belt of Venus" is not connected with the planet Venus; the Belt of Venus is part of Earth's upper atmosphere which is illuminated by the setting or rising sun. It is visible either after the sun ceases to be visible (at sunset) or before the sun becomes visible (at sunrise).[40][41]
When the sun is near the horizon at sunset or sunrise, the light from the sun is red; this is because the light is reaching the observer through an especially thick layer of the atmosphere, which works as a filter, scattering all but the red light.
From the viewpoint of the observer, the red sunlight directly illuminates small particles in the lower atmosphere on the other side of the sky from the sun. The red light is backscattered to the observer, and that is why the Belt of Venus appears pink.
The lower the sunset sun descends, the less clearly distinguished the boundary between the Earth's shadow and Belt of Venus becomes. This is because now the setting sun illuminates a thinner part of the upper atmosphere. The red light is not scattered there because there are fewer particles, and the eye only sees the "normal" (usual) blue sky, which is due to Rayleigh scattering from air molecules. Eventually, both the Earth's shadow and the Belt of Venus dissolve into the darkness of the night sky.[41]
Infrareds
[edit | edit source]


The images at top right are from the optical astronomy and infrared telescopes aboard the MESSENGER spacecraft.
The optical astronomy image delineates gaseous, liquid, and rocky portions of the Earth's atmosphere, oceans, and land masses. The infrared astronomy image detects primarily the rocky objects or land masses of Earth.
The second image at right is the "first full-disk thermal infrared (IR) image, [taken by GOES 14,] showing radiation with a wavelength of 10.7 micrometers emanating from Earth."[42]
"Infrared images are useful because they provide information about temperatures. A wavelength of 10.7 micrometers is 15 times longer than the longest wavelength of light (red) that people can see, but scientists can turn the data into a picture by having a computer display cold temperatures as bright white and hot temperatures as black. The hottest (blackest) features in the scene are land surfaces; the coldest (whitest) features in the scene are clouds."[42]
"In the heat of the midday sun, the exposed rock in sparsely vegetated mountain ranges and high-altitude deserts in western North and South America are dark. In North America, the temperatures cool (fade to lighter grey) along a gradient from west to east, as the semi-deserts of the West and South-west transition to the grasslands and crop-lands of the Great Plains, which transition to forests in the East."[42]
"A band of scattered storms across the equatorial Pacific shows the location of the Inter-tropical Convergence Zone, which is a belt of showers and thunderstorms that persists near the equator year round. Need help precisely locating the equator? Look for the dark (hot) spots in the Pacific Ocean west of South America: those are the Galapagos Islands, and the equator passes through the northern tip of the largest island."[42]
"Perhaps the most significant features related to U.S. weather appear in the upper right quadrant of the disk: the remnants of Tropical Storm Claudette drenching the eastern Gulf Coast, Tropical Depression Ana unwinding over Puerto Rico and the Dominican Republic, and Hurricane Bill approaching from the central Atlantic."[42]
At left is an image from the Galileo spacecraft. The "Galileo raw image of Earth [is] taken from 2.7 million km 3 days after the first flyby. Africa and the Middle East can be seen at the top and Antarctica at the bottom. Weather systems are visible in the clouds over the Atlantic and Indian oceans. The image was taken through the infrared (9680 nm) filter, which enhances the visibility of the land masses."[43]
Radars
[edit | edit source]
On the right is a composite of hourly radar images. These wind gusts averaged ~75 mph over about 450 miles. This is referred to as the Derecho event.
Gases
[edit | edit source]Def. matter "that can be contained only if it is fully surrounded by a solid (or in a bubble of liquid) (or held together by gravitational pull); it can condense into a liquid, or can (rarely) become a solid directly"[44] is called a gas.
"Turbines have been around for a long time—windmills and water wheels are early examples. The name comes from the Latin turbo, meaning vortex, and thus the defining property of a turbine is that a fluid or gas turns the blades of a rotor, which is attached to a shaft that can perform useful work."[45]
Def. relating "to, or existing as, gas"[46] is called gaseous.
Gaseous objects
[edit | edit source]"[T]he evolution of star accretion onto a supermassive gaseous object in the central region of an active galactic nucleus [may be addressed using] a gaseous model of relaxing dense stellar systems".[47]
Gaseous objects have at least one chemical element or compound present in the gaseous state. These gaseous components make up at least 50 % of the detectable portion of the gaseous object such as the image on the right which was produced by detecting only water vapor in the infrared by the GOES-10 satellite.
Chemicals
[edit | edit source]The concentration of water vapor varies significantly from around 10 ppm by volume in the coldest portions of the atmosphere to as much as 5% by volume in hot, humid air masses, and concentrations of other atmospheric gases are typically quoted in terms of dry air (without water vapor).[48]
The remaining gases are often referred to as trace gases,[49] among which are principally carbon dioxide, methane, nitrous oxide, and ozone.
Gas | Volume(A) | ||
---|---|---|---|
Name | Formula | in ppmv(B) | in % |
Nitrogen | N2 | 780,840 | 78.084 |
Oxygen | O2 | 209,460 | 20.946 |
Argon | Ar | 9,340 | 0.9340 |
Carbon dioxide | CO 2 |
400 | 0.04[51] |
Neon | Ne | 18.18 | 0.001818 |
Helium | He | 5.24 | 0.000524 |
Methane | CH4 | 1.79 | 0.000179 |
Not included in above dry atmosphere: | |||
Water vapor(C) | H2O | 10–50,000(D) | 0.001%–5%(D) |
notes: (A) volume fraction is equal to mole fraction for ideal gas only, |
The relative concentration of gases remains constant until about 10,000 m (33,000 ft).[52]
Atmospheres
[edit | edit source]



These molecules in many instances are in turn made up of atoms of chemical elements. At your geographical location, specified in latitude and longitude, this gaseous envelope extends upward. The atmosphere of Earth changes with altitude. At high enough altitude the composition changes significantly, as does the temperature and pressure.
The atmospheric pressure at sea level averages 101.325 kPa (14.696 psi),[53] with a scale height of about 8.5 km (5.3 mi).[54]
A dry atmosphere is composed of 78.084% nitrogen, 20.946% oxygen, 0.934% argon, and trace amounts of carbon dioxide and other gaseous molecules.[53]
Water vapor content varies between 0.01% and 4%[53] but averages about 1%.[54]
The atmosphere transports water vapor.[55]
It causes small meteors to burn up before they strike the surface.[55]
Trace molecules within the atmosphere serve to capture thermal energy emitted from the ground, thereby raising the average temperature: water vapor, carbon dioxide, methane, nitrous oxide, and ozone, where the average surface temperature would be −18 °C (0 °F), in contrast to the current +15 °C (59 °F).[56]
It moderates temperature.[55]
In May 2017, glints of light, seen as twinkling from an orbiting satellite a million miles away, were found to be reflected light from ice crystals in the atmosphere.[57][58]
The ozone layer blocks ultraviolet solar radiation.[59]
The map at right shows the global long term monthly average surface air temperature for Earth.
Temperature trends in two thick layers of the atmosphere [image on the right] were measured between January 1979 and December 2005 by Microwave Sounding Units and Advanced Microwave Sounding Units on NOAA weather satellites. The instruments record microwaves emitted from oxygen molecules in the atmosphere.[60]
Total atmospheric mass is 5.1480×1018 kg (1.135×1019 lb).[61]
The zones of Earth's climates, going from the equator upward (and downward) are Tropical, Dry, Moderate, Continental and Polar. There are subzones within these zones.
Climates
[edit | edit source]
tropical rainforest climate (Af) tropical monsoon climate (Am) tropical savanna climate (Aw/As) | hot desert climate (BWh) cold desert climate (BWk) hot semi-arid climate (BSh) cold semi-arid climate (BSk) | hot-summer Mediterranean climate (Csa) warm-summer Mediterranean climate (Csb) cold-summer Mediterranean climate (Csc) | humid subtropical climate (Cwa) subtropical highland climate (Cwb) subtropical highland climate (Cwc) | humid subtropical climate (Cfa) oceanic climate (Cfb) subpolar oceanic climate (Cfc) | humid continental climate (Dsa) humid continental climate (Dsb) subarctic climate (Dsc) subarctic climate (Dsd) | humid continental climate (Dwa) humid continental climate (Dwb) subarctic climate (Dwc) subarctic climate (Dwd) | humid continental climate humid continental climate subarctic climate (Dfc) subarctic climate (Dfd) | tundra climate (ET) ice cap climate (EF) |
The image centered above contains the Köppen-Geiger climate classification maps at 1-km resolution.[62]
Tropics
[edit | edit source]






Def. from "or similar to a hot humid climate" occurring within or near to "the equatorial region between 23 degrees north and 23 degrees south"[63] as the "short term state of the atmosphere at a specific time and place, including the temperature, relative humidity, cloud cover, precipitation, wind, etc"[64] is called the tropics, or tropical weather.
The map on the right shows the approximate atmospheric locations per the geographic grid where the Köppen–Geiger climate classification map for Tropical occurs.
The map on the left indicates the geographic zone affected by the Sun being at the zenith for portions of an Earth year.
The graphs in the center image indicate water rainfall monthly averages per latitude. The tropics receive more precipitation than higher latitudes. The precipitation maximum, which follows the solar equator through the year, is under the rising branch of the Hadley circulation.
Under the Köppen climate classification, for tropical climates, a wet-season month is defined as a month where average precipitation is 60 millimetres (2.4 in) or more.[65]
In some tropical regions rainfall is equally distributed through the year.[66] Some areas with pronounced rainy seasons see a break in rainfall during mid-season when the intertropical convergence zone or monsoon trough moves poleward of their location during the middle of the warm season;[67]
In rainforest climates (Af) such as marked in blue in the second image down on the right has rainfall normally heavy throughout the year, where one day in an equatorial climate can be very similar to the next, while the change in temperature between day and night may be larger than the average change in temperature during the year.[68]
Tropical monsoon climate (Am) regions marked in the third image down on the right results when the monsoon winds change direction according to the seasons, where there is a short but distinct dry season which nearly always occurs at or soon after the "winter" solstice for that side of the equator with rainfall less than 60 mm, but more than 1/25 the total annual precipitation.[68]
Tropical savanna climate (As) or tropical wet and dry climate (Aw) shown in the fourth image down on the right is a type of climate that corresponds to the Köppen climate classification categories "Aw" and "As", with monthly mean temperatures above 18 °C (64 °F) in every month of the year and typically a pronounced dry season, with the driest month having less than 60 mm (2.36 inches) of precipitation and less than 100 – total annual precipitation mm/25 of precipitation.[68]
The map second down on the left is an excellent illustration of the Hadley cell where the vertical velocity is 500 hPa (Pa/sec) in July from ERA-40 reanalysis, 1979-2001 average; the negative (blue) values represent rising air; and positive (red) values indicate sinking air. It also illustrates that atmospheric regions extend across bodies of water between the land.
Deserts
[edit | edit source]
The desert climate (in the Köppen climate classification BWh and BWk) illustrated in the image on the right is a climate in which there is an excess of evaporation over precipitation, covering 14.2% of earth's land area, hot deserts may be the most common type of climate on earth.[69]
Most desert and arid climates receive between 25 and 200 mm (1 to 8 inches) of rainfall annually.[70] In the Köppen classification system, a climate is classed as arid if
- its mean annual precipitation in millimeters is less than ten times its defined precipitation threshhold,
- its mean annual precipitation is less than five times this threshold,
- the precipitation threshold is twice its mean annual temperature in degrees Celsius, plus a constant to represent the distribution of its rainfall throughout the year
- the constant is 28 for regions that receive 70% or more of their rainfall during the six winter months,
- the constant is 0 for regions that receive 70% or more of their rainfall during the six summer months
- it is 14 for any climates falling between these two extremes.[71]
There are deserts designated BWh (hot desert climates) and BWk (cold desert climates).
Altitude regions
[edit | edit source]



On the right, the 1962 US Standard Atmosphere graph of geometric altitude is plotted against air density, pressure, the speed of sound and temperature with approximate altitudes of various objects.[72]
In the center image the Space Shuttle Endeavour is orbiting in the thermosphere. Because of the angle of the photo, it appears to straddle the stratosphere and mesosphere that actually lie more than 250 km below. The orange layer is the troposphere, which gives way to the whitish stratosphere and then the blue mesosphere.[73]
In summary, the mass of Earth's atmosphere is distributed approximately as follows:[74]
- 50% is below 5.6 km (18,000 ft).
- 90% is below 16 km (52,000 ft).
- 99.99997% is below 100 km (62 mi; 330,000 ft), the Kármán line.
The image on the left shows the Moon at the centre, with the limb of Earth near the bottom transitioning into the orange-colored troposphere. The troposphere ends abruptly at the tropopause, which appears in the image as the sharp boundary between the orange- and blue-colored atmosphere. The silvery-blue noctilucent clouds extend far above Earth's troposphere.
Earth's atmosphere can be divided (called atmospheric stratification) into five main layers. Excluding the exosphere, the atmosphere has four primary layers, which are the troposphere, stratosphere, mesosphere, and thermosphere.[75] From highest to lowest, the five main layers are:
- Exosphere: 700 to 10,000 km (440 to 6,200 miles)
- Thermosphere: 80 to 700 km (50 to 440 miles)[76]
- Mesosphere: 50 to 80 km (31 to 50 miles)
- Stratosphere: 12 to 50 km (7 to 31 miles)
- Troposphere: 0 to 12 km (0 to 7 miles)[77]
The Earth's atmosphere is divided into altitude regions:[78]
- Troposphere — surface to 8,000 m at the poles – 18,000 m at the equator, ending at the Tropopause.
- Stratosphere — Troposphere to 50 km
- Mesosphere — Stratosphere to 85 km
- Thermosphere — Mesosphere to 675 km
- Exosphere — Thermosphere to 10,000 km.
Tropospheres
[edit | edit source]



Def. the "lower levels of the atmosphere extending from [a solid or liquid] surface up to the tropopause ... characterized by convective air movements and a large vertical temperature change"[79] is called the troposphere.
The troposphere, the lowest layer of Earth's atmosphere, extends from Earth's surface to an average height of about 12 km (7.5 mi; 39,000 ft), although this altitude varies from about 9 km (5.6 mi; 30,000 ft) at the geographic poles to 17 km (11 mi; 56,000 ft) at the Equator,[77] with some variation due to weather. The troposphere is bounded above by the tropopause, a boundary marked in most places by a temperature inversion (i.e. a layer of relatively warm air above a colder one), and in others by a zone which is isothermal with height.[80][81]
The troposphere "contains about four-fifths [80 %] of the mass of the whole atmosphere and 99% of the total mass of water vapor and aerosols."[82]
Rotational turbulent mixing plays an important role in the troposphere's structure and behaviour and most of the phenomena associated with day-to-day weather occur in the troposphere.[83]
The pressure of the atmosphere is maximum at sea level and decreases with altitude, because the atmosphere is very nearly in hydrostatic equilibrium so that the pressure is equal to the weight of air above a given point; thus, the change in pressure with altitude can be equated to the density with the hydrostatic equation[84]
where:
- gn is the standard gravity
- ρ is the density
- z is the altitude
- P is the pressure
- R is the gas constant
- T is the thermodynamic (absolute) temperature
- m is the molar mass
The second image down on the right shows the temperature trend in the Middle Troposphere as measured by a series of satellite-based instruments between January 1979 and December 2005. The middle troposphere is centered around 5 kilometers above the surface. Oranges and yellows dominate the troposphere image, indicating that the air nearest the Earth's surface warmed during the period.[85]
The temperature of the troposphere generally decreases as altitude increases. The rate at which the temperature decreases, , is called the environmental lapse rate (ELR).
Altitude Region | Lapse rate | Lapse Rate |
---|---|---|
(m) | (Kelvin/km) | (°F/1000 feet) |
0 – 11,000 | 6.5 | 3.57 |
11,000 – 20,000 | 0.0 | 0.0 |
20,000 – 32,000 | -1.0 | -0.55 |
32,000 – 47,000 | -2.8 | -1.54 |
47,000 – 51,000 | 0.0 | 0.0 |
51,000 – 71,000 | 2.8 | 1.54 |
71,000 – 85,000 | 2.0 | 1.09 |
For dry air, which is approximately an ideal gas, the adiabatic equation for an ideal gas is[86]
where is the heat capacity ratio (=7/5, for air).
Combining with the equation for the pressure, produces the dry adiabatic lapse rate.[87]
In the troposphere, the average environmental lapse rate is a drop of about 6.5 °C for every 1 km (1,000 meters) in increased height.[83]
The average thickness of the tropical troposphere is roughly 7 kilometers greater than the average tropospheric thickness at the poles.[88]
A zonal flow regime as illustrated in the third diagram down on the right is the meteorological term meaning that the general flow pattern is west to east along the Earth's latitude lines, with weak shortwaves embedded in the flow.[89]
When the zonal flow buckles, the atmosphere can flow in a more longitudinal (or meridional [in the fourth image down on the right]) direction, and thus the term "meridional flow" arises, which features strong, amplified troughs of low pressure and ridges of high pressure, with more north-south flow in the general pattern than west-to-east flow.[90]
The three cells model (fifth image down on the right) of the atmosphere attempts to describe the actual flow of the Earth's atmosphere as a whole using the:
- tropical (Hadley cell),
- mid latitude (Ferrel cell), and
- polar (polar cell) regions to describe energy flow and global atmospheric circulation (mass flow) – the energy that the Earth absorbs from the Sun each year is equal to that which it loses to space by radiation,where the result is a circulation of the atmosphere that transports warm air poleward from the tropics and cold air equatorward from the poles so the effect of the three cells is the tendency to even out the heat and moisture in the Earth's atmosphere around the planet.[91]
The troposphere contains the planetary boundary layer, and ranges in height from an average of 9 km (5.6 mi; 30,000 ft) at the poles, to 17 km (11 mi; 56,000 ft) at the Equator.[92][93]
Tropopauses
[edit | edit source]Def. the "zone of transition between the troposphere and the stratosphere"[94] is called the tropopause.
The tropopause is an inversion layer, where the air temperature ceases to decrease with height and remains constant through its thickness.[83]
"The boundary between the troposphere and the stratosphere, where an abrupt change in lapse rate usually occurs. It is defined as the lowest level at which the lapse rate decreases to 2 °C/km or less, provided that the average lapse rate between this level and all higher levels within 2 km does not exceed 2 °C/km."[95]
The tropopause as defined above renders as a first-order discontinuity surface, that is, temperature as a function of height varies continuously through the atmosphere but the temperature gradient does not.[96]
Stratospheres
[edit | edit source]Def. the "region ... where temperature increases along with the altitude ... [extending] from the tropopause ... to ... the mesosphere"[97] is called the stratosphere.
Mesospheres
[edit | edit source]Def. a "layer of the Earth's atmosphere that is directly above the stratosphere and directly below the thermosphere"[98] is called a mesosphere.
Temperatures drop with increasing altitude to the mesopause that marks the top of this middle layer of the atmosphere, the coldest place on Earth and has an average temperature around −85 °C (−120 °F; 190 K).[99][100]
Thermospheres
[edit | edit source]
Def. a "layer of the Earth's atmosphere directly above the mesosphere and directly below the exosphere"[101] is called a thermosphere.
Within the thermosphere, ultraviolet radiation causes photoionization/photodissociation of molecules, creating ions in the ionosphere beginning at about 80 km (50 mi) above sea level.[102]
The temperature of the thermosphere gradually increases with height, the inversion in the thermosphere occurs due to the extremely low density of its molecules, the temperature of this layer can rise as high as 1500 °C (2700 °F), though the gas molecules are so far apart, i.e., the air is so rarefied that an individual molecule (of oxygen, for example) travels an average of 1 kilometre (0.62 mi; 3300 ft) between collisions with other molecules.[103]
Exospheres
[edit | edit source]Def. "the uppermost layer of a planet's atmosphere"[104] or "an extremely thin atmosphere""[105] is called an exosphere.
The exosphere is a thin, atmosphere-like volume surrounding a planet or natural satellite where molecules are gravitationally bound to that body, but where the density is too low for them to behave as a gas by colliding with each other.[106]
Mercury and several large moons, such as the Moon and the Galilean satellites of Jupiter, have exospheres without a denser atmosphere underneath,[107] referred to as a surface boundary exosphere.[108]
The lower boundary of the exosphere is called the exobase, exopause or 'critical altitude' as this is the altitude where barometric conditions no longer apply and atmospheric temperature becomes nearly a constant above this altitude.[109]
On Earth, the altitude of the exobase ranges from about 500 to 1,000 kilometres (310 to 620 mi) depending on solar activity.[110]
On 17 August 2015, based on studies with the Lunar Atmosphere and Dust Environment Explorer (LADEE) spacecraft, NASA reported the detection of neon in the exosphere of the moon.[106]
Ionospheres
[edit | edit source]
Def. the "part of the Earth's atmosphere beginning at an altitude of about 50 kilometers [31 miles] and extending outward 500 kilometers [310 miles] or more"[111] or the "similar region of the atmosphere of another planet"[112] is called the ionosphere.
Upon reaching the top of the mesosphere, the temperature starts to rise, but air pressure continues to fall. This is the beginning of the ionosphere, a region dominated by chemical ions. Many of them are the same chemicals such as nitrogen and oxygen in the atmosphere below, but an ever increasing number are hydrogen ions (protons) and helium ions. These can be detected by an ion spectrometer. The process of ionization removes one or more electrons from a neutral atom to yield a variety of ions depending on the chemical element species and incidence of sufficient energy to remove the electrons.
Earth
[edit | edit source]
"Earth is a blue planet"[113].
Atmospheric gases scatter blue light more than other wavelengths, giving the Earth a blue halo when seen from space. This is shown in the image at right.
When light passes through our atmosphere, photons interact with it through scattering. If the light does not interact with the atmosphere, it is called direct radiation and is what you see if you were to look directly at the Sun. Indirect radiation is light that has been scattered in the atmosphere. For example, on an overcast day when you cannot see your shadow there is no direct radiation reaching you, it has all been scattered. As another example, due to a phenomenon called Rayleigh scattering, shorter (blue) wavelengths scatter more easily than longer (red) wavelengths. This is why the sky looks blue; you are seeing scattered blue light. This is also why sunsets are red. Because the Sun is close to the horizon, the Sun's rays pass through more atmosphere than normal to reach your eye. Much of the blue light has been scattered out, leaving the red light in a sunset.
The image on the left is the famous Blue Marble image of Earth taken by Apollo 17. The image shows the eastern Southern Atlantic Ocean, the South African portion of the Southern Ocean, and the Western Indian Ocean. The land consists of most of Africa, Madagascar, Saudi Arabia, and portions of Iran, Irag, Turkey, and southern Greece. The gaseous portion consists of water vapor clouds over the southern portion of this hemisphere. Antarctica is completely covered in snow (a water-ice rocky substance).
If this image is the one chosen to decide whether the Earth is a dwarf gaseous object, a dwarf liquid object, or a dwarf rocky object, the decision becomes difficult.
Volcanoes
[edit | edit source]

Oblique images such as the one at right are taken by astronauts looking out from the ISS at an angle, rather than looking straight downward toward the Earth (a perspective called a nadir view), as is common with most remotely sensed data from satellites. An oblique view gives the scene a more three-dimension quality, and provides a look at the vertical structure of the volcanic plume. While much of the island is covered in green vegetation, grey deposits that include pyroclastic flows and volcanic mud-flows (lahars) are visible extending from the volcano toward the coastline. When compared to its extent in earlier views, the volcanic debris has filled in more of the eastern coastline. Urban areas are visible in the northern and western portions of the island; they are recognizable by linear street patterns and the presence of bright building rooftops. The silver-grey appearance of the Caribbean Sea surface is due to sun-glint, which is the mirror-like reflection of sunlight off the water surface back towards the hand-held camera on-board the ISS. The sun-glint highlights surface wave patterns around the island.
Auroras
[edit | edit source]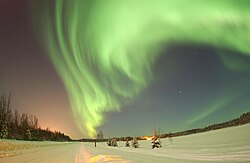

Computer simulations are usually used to represent auroras. The image at right shows a terrella in a laboratory experiment to produce auroras.
"Although auroras might first appear to be moonlit clouds, they only add light to the sky and do not block background stars from view. Called "Northern Lights" in the Northern Hemisphere, auroras are caused by collisions between charged particles from the magnetosphere and air molecules high in the Earth's atmosphere. If viewed from space, auroras can be seen to glow in X-ray and ultraviolet light as well. Predictable auroras might occur a few days after a powerful magnetic event has been seen on the sun."[114]
Most aurorae occur in a band known as the auroral zone,[115][116] which is typically 3° to 6° in latitudinal extent and at all local times or longitudes. The auroral zone is typically 10° to 20° from the magnetic pole defined by the axis of the Earth's magnetic dipole. During a geomagnetic storm, the auroral zone will expand to lower latitudes. The diffuse aurora is a featureless glow in the sky which may not be visible to the naked eye even on a dark night and defines the extent of the auroral zone. The discrete aurora are sharply defined features within the diffuse aurora which vary in brightness from just barely visible to the naked eye to bright enough to read a newspaper at night. Discrete aurorae are usually observed only in the night sky because they are as bright as the sunlit sky. Aurorae occasionally occur poleward of the auroral zone as diffuse patches[117] or arcs (polar cap arcs[118]), which are generally invisible to the naked eye.
Jet streams
[edit | edit source]
Def. any of the high-speed, high-altitude air currents that circle the Earth in a westerly direction is called a jet stream.
Jet streams are fast flowing, narrow air currents found in the atmospheres of some planets, including Earth. The main jet streams are located near the tropopause, the transition between the troposphere (where temperature decreases with altitude) and the stratosphere (where temperature increases with altitude).[119] The major jet streams on Earth are westerly winds (flowing west to east). Their paths typically have a meandering shape; jet streams may start, stop, split into two or more parts, combine into one stream, or flow in various directions including the opposite direction of most of the jet. The strongest jet streams are the polar jets, at around 7–12 km (23,000–39,000 ft) above sea level, and the higher and somewhat weaker subtropical jets at around 10–16 km (33,000–52,000 ft). The Northern Hemisphere and the Southern Hemisphere each have both a polar jet and a subtropical jet. The northern hemisphere polar jet flows over the middle to northern latitudes of North America, Europe, and Asia and their intervening oceans, while the southern hemisphere polar jet mostly circles Antarctica all year round.
Poles
[edit | edit source]"A polar vortex is a natural part of nature gone wrong: It starts off as a standard low-pressure system over the Arctic, but when the jet streams keeping these low-pressure systems in check weaken, their freezing cold winds can fall down right onto midwestern American. Beyond extreme discomfort and disruption of services, the freezing cold, which hit 32 below zero in parts of Illinois, is responsible for over 20 deaths."[120]
"NASA's Earth-observing Aqua satellite was able to capture [the] system's movement across the globe. Among Aqua's six instruments is its Atmospheric Infrared Sounder (AIRS), which measures temperature and water vapor as functions of height. Every temperature profile, also known as a sounding, is sensitive to temperature and water vapor. AIRS can measure these soundings using its 2,378 sensors-prior to its launch in 2002, [early] Earth-observing satellites only had 15 such sensors."[120]
"The coldest temperatures in this image are the purple and blue, which range from -40 degrees Fahrenheit (also -40 degrees Celsius) to -10 degrees Fahrenheit (-23 degrees Celsius)."[120]
Earth sciences
[edit | edit source]Def. the study of the atmosphere and its phenomena, especially with weather and weather forecasting is called meteorology.
Def. the study of the theoretical effects of astronomical bodies and forces on the Earth’s atmosphere and on the atmosphere of other astronomical objects is called astrometeorology.
Def. a system of winds rotating around a center of low atmospheric pressure, the more or less violent small-scale circulations such as tornadoes, waterspouts, and dust devils is called a cyclone.
Moon
[edit | edit source]"Where Earth's atmosphere merges into outer space, there is a cloud of hydrogen atoms called the geocorona."[121]
"A recent discovery based on observations by the ESA/NASA Solar and Heliospheric Observatory, SOHO, shows that the geocorona extends well beyond the orbit of the Moon, reaching up to 630 000 km above Earth's surface, or 50 times the diameter of our planet."[121]
See also
[edit | edit source]References
[edit | edit source]- ↑ 1.0 1.1 1.2 1.3 1.4 Tariq Malik (29 August 2021). "See Hurricane Ida from 1 million miles away in this NOAA satellite view". Live Science. Retrieved 31 August 2021.
- ↑ Brian Dunbar (30 August 2021). "See Hurricane Ida from 1 million miles away in this NOAA satellite view". Live Science. Retrieved 31 August 2021.
- ↑ Craig F. Bohren. Atmospheric Optics. http://homepages.wmich.edu/%7Ekorista/atmospheric_optics.pdf.
- ↑ cumulus. San Francisco, California: Wikimedia Foundation, Inc. February 8, 2013. http://en.wiktionary.org/wiki/cumulus. Retrieved 2013-02-17.
- ↑ cloud. San Francisco, California: Wikimedia Foundation, Inc. February 13, 2013. http://en.wiktionary.org/wiki/cloud. Retrieved 2013-02-18.
- ↑ Baffled Scientists Say Less Sunlight Reaching Earth. LiveScience. 2006-01-24. http://www.livescience.com/environment/060124_earth_albedo.html. Retrieved 2011-08-19.
- ↑ 7.0 7.1 7.2 7.3 Mark Fischetti (February 5, 2019). Warning Scale Unveiled for Dangerous Rivers in the Sky. Scientific American. https://www.scientificamerican.com/article/warning-scale-unveiled-for-dangerous-rivers-in-the-sky/. Retrieved 8 February 2019.
- ↑ 8.0 8.1 Martin Ralph (February 5, 2019). Warning Scale Unveiled for Dangerous Rivers in the Sky. Scientific American. https://www.scientificamerican.com/article/warning-scale-unveiled-for-dangerous-rivers-in-the-sky/. Retrieved 8 February 2019.
- ↑ E. C. Roelof, D. G. Mitchell, D. J. Williams (1985). "Energetic neutral atoms (E ∼ 50 keV) from the ring current: IMP 7/8 and ISEE 1". Journal of Geophysical Research 90 (A11): 10,991-11,008. doi:10.1029/JA090iA11p10991. http://www.agu.org/pubs/crossref/1985/JA090iA11p10991.shtml. Retrieved 2012-08-12.
- ↑ D. G. Mitchell, K. C. Hsieh, C. C. Curtis, D. C. Hamilton, H. D. Voes, E. C, Roelof, P. C:son-Brandt (2001). "Imaging two geomagnetic storms in energetic neutral atoms". Geophysical Research Letters 28 (6): 1151-4. doi:10.1029/2000GL012395. http://www.agu.org/pubs/crossref/2001/2000GL012395.shtml. Retrieved 2012-08-12.
- ↑ Mae Devoe Lethbridge (1990). "Thunderstorms, cosmic rays, and solar-lunar influences". Journal of Geophysical Research 95 (D9): 13,645-9. doi:10.1029/JD095iD09p13645. http://www.agu.org/pubs/crossref/1990/JD095iD09p13645.shtml. Retrieved 2012-08-22.
- ↑ Tascione, Thomas F. (1994). Introduction to the Space Environment, 2nd. Ed.. Malabar, Florida USA: Kreiger Publishing CO.. ISBN 0-89464-044-5.
- ↑ Adriani, O.; Barbarino, G. C.; Bazilevskaya, G. A.; Bellotti, R.; Boezio, M.; Bogomolov, E. A.; Bongi, M.; Bonvicini, V. et al. (2011). "The Discovery of Geomagnetically Trapped Cosmic-Ray Antiprotons". The Astrophysical Journal Letters 737 (2): L29. doi:10.1088/2041-8205/737/2/L29.
- ↑ 14.0 14.1 G. Barbiellini, G. Basini, R. Bellotti, M. Bpcciolini, M. Boezio, F. Massimo Brancaccio, U. Bravar, F. Cafagna, M. Candusso, P. Carlson, M. Casolino, M. Castellano, M. Circella, A. Codino, G. De Cataldo, C. De Marzo, M.P. De Pascale, N. Finetti, T. Francke, N. Giglietto, R.L. Golden, C. Grimani, M. Hof, B. Marangelli, W. Menn, J.W. Mitchell, A. Morselli, J.F. Ormes, P. Papini, a. Perego, S. Piccardi, P. Picozza, M. Ricci, P. Schiavon, M. Simon, R. Sparvoli, P. Spillatini, P. Spinelli, S.A. Stephens, S.J. Stochaj, R.E. Streitmatter, M. Suffert, A. Vacchi, N. Weber, and N. Zampa (May 1996). "The cosmic-ray positron-to-electron ratio in the energy range 0.85 to 14 GeV". Astronomy and Astrophysics 309 (05): L15-8. http://adsabs.harvard.edu/abs/1996A&A...309L..15B. Retrieved 2013-08-11.
- ↑ 15.0 15.1 15.2 15.3 Space Radiation Storm. NASA. 2004-07-14. http://science.nasa.gov/headlines/y2000/ast14jul_2m.htm. Retrieved 2007-03-09.
- ↑ Associated Press (2000-07-14). NASA Says Solar Flare Caused Radio Blackouts. The New York Times. http://docs.newsbank.com/openurl?ctx_ver=z39.88-2004&rft_id=info:sid/iw.newsbank.com:AWNB:NYTB&rft_val_format=info:ofi/fmt:kev:mtx:ctx&rft_dat=10192EC724742FDF&svc_dat=InfoWeb:aggregated4&req_dat=0D0CB57AB53DF815. Retrieved 2007-03-09.
- ↑ 17.0 17.1 Frank D. Roylance (2000-07-15). Solar flare biggest since '89. Contra Costa Times. http://docs.newsbank.com/openurl?ctx_ver=z39.88-2004&rft_id=info:sid/iw.newsbank.com:AWNB:CCYB&rft_val_format=info:ofi/fmt:kev:mtx:ctx&rft_dat=1064A3E55B815DA8&svc_dat=InfoWeb:aggregated4&req_dat=0D0CB57AB53DF815. Retrieved 2007-03-09.
- ↑ D. Fargion, P.G. De Sanctis Lucentini, M. De Santis. M.Grossi (10 2004). "Tau Air Showers from Earth". The Astrophysical Journal 613 (2): 1285-1301. doi:10.1086/423124. https://arxiv.org/pdf/hep-ph/0305128. Retrieved 2018-4-04.
- ↑ 19.0 19.1 19.2 19.3 19.4 Paolo Strolin (August 15, 2013). The secret life of volcanoes: using muon radiography. EIROforum. http://www.scienceinschool.org/2013/issue27/muons. Retrieved 2014-03-22.
- ↑ M. Ackermann, M. Ajello, A. Allafort, W. B. Atwood, L. Baldini, G. Barbiellini, D. Bastieri, K. Bechtol, R. Bellazzini, B. Berenji, R. D. Blandford, E. D. Bloom, E. Bonamente, A. W. Borgland, A. Bouvier, J. Bregeon, M. Brigida, P. Bruel, R. Buehler, S. Buson, G. A. Caliandro, R. A. Cameron, P. A. Caraveo, J. M. Casandjian, C. Cecchi, E. Charles, A. Chekhtman, C. C. Cheung, J. Chiang, S. Ciprini, R. Claus, J. Cohen-Tanugi, J. Conrad, S. Cutini, A. de Angelis, F. de Palma, C. D. Dermer, S. W. Digel, E. do Couto e Silva, P. S. Drell, A. Drlica-Wagner, C. Favuzzi, S. J. Fegan, E. C. Ferrara, W. B. Focke, P. Fortin, Y. Fukazawa, S. Funk, P. Fusco, F. Gargano, D. Gasparrini, S. Germani, N. Giglietto, P. Giommi, F. Giordano, M. Giroletti, T. Glanzman, G. Godfrey, I. A. Grenier, J. E. Grove, S. Guiriec, M. Gustafsson, D. Hadasch, A. K. Harding, M. Hayashida, R. E. Hughes, G. Jóhannesson, A. S. Johnson, T. Kamae, H. Katagiri, J. Kataoka, J. Knǒdlseder, M. Kuss, J. Lande, L. Latronico, M. Lemoine-Goumard, M. Llena Garde, F. Longo, F. Loparco, M. N. Lovellette, P. Lubrano, G. M. Madejski, M. N. Mazziotta, J. E. McEnery, P. F. Michelson, W. Mitthumsiri, T. Mizuno, A. A. Moiseev, C. Monte, M. E. Monzani, A. Morselli, I. V. Moskalenko, S. Murgia, T. Nakamori, P. L. Nolan, J. P. Norris, E. Nuss, M. Ohno, T. Ohsugi, A. Okumura, N. Omodei, E. Orlando, J. F. Ormes, M. Ozaki, D. Paneque, D. Parent, M. Pesce-Rollins, M. Pierbattista, F. Piron, G. Pivato, T. A. Porter, S. Rainò, R. Rando, M. Razzano, S. Razzaque, A. Reimer, O. Reimer, T. Reposeur, S. Ritz, R. W. Romani, M. Roth, H. F.-W. Sadrozinski, C. Sbarra, T. L. Schalk, C. Sgrò, E. J. Siskind, G. Spandre, P. Spinelli, A. W. Strong, H. Takahashi, T. Takahashi, T. Tanaka, J. G. Thayer, J. B. Thayer, L. Tibaldo, M. Tinivella, D. F. Torres, G. Tosti, E. Troja, Y. Uchiyama, T. L. Usher, J. Vandenbroucke, V. Vasileiou, G. Vianello, V. Vitale, A. P. Waite, B. L. Winer, K. S. Wood, M. Wood, Z. Yang, and S. Zimmer (2012). "Measurement of separate cosmic-ray electron and positron spectra with the Fermi Large Area Telescope". Physical Review Letters 108 (1): e011103. http://prl.aps.org/abstract/PRL/v108/i1/e011103. Retrieved 2014-01-31.
- ↑ http://news.nationalgeographic.com/news/2011/01/110111-thunderstorms-antimatter-beams-fermi-radiation-science-space/
- ↑ http://www.nasa.gov/mission_pages/GLAST/news/fermi-thunderstorms.html
- ↑ S. Wolpert (July 24, 2008). Scientists solve 30-year-old aurora borealis mystery. University of California. http://www.universityofcalifornia.edu/news/article/18277. Retrieved 2008-10-11.
- ↑ Barry H. Mauk and Joachim Saur (October 26, 2007). "Equatorial electron beams and auroral structuring at Jupiter". Journal of Geophysical Research 112 (A10221): 20. doi:10.1029/2007JA012370. http://www.igpp.ucla.edu/public/mkivelso/refs/PUBLICATIONS/Mauk2007JA012370.pdf. Retrieved 2012-06-02.
- ↑ Signature Of Antimatter Detected In Lightning - Science News
- ↑ U.S. Inan, S.C. Reising, G.J. Fishman, and J.M. Horack. On the association of terrestrial gamma-ray bursts with lightning and implications for sprites. Geophysical Research Letters, 23(9):1017-20, May 1996. As quoted by elf.gi.alaska.edu Retrieved 2007-03-06.
- ↑ Łukasz Staszewski (2009). "Lightning Phenomenon – Introduction and Basic Information to Understand the Power of Nature". Environment and Electrical Engineering: 4. http://eeeic.org/proc/papers/52.pdf. Retrieved 5 February 2019.
- ↑ Steven Cummer (2009). "Lightning Phenomenon – Introduction and Basic Information to Understand the Power of Nature". Environment and Electrical Engineering: 4. http://eeeic.org/proc/papers/52.pdf. Retrieved 5 February 2019.
- ↑ http://news.nationalgeographic.com/news/2011/01/110111-thunderstorms-antimatter-beams-fermi-radiation-science-space/
- ↑ Manuel Güdel (September 2004). "X-ray astronomy of stellar coronae". The Astronomy and Astrophysics Review 12 (2-3): 71–237. doi:10.1007/s00159-004-0023-2.
- ↑ A. Bhardwaj & R. Elsner (February 20, 2009). Earth Aurora: Chandra Looks Back At Earth. Cambridge, MA, USA: Harvard-Smithsonian Center for Astrophysics. http://chandra.harvard.edu/photo/2005/earth/. Retrieved 2013-05-10.
- ↑ Ruth Netting (March 22, 2011). ULTRAVIOLET LIGHT FROM OUR SUN. Washington, DC USA: NASA. http://missionscience.nasa.gov/ems/10_ultravioletwaves.html. Retrieved 2013-05-29.
- ↑ Far Ultraviolet Camera/Spectrograph
- ↑ D. Reeves (1936). "Aerial photography and archaeology". American Antiquity 2 (2): 102-7. http://www.jstor.org/stable/275881.
- ↑ 35.0 35.1 35.2 35.3 35.4 Steve Cole and Rob Gutro (20 July 2015). NASA Satellite Camera Provides “EPIC” View of Earth. Washington, DC USA: NASA. http://www.nasa.gov/press-release/nasa-satellite-camera-provides-epic-view-of-earth. Retrieved 2015-12-09.
- ↑ Charlie Bolden (20 July 2015). NASA Satellite Camera Provides “EPIC” View of Earth. Washington, DC USA: NASA. http://www.nasa.gov/press-release/nasa-satellite-camera-provides-epic-view-of-earth. Retrieved 2015-12-09.
- ↑ 37.0 37.1 Adam Szabo (20 July 2015). NASA Satellite Camera Provides “EPIC” View of Earth. Washington, DC USA: NASA. http://www.nasa.gov/press-release/nasa-satellite-camera-provides-epic-view-of-earth. Retrieved 2015-12-09.
- ↑ 212.159.113.112 (6 May 2003). atmosphere. San Francisco, California: Wikimedia Foundation, Inc. https://en.wiktionary.org/wiki/atmosphere. Retrieved 2016-02-06.
- ↑ Dan Burbank (December 21, 2011). Love and Joy for the New Year. International Space Station: NASA's Earth Observatory. http://www.flickr.com/photos/nasaearthobservatory/6620255181/. Retrieved 2012-07-22.
- ↑ Les Cowley. Earth's shadow. www.atoptics.co.uk. http://www.atoptics.co.uk/atoptics/earshad.htm.
- ↑ 41.0 41.1 What causes layers in the sunrise and sunset?. earthsky.org. http://www.webexhibits.org/causesofcolor/14E.html.
- ↑ 42.0 42.1 42.2 42.3 42.4 Warren Wiscombe (August 19, 2009). First IR Image from Newest Weather Satellite Captures Hurricane Bill. NASA/NOAA. http://earthobservatory.nasa.gov/IOTD/view.php?id=39848. Retrieved 2012-11-26.
- ↑ NASAGalileo (March 14, 2003). Earth - Galileo Raw image of Earth showing Antarctica and Africa. Greenbelt, Maryland USA: NASA Goddard Space Flight Center. http://nssdc.gsfc.nasa.gov/imgcat/html/object_page/gal_0061520300.html. Retrieved 2013-04-01.
- ↑ gas. San Francisco, California: Wikimedia Foundation, Inc. September 19, 2013. https://en.wiktionary.org/wiki/gas. Retrieved 2013-10-05.
- ↑ Lee S. Langston (July-August 2013). "The Adaptable Gas Turbine". American Scientist. http://www.americanscientist.org/issues/pub/2013/4/the-adaptable-gas-turbine. Retrieved 2013-10-05.
- ↑ gaseous. San Francisco, California: Wikimedia Foundation, Inc. September 29, 2013. https://en.wiktionary.org/wiki/gaseous. Retrieved 2013-10-05.
- ↑ P. Amaro-Seoane and R. Spurzem (2001). J. H. Knapen. ed. Gas in the Central Regions of AGN: The Interstellar Medium and Supermassive Gaseous Objects, In: The Central Kiloparsec of Starbursts and AGN: The La Palma Connection. 249. San Francisco, California USA: Astronomical Society of the Pacific. pp. 731-4. ISBN 1-58381-089-7. Bibcode: 2001ASPC..249..731A. http://adsabs.harvard.edu/abs/2001ASPC..249..731A. Retrieved 2013-07-16.
- ↑ 48.0 48.1 Wallace, John M. and Peter V. Hobbs. Atmospheric Science: An Introductory Survey. Elsevier. Second Edition, 2006. ISBN 978-0-12-732951-2. Chapter 1
- ↑ "Trace Gases". Ace.mmu.ac.uk. Retrieved 2010-10-16.
- ↑ Source for figures: Carbon dioxide, NOAA Earth System Research Laboratory, (updated 2013-03). Methane, Intergovernmental Panel on Climate Change (IPCC) |url=https://web.archive.org/web/20070615161122/http://www.grida.no/climate/ipcc_tar/wg1/221.htm |date=2007-06-15 }}
- ↑ Vaughan, Adam (2015-05-06). "Global carbon dioxide levels break 400ppm milestone". The Guardian. ISSN 0261-3077. Retrieved 2016-12-25.
- ↑ "Air Composition". The Engineering ToolBox. Retrieved 2017-07-04.
The composition of air is unchanged until elevation of approximately 10.000 m
- ↑ 53.0 53.1 53.2 Exline, Joseph D.; Levine, Arlene S.; Levine, Joel S. (2006). Meteorology: An Educator's Resource for Inquiry-Based Learning for Grades 5-9. NASA/Langley Research Center. p. 6. https://www.nasa.gov/pdf/288978main_Meteorology_Guide.pdf.
- ↑ 54.0 54.1 Williams, David R. (16 March 2017). "Earth Fact Sheet". NASA/Goddard Space Flight Center. Retrieved 26 July 2018.
- ↑ 55.0 55.1 55.2 Staff (8 October 2003). "Earth's Atmosphere". NASA. Retrieved 21 March 2007.
- ↑ Pidwirny, Michael (2006). "Fundamentals of Physical Geography (2nd Edition)". PhysicalGeography.net. Retrieved 19 March 2007.
- ↑ St. Fleur, Nicholas (19 May 2017). "Spotting Mysterious Twinkles on Earth From a Million Miles Away". The New York Times. Retrieved 20 May 2017.
- ↑ Marshak, Alexander; Várnai, Tamás; Kostinski, Alexander (15 May 2017). "Terrestrial glint seen from deep space: oriented ice crystals detected from the Lagrangian point". Geophysical Research Letters 44 (10): 5197–5202. doi:10.1002/2017GL073248.
- ↑ Harrison, Roy M.; Hester, Ronald E. (2002). Causes and Environmental Implications of Increased UV-B Radiation. Royal Society of Chemistry. ISBN 978-0-85404-265-4.
- ↑ "Atmospheric Temperature Trends, 1979–2005 : Image of the Day". Earthobservatory.nasa.gov. 2000-01-01. Retrieved 2014-06-10.
- ↑ Trenberth, Kevin E.; Smith, Lesley (1970-01-01). "The Mass of the Atmosphere: A Constraint on Global Analyses". Journal of Climate 18 (6): 864. doi:10.1175/JCLI-3299.1.
- ↑ Beck, Hylke E.; Zimmermann, Niklaus E.; McVicar, Tim R.; Vergopolan, Noemi; Berg, Alexis; Wood, Eric F. (30 October 2018). "Present and future Köppen-Geiger climate classification maps at 1-km resolution". Scientific Data 5: 180214. doi:10.1038/sdata.2018.214. ISSN 2052-4463. PMID 30375988. PMC 6207062. https://www.nature.com/articles/sdata2018214.
- ↑ Dmol (14 March 2005). tropical. San Francisco, California: Wikimedia Foundation, Inc. https://en.wiktionary.org/wiki/tropical. Retrieved 13 February 2019.
- ↑ 144.82.194.13 (10 March 2005). weather. San Francisco, California: Wikimedia Foundation, Inc. https://en.wiktionary.org/wiki/weather. Retrieved 13 February 2019.
- ↑ "Updated world Koppen-Geiger climate classification map" (PDF).
- ↑ Elisabeth M. Benders-Hyde (2003). World Climates. Blue Planet Biomes. Retrieved on 2008-12-27.
- ↑ J . S. 0guntoyinbo and F. 0. Akintola (1983). Rainstorm characteristics affecting water availability for agriculture, In: IAHS Publication Number 140. https://web.archive.org/web/20090205200119/http://www.cig.ensmp.fr/~iahs/redbooks/a140/iahs_140_0063.pdf. Retrieved 2008-12-27.
- ↑ 68.0 68.1 68.2 McKnight, Tom L; Hess, Darrel (2000). "Climate Zones and Types". Physical Geography: A Landscape Appreciation. Upper Saddle River, NJ: Pretice Hall. pp. 205–8. ISBN 978-0-13-020263-5.
- ↑ Peel, M. C.; B. L. Finlayson; T. A. McMahon (2007). "Updated world map of the Köppen-Geiger climate classification". Hydrology and Earth System Sciences 11 (5): 1633–1644. doi:10.5194/hess-11-1633-2007. http://www.hydrol-earth-syst-sci.net/11/1633/2007/hess-11-1633-2007.pdf.
- ↑ Laity, Julie J. (2009). Deserts and Desert Environments. John Wiley & Sons. p. 7. ISBN 978-1444300741. https://books.google.com/books?id=wtAbzLLTcwcC&pg=PR5.
- ↑ Peel, M. C.; B. L. Finlayson; T. A. McMahon (2007). "Updated world map of the Köppen-Geiger climate classification". Hydrology and Earth System Sciences 11 (5): 1633–1644. doi:10.5194/hess-11-1633-2007. http://www.hydrol-earth-syst-sci.net/11/1633/2007/hess-11-1633-2007.pdf.
- ↑ Geometric altitude vs. temperature, pressure, density, and the speed of sound derived from the 1962 U.S. Standard Atmosphere.
- ↑ "ISS022-E-062672 caption". NASA. Retrieved 21 September 2012.
- ↑ Lutgens, Frederick K. and Edward J. Tarbuck (1995) The Atmosphere, Prentice Hall, 6th ed., pp. 14–17, isbn=0-13-350612-6 }}
- ↑ Zell, Holly (2015-03-02). "Earth's Upper Atmosphere". NASA. Retrieved 2017-02-20.
- ↑ Randy Russell (2008). "The Thermosphere". Retrieved 2013-10-18.
- ↑ 77.0 77.1 "The height of the tropopause". Das.uwyo.edu. Retrieved 2012-04-18.
- ↑ Layers of the Atmosphere, In: JetStream, the National Weather Service Online Weather School. National Weather Service. http://www.srh.noaa.gov/srh/jetstream/atmos/layers.htm. Retrieved 22 December 2005.
- ↑ troposphere. San Francisco, California: Wikimedia Foundation, Inc. January 13, 2013. http://en.wiktionary.org/wiki/troposphere. Retrieved 2013-02-21.
- ↑ Barry, R.G.; Chorley, R.J. (1971). Atmosphere, Weather and Climate. London: Menthuen & Co Ltd.. p. 65.
- ↑ Tyson, P.D.; Preston-Whyte, R.A. (2013). The Weather and Climate of Southern Africa (2nd ed.). Oxford: Oxford University Press. p. 4.
- ↑ Concise Encyclopedia of Science & Technology. McGraw-Hill. 1984.
- ↑ 83.0 83.1 83.2 Danielson; Levin; Abrams (2003). Meteorology. McGraw Hill.
- ↑ Landau and Lifshitz, Fluid Mechanics, Pergamon, 1979
- ↑ [1]
- ↑ Landau and Lifshitz, Statistical Physics Part 1, Pergamon, 1980
- ↑ Kittel and Kroemer, Thermal Physics, Freeman, 1980; chapter 6, problem 11
- ↑ Paul E. Lydolph (1985). "The Climate of the Earth". Rowman and Littlefield Publishers Inc. p. 12.
- ↑ "American Meteorological Society Glossary – Zonal Flow". Allen Press Inc. June 2000. Retrieved 2006-10-03.
- ↑ "American Meteorological Society Glossary – Meridional Flow". Allen Press Inc. June 2000. Retrieved 2006-10-03.
- ↑ "Meteorology – MSN Encarta, "Energy Flow and Global Circulation"". Encarta.Msn.com. Retrieved 2006-10-13.
- ↑ Hoinka, K. P. (1999). "Temperature, Humidity, and Wind at the Global Tropopause". Monthly Weather Review (American Meteorological Society) 127: 2248–2265. doi:10.1175/1520-0493(1999)127<2248:THAWAT>2.0.CO;2.
- ↑ Gettelman, A.; Salby, M. L.,; Sassi, F. (2002). "Distribution and influence of convection in the tropical tropopause region". Journal of Geophysical Research (American Geophysical Union) 107. doi:10.1029/2001JD001048.
- ↑ tropopause,. San Francisco, California: Wikimedia Foundation, Inc. October 15, 2012. http://en.wiktionary.org/wiki/tropopause. Retrieved 2013-02-21.
- ↑ International Meteorological Vocabulary (2nd ed.). Geneva: Secretariat of the World Meteorological Organization. 1992. p. 636. ISBN 92-63-02182-1.
- ↑ Panchev, Stoǐcho (1985). Dynamic meteorology. D. Reidel Publishing Company. ISBN 90-277-1744-3.
- ↑ stratosphere. San Francisco, California: Wikimedia Foundation, Inc. February 10, 2013. http://en.wiktionary.org/wiki/stratosphere. Retrieved 2013-02-21.
- ↑ Joel7687 (17 September 2005). mesosphere. San Francisco, California: Wikimedia Foundation, Inc. https://en.wiktionary.org/wiki/mesosphere. Retrieved 2013-02-21.
- ↑ States, Robert J.; Gardner, Chester S. (January 2000). "Thermal Structure of the Mesopause Region (80–105 km) at 40°N Latitude. Part I: Seasonal Variations". Journal of the Atmospheric Sciences 57 (1): 66–77. doi:10.1175/1520-0469(2000)057<0066:TSOTMR>2.0.CO;2.
- ↑ Joe Buchdahl. "Atmosphere, Climate & Environment Information Programme". Ace.mmu.ac.uk. Retrieved 2012-04-18.
- ↑ Joel7687 (17 September 2006). thermosphere. San Francisco, California: Wikimedia Foundation, Inc. https://en.wiktionary.org/wiki/thermosphere. Retrieved 2013-02-21.
- ↑ Duxbury & Duxbury. Introduction to the World's Oceans. 5ed. (1997)
- ↑ Ahrens, C. Donald. Essentials of Meteorology. Published by Thomson Brooks/Cole, 2005.
- ↑ SemperBlotto (22 November 2008). exosphere. San Francisco, California: Wikimedia Foundation, Inc. https://en.wiktionary.org/wiki/exosphere. Retrieved 2013-02-21.
- ↑ Kwamikagami (5 March 2012). exosphere. San Francisco, California: Wikimedia Foundation, Inc. https://en.wiktionary.org/wiki/exosphere. Retrieved 2013-02-21.
- ↑ 106.0 106.1 Steigerwald, William (17 August 2015). "NASA's LADEE Spacecraft Finds Neon in Lunar Atmosphere". NASA. Retrieved 18 August 2015.
- ↑ Day, Brian (20 August 2013). "Why LADEE Matters". NASA Ames Research Center. Retrieved 19 April 2015.
- ↑ "Is There an Atmosphere on the Moon?". NASA. 30 January 2014. Retrieved 4 August 2016.
- ↑ Bauer, Siegfried; Lammer, Helmut. Planetary Aeronomy: Atmosphere Environments in Planetary Systems, Springer Publishing, 2004.
- ↑ "Exosphere - overview". UCAR. 2011. Retrieved April 19, 2015.
- ↑ CORNELIUSSEON (11 June 2006). ionosphere. San Francisco, California: Wikimedia Foundation, Inc. https://en.wiktionary.org/wiki/ionosphere. Retrieved 2012-09-20.
- ↑ RJFJR (15 November 2008). ionosphere. San Francisco, California: Wikimedia Foundation, Inc. https://en.wiktionary.org/wiki/ionosphere. Retrieved 2012-09-20.
- ↑ AT Young (May 1985). "What color is the solar system?". Sky and Telescope 69 (5): 399-402. http://adsabs.harvard.edu/abs/1985S&T....69..399Y. Retrieved 2012-06-19.
- ↑ Samantha Harvey (September 28, 2011). Aurora Over Norway. NASA. http://solarsystem.nasa.gov/multimedia/display.cfm?Category=GreatShots&IM_ID=12648. Retrieved 2012-07-21.
- ↑ Feldstein, Y. I. (1963). "Some problems concerning the morphology of auroras and magnetic disturbances at high latitudes". Geomagnetism and Aeronomy 3: 183–192.
- ↑ Feldstein, Y. I. (1986). "A Quarter Century with the Auroral Oval". EOS 67 (40): 761. doi:10.1029/EO067i040p00761-02.
- ↑ E. J. Weber et al. (1984). "F layer ionization patches in the polar cap". J. Geophys. Res. 89 (A3): 1683–94. doi:10.1029/JA089iA03p01683.
- ↑ Frank, L. A. et al. (1986). "The theta aurora". J. Geophys. Res. 91 (A3): 3177–3224. doi:10.1029/JA091iA03p03177.
- ↑ United States Department of Energy (26 June 2002). Ask a Scientist. http://www.newton.dep.anl.gov/askasci/wea00/wea00135.htm. Retrieved 5 May 2008.
- ↑ 120.0 120.1 120.2 David Grossman (February 1, 2019). "The Polar Vortex Looks Absolutely Chilling From Space". Popular Mechanics: 1. https://news.yahoo.com/polar-vortex-looks-absolutely-chilling-220600844.html?.tsrc=daily_mail&uh_test=1_07. Retrieved 4 February 2019.
- ↑ 121.0 121.1 ESA (20 February 2019). The extent of Earth's geocorona. European Space Agency. http://sci.esa.int/soho/61131-the-extent-of-earth-s-geocorona/. Retrieved 22 February 2019.