Gases/Gaseous objects/Uranus

Uranus is a gaseous object in orbit around the Sun at a distance of less than one light-year.
Picture dictionary | |
---|---|
|
Jupiter and Saturn are systematically closer to the Sun, and Neptune is systematically further from the Sun.
Def. any "collection of heavenly bodies including a star or binary star, and any lighter stars, brown dwarfs, planets, and other objects in orbit"[1] is called a solar system.
Usage notes
- "As Sol is the name of our star, this phrase is usually used to refer specifically to our own sun and planets (the Sol system), in which case it is used with the and generally capitalised (as the Solar system or the Solar System). Other systems are then known as star systems or planetary systems, or specified by the name of the individual star (the Alpha Centauri system)."[1]
Planets "of the Solar System [are] Mercury, Venus, Earth, Mars, Jupiter, Saturn, Uranus]], Neptune".[1]
Theory of Uranus
[edit | edit source]Here's a theoretical definition:
Def. the "seventh planet in our solar system,[2] is called Uranus.
A "huge rock, possibly the size of a small planet, [may have] collided with Uranus, causing it to tilt dramatically, affecting its spin, its magnetic field, and even its heat distribution."[3]
"Along with affecting the planet's tilt, the impact event may have also spurred on the development of Uranus's thick icy outer layer, which keeps the heat from planet's core locked inside. Uranus is the only planet in our solar system that doesn't leak heat from its core, reaching temperatures of -371 degrees Fahrenheit at some of its chilliest points."[4]
"The impact likely caused Uranus's larger moons and rings, which orbit in line with its rotation, to gain their unique path as well."[4]
Electromagnetics
[edit | edit source]

"The discovery of [Uranus]'s non-dipolar, non-axisymmetric magnetic [field at the right] destroyed the picture-established by Earth, Jupiter and Saturn-that planetary magnetic fields are dominated by axial dipoles."[5]
"Planetary magnetic fields are generated by complex fluid motions in electrically conducting regions of the planets (a process known as dynamo action), and so are intimately linked to the structure and evolution of planetary interiors."[5]
Three-dimensional "numerical dynamo simulations [...] model the dynamo source region as a convecting thin shell surrounding a stably stratified fluid interior."[5]
This "convective-region geometry produces magnetic fields similar in morphology to [that] of Uranus [The field is] non-dipolar and non-axisymmetric, and [results] from a combination of the stable fluid's response to electromagnetic stress and the small length scales imposed by the thin shell."[5]
The planet had "a strong planetary magnetic field of Uranus and an associated magnetosphere and fully developed bipolar magnetotail [and a] detached bow shock wave [which] was observed upstream at 23.7 Uranus radii (1 RU = 25,600 km) and the magnetopause boundary at 18.0 RU. [The] maximum magnetic field of 413 nanotesla was observed at 4.19 RU [The] planetary magnetic field is well represented by that of a dipole offset from the center of the planet by 0.3 RU. The angle between Uranus' angular momentum vector and the dipole moment vector has the surprisingly large value of 60 degrees. [The] field of Uranus may be described as that of an oblique rotator. The dipole moment of 0.23 gauss R3U, combined with the large spatial offset, leads to minimum and maximum magnetic fields on the surface of the planet of approximately 0.1-1.1 gauss. The rotation period of the magnetic field and [that] of the interior of the planet is estimated to be 17.29±0.10 [hr]."[6]
Voyager's observations revealed that the magnetic field is peculiar, both because it does not originate from the planet's geometric center, and because it is tilted at 59° from the axis of rotation.[6][7] In fact the magnetic dipole is shifted from the center of the planet towards the south rotational pole by as much as one third of the planetary radius.[6] This unusual geometry results in a highly asymmetric magnetosphere, where the magnetic field strength on the surface in the southern hemisphere can be as low as 0.1 gauss (10 µT), whereas in the northern hemisphere it can be as high as 1.1 gauss (110 µT).[6] The average field at the surface is 0.23 gauss (23 µT).[6] In comparison, the magnetic field of Earth is roughly as strong at either pole, and its "magnetic equator" is roughly parallel with its geographical equator.[7] The dipole moment of Uranus is 50 times that of Earth.[6][7] Neptune has a similarly displaced and tilted magnetic field, suggesting that this may be a common feature of ice giants.[7] One hypothesis is that, unlike the magnetic fields of the terrestrial and gas giants, which are generated within their cores, the ice giants' magnetic fields are generated by motion at relatively shallow depths, for instance, in the water–ammonia ocean.[8][9]
Despite its curious alignment, in other respects the Uranian magnetosphere is like those of other planets: it has a bow shock located at about 23 Uranian radii ahead of it, a magnetopause at 18 Uranian radii, a fully developed magnetotail and radiation belts.[6][7][10] Overall, the structure of Uranus's magnetosphere is different from Jupiter's and more similar to Saturn's.[6][7] Uranus's magnetotail trails behind the planet into space for millions of kilometers and is twisted by the planet's sideways rotation into a long corkscrew.[6][11]
Uranus's magnetosphere contains charged particles: protons and electrons with small amount of H2+ ions.[7][10] No heavier ions have been detected. Many of these particles probably derive from the hot atmospheric corona.[10] The ion and electron energies can be as high as 4 and 1.2 megaelectronvolts, respectively.[10] The density of low energy (below 1 kiloelectronvolt) ions in the inner magnetosphere is about 2 cm−3.[12] The particle population is strongly affected by the Uranian moons that sweep through the magnetosphere leaving noticeable gaps.[10] The particle flux is high enough to cause darkening or space weathering of the moon’s surfaces on an astronomically rapid timescale of 100,000 years.[10] This may be the cause of the uniformly dark colouration of the moons and rings.[13] Uranus has relatively well developed aurorae, which are seen as bright arcs around both magnetic poles.[14] Unlike Jupiter's, Uranus's aurorae seem to be insignificant for the energy balance of the planetary thermosphere.[15]
Meteors
[edit | edit source]
Uranus has a complex, layered cloud structure, with methane thought to make up the uppermost layer of clouds.[16] With a large telescope of 25 cm or wider, cloud patterns may be visible.[17] When Voyager 2 flew by Uranus in 1986, it observed a total of ten cloud features across the entire planet.[18] Besides the large-scale banded structure, Voyager 2 observed ten small bright clouds, most lying several degrees to the north from the collar.[18]
In the 1990s, the number of the observed bright cloud features grew considerably partly because new high resolution imaging techniques became available.[19] Most were found in the northern hemisphere as it started to become visible.[19] An early explanation - that bright clouds are easier to identify in the dark part of the planet, whereas in the southern hemisphere the bright collar masks them - was shown to be incorrect: the actual number of features has indeed increased considerably.[20][21] Nevertheless there are differences between the clouds of each hemisphere. The northern clouds are smaller, sharper and brighter.[21] They appear to lie at a higher altitude.[21] The lifetime of clouds spans several orders of magnitude. Some small clouds live for hours, while at least one southern cloud may have persisted since Voyager flyby.[19] Recent observation also discovered that cloud features on Uranus have a lot in common with those on Neptune.[19] For example, the dark spots common on Neptune had never been observed on Uranus before 2006, when the first such feature dubbed Uranus Dark Spot was imaged.[22] The speculation is that Uranus is becoming more Neptune-like during its equinoctial season.[23]
For a short period from March to May 2004, a number of large clouds appeared in the Uranian atmosphere, giving it a Neptune-like appearance.[21][24]
On August 23, 2006, researchers at the Space Science Institute (Boulder, CO) and the University of Wisconsin observed a dark spot on Uranus's surface, giving astronomers more insight into the planet's atmospheric activity.[22]
The bright collar at −45° latitude is also connected with methane clouds.[25] Other changes in the southern polar region can be explained by changes in the lower cloud layers.[25]
The wind speeds on Uranus can reach 250 meters per second (900 km/h, 560 mph).[19] The tracking of numerous cloud features allowed determination of zonal winds blowing in the upper troposphere of Uranus.[19] At the equator winds are retrograde, which means that they blow in the reverse direction to the planetary rotation. Their speeds are from −100 to −50 m/s.[19][26] Wind speeds increase with the distance from the equator, reaching zero values near ±20° latitude, where the troposphere's temperature minimum is located.[19][27] Closer to the poles, the winds shift to a prograde direction, flowing with the planet's rotation. Windspeeds continue to increase reaching maxima at ±60° latitude before falling to zero at the poles.[19] Windspeeds at −40° latitude range from 150 to 200 m/s. Since the collar obscures all clouds below that parallel, speeds between it and the southern pole are impossible to measure.[19] In contrast, in the northern hemisphere maximum speeds as high as 240 m/s are observed near +50 degrees of latitude.[19][26][28] Observations included record-breaking wind speeds of 229 m/s (824 km/h) and a persistent thunderstorm referred to as "Fourth of July fireworks".[29]
Rocks
[edit | edit source]"Models of Uranus and Neptune are computed based on the assumption that these planets consist of three layers: a rock core, an ice shell, and an atmosphere."[30]
Lightnings
[edit | edit source]The extreme pressure and temperature deep within Uranus may break up the methane molecules, with the carbon atoms condensing into crystals of diamond that rain down through the mantle like hailstones.[31][32][33] Very-high-pressure experiments at the Lawrence Livermore National Laboratory suggest that the base of the mantle may comprise an ocean of liquid diamond, with floating solid 'diamond-bergs'.[34][35] Scientists also believe that lightning storms make rainfalls of solid diamonds occur on Uranus, as well as on Jupiter, Saturn, and Neptune.[36][37]
Cosmic rays
[edit | edit source]"[F]or the regions of the giant planets, especially Uranus and Neptune, ... ionization is due mainly to cosmic rays."[38]
X-rays
[edit | edit source]"Using images taken by Chandra's Advanced CCD Imaging Spectrometer in 2002 and Chandra's High-Resolution Camera in 2017, [...] Uranus [has been observed] emitting faint X-rays."[39]
"The observed Uranus X‐ray fluxes of 10−15–10−16 erg/cm2/s are consistent with previous observational limits and modeling predictions. These fluxes exceed expectations from scattered solar emission alone, suggesting either a larger X‐ray albedo than Jupiter/Saturn or the possibility of additional X‐ray production processes at Uranus."[40]
Ultraviolets
[edit | edit source]
The images at the top of the article are in the ultraviolet showing the aurorae of Uranus.
"These are among the first clear images, taken from the distance of Earth, to show aurorae on the planet Uranus. Aurorae are produced when high-energy particles from the Sun cascade along magnetic field lines into a planet's upper atmosphere. This causes the planet's atmospheric gasses to fluoresce. The ultraviolet images were taken at the time of heightened solar activity in November 2011 that successively buffeted the Earth, Jupiter, and Uranus with a gusher of charged particles from the Sun. Because Uranus' magnetic field is inclined 59 degrees to its spin axis, the auroral spots appear far from the planet's north and south poles. This composite image combines 2011 Hubble observations of the aurorae in visible and ultraviolet light, 1986 Voyager 2 photos of the cyan disk of Uranus as seen in visible light, and 2011 Gemini Observatory observations of the faint ring system as seen in infrared light."[41]
"In 2011, the NASA/ESA Hubble Space Telescope became the first Earth-based telescope to snap an image of the aurorae on Uranus. In 2012 and 2014 a team led by an astronomer from Paris Observatory took a second look at the aurorae using the ultraviolet capabilities of the Space Telescope Imaging Spectrograph (STIS) installed on Hubble."[42]
"They tracked the interplanetary shocks caused by two powerful bursts of solar wind travelling from the Sun to Uranus, then used Hubble to capture their effect on Uranus’ aurorae — and found themselves observing the most intense aurorae ever seen on the planet. By watching the aurorae over time, they collected the first direct evidence that these powerful shimmering regions rotate with the planet. They also re-discovered Uranus’ long-lost magnetic poles, which were lost shortly after their discovery by Voyager 2 in 1986 due to uncertainties in measurements and the featureless planet surface."[42]
Opticals
[edit | edit source]
The colors and filters used to obtain the image on the right are blue, 467 nm, green 547 nm, orange 647 nm, and red 845 nm. The image is part of the Outer Planet Atmosphere Legacy (OPAL) program.
Violets
[edit | edit source]
"This false-color view of the rings of Uranus was made from images taken by Voyager 2 on Jan. 21, 1986, from a distance of 4.17 million kilometers (2.59 million miles). All nine known rings are visible here; the somewhat fainter, pastel lines seen between them are contributed by the computer enhancement. Six 15-second narrow-angle images were used to extract color information from the extremely dark and faint rings. Two images each in the green, clear and violet filters were added together and averaged to find the proper color differences between the rings. The final image was made from these three color averages and represents an enhanced, false-color view. The image shows that the brightest, or epsilon, ring at top is neutral in color, with the fainter eight other rings showing color differences between them. Moving down, toward Uranus, we see the delta, gamma and eta rings in shades of blue and green; the beta and alpha rings in somewhat lighter tones; and then a final set of three, known simply as the 4, 5 and 6 rings, in faint off-white tones. Scientists will use this color information to try to understand the nature and origin of the ring material. The resolution of this image is approximately 40 km (25 mi). The Voyager project is managed for NASA by the Jet Propulsion Laboratory."[43]
Blues
[edit | edit source]

The images show Uranus through a blue filter.
Cyans
[edit | edit source]
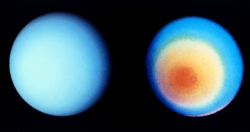


In larger amateur telescopes with an objective diameter of between 15 and 23 cm, the planet appears as a pale cyan disk with distinct limb darkening.
"Methane possesses prominent absorption bands in the visible and near-infrared (IR) making Uranus aquamarine or cyan in color."[16]
In 1986 Voyager 2 found that the visible southern hemisphere of Uranus can be subdivided into two regions: a bright polar cap and dark equatorial bands (see figure on the right).[18] Their boundary is located at about -45 degrees of latitude. A narrow band straddling the latitudinal range from -45 to -50 degrees is the brightest large feature on the visible surface of the planet.[18][26] It is called a southern "collar". The cap and collar are thought to be a dense region of methane clouds located within the pressure range of 1.3 to 2 bar (see above).[25] Besides the large-scale banded structure, Voyager 2 observed ten small bright clouds, most lying several degrees to the north from the collar.[18] In all other respects Uranus looked like a dynamically dead planet in 1986. Unfortunately Voyager 2 arrived during the height of the planet's southern summer and could not observe the northern hemisphere. At the beginning of the 21st century, when the northern polar region came into view, the Hubble Space Telescope (HST) and Keck telescope initially observed neither a collar nor a polar cap in the northern hemisphere.[26] So Uranus appeared to be asymmetric: bright near the south pole and uniformly dark in the region north of the southern collar.[26] In 2007, when Uranus passed its equinox, the southern collar almost disappeared, while a faint northern collar emerged near 45 degrees of latitude.[44]
On August 23, 2006, researchers at the Space Science Institute (Boulder, CO) and the University of Wisconsin observed a dark spot on Uranus's surface, giving astronomers more insight into the planet's atmospheric activity.[22] Why this sudden upsurge in activity should be occurring is not fully known, but it appears that Uranus's extreme axial tilt results in extreme seasonal variations in its weather.[45][23] Determining the nature of this seasonal variation is difficult because good data on Uranus's atmosphere have existed for less than 84 years, or one full Uranian year. A number of discoveries have been made. Photometry over the course of half a Uranian year (beginning in the 1950s) has shown regular variation in the brightness in two spectral bands, with maxima occurring at the solstices and minima occurring at the equinoxes.[46] A similar periodic variation, with maxima at the solstices, has been noted in microwave measurements of the deep troposphere begun in the 1960s.[47] Stratospheric temperature measurements beginning in the 1970s also showed maximum values near the 1986 solstice.[48] The majority of this variability is believed to occur owing to changes in the viewing geometry.[20]
There are some reasons to believe that physical seasonal changes are happening in Uranus. While the planet is known to have a bright south polar region, the north pole is fairly dim, which is incompatible with the model of the seasonal change outlined above.[23] During its previous northern solstice in 1944, Uranus displayed elevated levels of brightness, which suggests that the north pole was not always so dim.[46] This information implies that the visible pole brightens some time before the solstice and darkens after the equinox.[23] Detailed analysis of the visible and microwave data revealed that the periodical changes of brightness are not completely symmetrical around the solstices, which also indicates a change in the meridional albedo patterns.[23] Finally in the 1990s, as Uranus moved away from its solstice, Hubble and ground based telescopes revealed that the south polar cap darkened noticeably (except the southern collar, which remained bright),[25] while the northern hemisphere demonstrated increasing activity,[29] such as cloud formations and stronger winds, bolstering expectations that it should brighten soon.[21] This indeed happened in 2007 when the planet passed an equinox: a faint northern polar collar arose, while the southern collar became nearly invisible, although the zonal wind profile remained slightly asymmetric, with northern winds being somewhat slower than southern.[44]
Greens
[edit | edit source]
"The "aqua" image (on the right) is taken at 5,470 Angstroms, which is near the human eye's peak response to wavelength. Color has been added to the image to show what a person on a spacecraft near Uranus might see. Little structure is evident at this wavelength, though with image-processing techniques, a small cloud can be seen near the planet's northern limb (rightmost edge)."[49]
Oranges
[edit | edit source]

"Spring has finally come to the northern hemisphere of Uranus. The newest images, both the visible-wavelength ones described here and those taken a few days earlier with the Near Infrared and Multi-Object Spectrometer (NICMOS) by Erich Karkoschka (University of Arizona), show a planet with banded structure and detectable clouds."[49]
"The "red" image (on the right) is taken at 6,190 Angstroms, and is sensitive to absorption by methane molecules in the planet's atmosphere. The banded structure of Uranus is evident, and the small cloud near the northern limb is now visible."[49]
The picture of Uranus in true color was "compiled from images returned Jan. 17, 1986, by the narrow-angle camera of Voyager 2. The spacecraft was 9.1 million kilometers (5.7 million miles) from the planet, several days from closest approach. The picture [...] has been processed to show Uranus as human eyes would see it from the vantage point of the spacecraft. The picture is a composite of images taken through blue, green and orange filters. The darker shadings at the upper right of the disk correspond to the day-night boundary on the planet. Beyond this boundary lies the hidden northern hemisphere of Uranus, which currently remains in total darkness as the planet rotates. The blue-green color results from the absorption of red light by methane gas in Uranus' deep, cold and remarkably clear atmosphere."[50]
Yellows
[edit | edit source]
The image on the right depicts changes of the visible magnitude, or brightness, of Uranus in the yellow and blue bands (upper graph), the effective microwave temperature of the deep troposphere (middle graph) and stratospheric temperature in the pressure range 20–50 μbar (lower graph).
The data for the plots are
- yellow intensity and blue intensity, both as visible magnitude (Tables 1-3),[51]
- microwave brightness temperature (Tables 1-2),[52] and
- stratospheric temperature.[53]
Infrareds
[edit | edit source]


At right is an infrared image of Uranus "showing Ariel in transit across Uranus, taken on July 26, 2006. Due to the planet's extreme axial tilt--carried through in the tilt of its satellites's orbits--transits are only possible near the equinoxes. Taken in the near-infrared, atmospheric banding and the planet's oblateness are readily apparent."[54]
The second pair of images on the left are an "infrared composite ... of the two hemispheres of Uranus obtained with Keck adaptive optics. The component colors of blue, green, and red were obtained from images made at near infrared wavelengths of 1.26, 1.62, and 2.1 microns respectively. The images were obtained on July 11 and 12, 2004. The representative balance of these infrared images which were selected to display the vertical structure of atmospheric features gives a reddish tint to the rings, an artifact of the process. The North pole is at 4 o'clock."[55]
The lower right image shows an apparent linear arrangement of clouds in the southern hemisphere using the Hubble Space Telescope in 1998.
The second image at the left is in the "near infrared wavelengths by the Gemini North Telescope [revealing] what scientists are calling an “anvil cloud of methane” rising up from the depths into the sunshine. Reflections from methane ice crystals are supposed to be causing the bright patch."[56]
"Over the years, the Hubble Space Telescope has observed many bright spots on Uranus. They appear similar to the bright spots seen in Jupiter’s southern latitudes, as well as in its polar aurorae."[56]
On Saturn, a “great white spot” periodically appears in its southern latitudes."[56]
“Saturn occasionally ‘burps’, creating a great white spot 3 times the size of the Earth. It is inexplicable on standard models. However, it is the kind of thing to be expected following an exceptionally powerful lightning discharge deep into Saturn’s atmosphere. The discharge forms a vertical jet of matter from the depths that spouts into the upper atmosphere.”[57]
"Hubble Space Telescope has peered deep into Uranus' atmosphere to see clear and hazy layers created by a mixture of gases. Using infrared filters, Hubble captured detailed features of three layers of Uranus' atmosphere."[58]
"Hubble's images are different from the ones taken by the Voyager 2 spacecraft, which flew by Uranus 10 years ago. Those images - not taken in infrared light - showed a greenish-blue disk with very little detail."[58]
"The infrared image [third down on the right] allows astronomers to probe the structure of Uranus' atmosphere, which consists of mostly hydrogen with traces of methane. The red around the planet's edge represents a very thin haze at a high altitude. The haze is so thin that it can only be seen by looking at the edges of the disk, and is similar to looking at the edge of a soap bubble. The yellow near the bottom of Uranus is another hazy layer. The deepest layer, the blue near the top of Uranus, shows a clearer atmosphere."[58]
"Image processing has been used to brighten the rings around Uranus so that astronomers can study their structure. In reality, the rings are as dark as black lava or charcoal."[58]
"This false color picture was assembled from several exposures taken July 3, 1995 by the Wide Field Planetary Camera-2."[58]
Submillimeters
[edit | edit source]
For observations on "UT 1991 November 19 and 20[, the] primary pointing and calibration source was Uranus, for which we used S0.8 = 73.5 Jy (TB = 84 K) and S1.1 = 43.4 Jy (TB = 93 K)."[59]
"As a demonstration of ALMA's new short wavelength capabilities, the commissioning team released a new image of planet Uranus as it appears in submillimetre wavelength light. The image — obtained with ALMA's shortest wavelength, Band 10 receivers — reveals the icy glow from the planet's atmosphere, which can reach temperatures as low as -224 C (giving Uranus the coldest atmosphere in the Solar System). ALMA's now broader range of capabilities will enable astronomers and planetary scientists to study and monitor temperature changes at different altitudes above the clouds of Uranus and other giant planets in our Solar System."[60]
Astrognosy
[edit | edit source]
Uranus has a core and a mantle as shown in the model on the right.
Astrochemistry
[edit | edit source]In January 1986, the Voyager 2 spacecraft flew by Uranus at a minimal distance of 107,100 km[61] providing the first close-up images and spectra of its atmosphere. They generally confirmed that the atmosphere was made of mainly hydrogen and helium with around 2% methane.[62] The atmosphere appeared highly transparent and lacking thick stratospheric and tropospheric hazes. Only a limited number of discrete clouds were observed.[18]
Atmospheres
[edit | edit source]
Uranus's atmosphere has a primary composition of hydrogen and helium and contains more "ices" such as water, ammonia, and methane, along with traces of hydrocarbons.[16] The helium molar fraction, i.e. the number of helium atoms per molecule of gas, is 0.15 ± 0.03[63] in the upper troposphere, which corresponds to a mass fraction 0.26 ± 0.05.[16][64] The third most abundant constituent of the Uranian atmosphere is methane (CH4).[16] Methane molecules account for 2.3% of the atmosphere by molar fraction below the methane cloud deck at the pressure level of 1.3 bar (130 kPa); this represents about 20 to 30 times the carbon abundance found in the Sun.[16][65][66] The mixing ratio is much lower in the upper atmosphere owing to its extremely low temperature, which lowers the saturation level and causes excess methane to freeze out.[67] The abundances of less volatile compounds such as ammonia, water and hydrogen sulfide in the deep atmosphere are poorly known. Along with methane, trace amounts of various hydrocarbons are found in the stratosphere of Uranus, which are thought to be produced from methane by photolysis induced by the solar ultraviolet (UV) radiation.[68] They include ethane (C2H6), acetylene (C2H2), methylacetylene (CH3C2H), and diacetylene (C2HC2H).[67][69][70] Spectroscopy has also uncovered traces of water vapor, carbon monoxide and carbon dioxide in the upper atmosphere, which can only originate from an external source such as infalling dust and comets.[69][70][71]
“The weather on Uranus is incredibly active.”[72]
“This type of activity would have been expected in 2007, when Uranus’s once-every-42-year equinox occurred and the Sun shined directly on the equator. But we predicted that such activity would have died down by now. Why we see these incredible storms now is beyond anybody’s guess.”[73]
Eight "large storms [were detected] on Uranus when observing the planet with the Keck Observatory on August 5 and 6, 2014."[72]
Atmospheric astrognosy
[edit | edit source]
The troposphere is the lowest and densest part of the atmosphere and is characterized by a decrease in temperature with altitude.[16] The temperature falls from about 320 K at the base of the nominal troposphere at −300 km to 53 K at 50 km.[74][66] The temperatures in the coldest upper region of the troposphere (the tropopause) actually vary in the range between 49 and 57 K depending on planetary latitude.[16][27] The tropopause region is responsible for the vast majority of the planet’s thermal far infrared emissions, thus determining its effective temperature of 59.1 ± 0.3 K.[27][64]
The middle layer of the Uranian atmosphere is the stratosphere, where temperature generally increases with altitude from 53 K in the tropopause to between 800 and 850 K at the base of the thermosphere.[75] The heating of the stratosphere is caused by absorption of solar UV and IR radiation by methane and other hydrocarbons,[76] which form in this part of the atmosphere as a result of methane photolysis.[68] Heat is also conducted from the hot thermosphere.[76] The hydrocarbons occupy a relatively narrow layer at altitudes of between 100 and 300 km corresponding to a pressure range of 10 to 0.1 mbar (1000 to 10 kPa) and temperatures of between 75 and 170 K.[67][69] The most abundant hydrocarbons are methane, acetylene and ethane with mixing ratios of around 10−7 relative to hydrogen. The mixing ratio of carbon monoxide is similar at these altitudes.[67][69][71] Heavier hydrocarbons and carbon dioxide have mixing ratios three orders of magnitude lower.[69] The abundance ratio of water is around 7×10−9.[70] Ethane and acetylene tend to condense in the colder lower part of stratosphere and tropopause (below 10 mBar level) forming haze layers,[68] which may be partly responsible for the bland appearance of Uranus. The concentration of hydrocarbons in the Uranian stratosphere above the haze is significantly lower than in the stratospheres of the other giant planets.[67][14]
The outermost layer of the Uranian atmosphere is the thermosphere and corona, which has a uniform temperature around 800 to 850 K.[16][14] The heat sources necessary to sustain such a high value are not understood, since neither solar far UV and extreme UV radiation nor auroral activity can provide the necessary energy. The weak cooling efficiency due to the lack of hydrocarbons in the stratosphere above 0.1 mBar pressure level may contribute too.[75][14] In addition to molecular hydrogen, the thermosphere-corona contains many free hydrogen atoms. Their small mass together with the high temperatures explain why the corona extends as far as 50 000 km or two Uranian radii from the planet.[75][14] This extended corona is a unique feature of Uranus.[14] Its effects include a drag on small particles orbiting Uranus, causing a general depletion of dust in the Uranian rings.[75] The Uranian thermosphere, together with the upper part of the stratosphere, corresponds to the ionosphere of Uranus.[66] Observations show that the ionosphere occupies altitudes from 2 000 to 10 000 km.[66] The Uranian ionosphere is denser than that of either Saturn or Neptune, which may arise from the low concentration of hydrocarbons in the stratosphere.[14][77] The ionosphere is mainly sustained by solar UV radiation and its density depends on the solar activity.[78] Auroral activity is insignificant as compared to Jupiter and Saturn.[14] [15]
It is the coldest planetary atmosphere in the Solar System, with a minimum temperature of 49 K (−224 °C). The lowest temperature recorded in Uranus's tropopause is 49 K (−224 °C), making Uranus the coldest planet in the Solar System.[16][64]
Coronal clouds
[edit | edit source]The upper part of the thermosphere, where the mean free path of the molecules exceeds the scale height, [The scale height sh is defined as sh = RT/(Mgj), where R = 8.31 J/mol/K is the gas constant, M ≈ 0.0023 kg/mol is the average molar mass in the Uranian atmosphere,[16] T is temperature and gj ≈ 8.9 m/s2 is the gravitational acceleration at the surface of Uranus. As the temperature varies from 53 K in the tropopause up to 800 K in the thermosphere, the scale height changes from 20 to 400 km.] is called the exosphere.[79] The lower boundary of the Uranian exosphere, the exobase, is located at a height of about 6,500 km, or 1/4 of the planetary radius, above the surface.[79] The exosphere is unusually extended, reaching as far as several Uranian radii from the planet.[79][14] It is made mainly of hydrogen atoms and is often called the hydrogen corona of Uranus.[75] The high temperature and relatively high pressure at the base of the thermosphere explain in part why Uranus's exosphere is so vast. The corona contains a significant population of supra-thermal (energy of up to 2 eV) hydrogen atoms. Their origin is unclear, but they may be produced by the same mechanism that heats the thermosphere.[79][14] The number density of atomic hydrogen in the corona falls slowly with the distance from the planet, remaining as high a few hundred atoms per cm3 at a few radii from Uranus.[79] The effects of this bloated exosphere include a drag on small particles orbiting Uranus, causing a general depletion of dust in the Uranian rings. The infalling dust in turn contaminates the upper atmosphere of the planet.[75]
Uranus systems
[edit | edit source]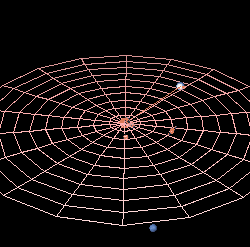







"A near-infrared view [in the K band, 2.2 µm, is on the lower right] of the giant planet Uranus with rings and some of its moons, obtained on November 19, 2002, with the ISAAC multi-mode instrument on the 8.2-m VLT ANTU telescope at the ESO Paranal Observatory (Chile). The moons are identified; the unidentified, round object to the left is a background star. The image scale in indicated by the bar."[80]
The second image down on the right is a montage of Uranus' five largest satellites. From left to right in order of increasing distance from Uranus are Miranda, Ariel, Umbriel, Titania and Oberon. Images are presented to show correct relative sizes and brightness. Coverage is incomplete for Miranda and Ariel; gray circles depict missing areas (original caption from NASA though revised as image has been rotated).
The second image down on the left shows the "rings of Uranus are shown here captured almost exactly edge-on to Earth. This false-colour image was obtained by the NAOS-CONICA infrared camera on ESO's Very Large Telescope at Paranal, Chile. It was taken at 9:00 UT on 16 August 2007, just two hours after Earth had crossed to the lit side of the ring plane. We are peering over the sunlit face of the rings at an opening of only 0.003 degree, an angle so small that the thin rings nearly disappear. At right, the region around the planet has been enhanced to show a thin line, which is sunlight glinting off the ring edges and also reflected by dust clouds embedded within the system. The pictures at left shows the planet and identifies four of its largest moons. One can clearly discern banding in the atmosphere and a bright cloud feature near the planet's south polar collar, on the left side of the image. This is a composite of images taken at infrared wavelengths. The planet is shown in false colour, based on images taken at wavelengths of 1.2 and 1.6 microns. The rings are extracted from an image taken at 2.2 microns, where the planet is darker and therefore the rings are easier to detect."[81]
The third image down on the right shows a scheme of the rings and close moons of Uranus. Rings are full lines, to scale where possible. Orbits of moons are dashed.
Fourth down on the right is a diagram of the planet Uranus that includes the moons and rings.
On the bottom right is the Uranus system with its moons at 2006-16-08 03:25 UT, just half an hour from a transit of Miranda. It is imaged through the infrared I filter.
Its average distance from the Sun is roughly 3 billion km (about 20 AU).
Titania
[edit | edit source]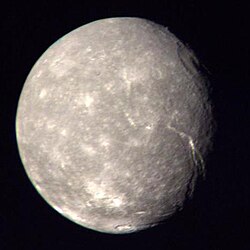
Voyager's narrow-angle camera acquired the image at right of Titania, one of the large moons of Uranus, through the violet and clear filters. The spacecraft was about 500,000 kilometers (300,000 miles) away; the picture shows details about 9 km (6 mi) in size. Titania has a diameter of about 1,600 km (1,000 mi). In addition to many scars due to impacts, Titania displays evidence of other geologic activity at some point in its history. The large, trenchlike feature near the terminator (day-night boundary) at middle right suggests at least one episode of tectonic activity. Another, basinlike structure near the upper right is evidence of an ancient period of heavy impact activity. The neutral gray color of Titania is characteristic of the Uranian satellites as a whole.
Titania consists of approximately equal amounts of ice and rock.
The surface has a mottled appearance with bright patches among relatively dark terrain. The terminator is located near the right edge. A large crater can be seen at the terminator in the upper half of the image. Another bright crater can be seen at the bottom. A large canyon runs from the darkness at the lower-right side to visible center of the body.
The surface of Titania, which is relatively dark and slightly red in color, appears to have been shaped by both impacts and endogenic processes. It is covered with numerous impact craters reaching up to 326 km in diameter ... Titania's surface is cut by a system of enormous canyons and scarps, the result of the expansion of its interior during the later stages of its evolution.
Infrared spectroscopy conducted from 2001 to 2005 revealed the presence of water ice as well as frozen carbon dioxide on the surface of Titania, which in turn suggested that the moon may possess a tenuous carbon dioxide atmosphere with a surface pressure of about one 10 trillionth of a bar.
Miranda
[edit | edit source]
"This color composite [at lower right] of the Uranian satellite Miranda was taken by Voyager 2 on Jan. 24, 1986, from a distance of 147,000 kilometers (91,000 miles). This picture was constructed from images taken through the narrow-angle camera's green, violet and ultraviolet filters. It is the best color view of Miranda returned by Voyager."[82]
"Miranda, just 480 km (300 mi) across, is the smallest of Uranus' five major satellites. Miranda's regional geologic provinces show very well in this view of the southern hemisphere, imaged at a resolution of 2.7 km (1.7 mi). The dark- and bright-banded region with its curvilinear traces covers about half of the image. Higher-resolution pictures taken later show many fault valleys and ridges parallel to these bands. Near the terminator (at right), another system of ridges and valleys abuts the banded terrain; many impact craters pockmark the surface in this region. The largest of these are about 30 km (20 mi) in diameter; many more lie in the range of 5 to 10 km (3 to 6 mi) in diameter."[82]
Oberon
[edit | edit source]
This Voyager 2 picture of Oberon is the best the spacecraft acquired of Uranus' outermost moon. The picture was taken shortly after 3:30 a.m. PST on Jan. 24, 1986, from a distance of 660,000 kilometers (410,000 miles). The color was reconstructed from images taken through the narrow-angle camera's violet, clear and green filters. The picture shows features as small as 12 km (7 mi) on the moon's surface. Clearly visible are several large impact craters in Oberon's icy surface surrounded by bright rays similar to those seen on Jupiter's moon Callisto. Quite prominent near the center of Oberon's disk is a large crater with a bright central peak and a floor partially covered with very dark material. This may be icy, carbon-rich material erupted onto the crater floor sometime after the crater formed. Another striking topographic feature is a large mountain, about 6 km (4 mi) high, peeking out on the lower left limb.
Ancient history
[edit | edit source]


The ancient history period dates from around 8,000 to 3,000 b2k.
In the Chinese, Japanese, Korean, and Vietnamese languages, the planet's name is literally translated as the sky king star[83][84]
Uranus is named after the ancient Greek deity of the sky Uranus, the father of Cronus (Saturn) and grandfather of Zeus (Jupiter). Though it is visible to the naked eye like the five classical planets, it was never recognized as a planet by ancient observers because of its dimness and slow orbit.[85]
The second image at right is a painting by artist Giorgio Vasari (1511–1574). The main focus is on Cronus (Saturn) castrating Uranus (the Greek sky god). As both Uranus and Cronus are represented by men, this suggests that they were similar in nature. "[T]he ancients’ religions and mythology speak for their knowledge of Uranus; the dynasty of gods had Uranus followed by Saturn, and the latter by Jupiter. ... It is quite possible that the planet Uranus is the very planet known by this name to the ancients. The age of Uranus preceded the age of Saturn; it came to an end with the “removal” of Uranus by Saturn. Saturn is said to have emasculated his father Uranus."[86]
Ouranos
[edit | edit source]“Uranus was the Sky in Greek mythology, which was thought to be dominated by the combined powers of the Sun and Mars.[87]
Uranus ... , Ouranos meaning "sky" or "heaven") was the primal Greek god personifying the sky. His equivalent in Roman mythology was Caelus. In Ancient Greek literature, Uranus or Father Sky was the son and husband of Gaia, Mother Earth. According to Hesiod's Theogony, Uranus was conceived by Gaia alone, but other sources cite Aether as his father.[88]
According to the poet Alcman, Aether was the father of Ouranos, the god of the sky. While Aether was the personification of the upper air, Ouranos was literally the sky itself, composed of a solid dome of brass.[89]
Caelus
[edit | edit source]
Caelus or Coelus was a primal god of the sky in Roman myth and theology, iconography, and literature (compare caelum, the Latin word for "sky" or "the heavens", hence English "celestial").
The name of Caelus indicates that he was the Roman counterpart of the Greek god Uranus, who was of major importance in the theogonies of the Greeks. Varro couples him with Terra (Earth) as pater and mater (father and mother), and says that they are "great deities" (dei magni) in the theology of the mysteries at Samothrace.[90]
According to Cicero and Hyginus, Caelus was the son of Aether and Dies ("Day" or "Daylight").[91] Caelus and Dies were in this tradition the parents of Mercury.[92] Caelus was the father with Hecate of the distinctively Roman god Janus, as well as of Saturn and Ops.[93] Caelus was also the father of one of the three forms of Jupiter, the other two fathers being Aether and Saturn.[94]
Aion
[edit | edit source]
Aion (Greek Αἰών) is a Hellenistic deity associated with time, the orb or circle encompassing the universe, and the zodiac. The "time" represented by Aion is unbounded, in contrast to Chronos as empirical time divided into past, present, and future.[95] He is thus a god of eternity, associated with mystery religions concerned with the afterlife, such as the mysteries of Cybele, Dionysus, Orpheus, and Mithras. In Latin the concept of the deity may appear as Aevum or Saeculum.[96] He is typically in the company of an earth or mother goddess such as Tellus or Cybele, as on the Parabiago plat].[97] The picture at page right top is of Aion-Uranus.
Physics
[edit | edit source]Uranus has an equatorial radius of 25,559 ± 4 km and a polar radius of 24,973 ± 20 km.[98]
Hypotheses
[edit | edit source]- Uranus was closer to the Sun about 40,000 b2k.
- What was to become Uranus may have been in a binary system with the Sun.
- The Sun with the Earth in orbit may have caught up to or been caught up to by a red or brown dwarf star in orbit around the galaxy. Earth's orbit around the Sun at some 51° to the galactic plane may have produced the effect that the approaching dwarf appeared as a pole star ever increasing in size and brightness on approach.
Current theories of formation
[edit | edit source]

An orbital pole is either end of an imaginary line running through the center of an orbit perpendicular to the orbital plane, projected onto the celestial sphere. It is similar in concept to a celestial pole but based on the planet's orbit instead of the planet's rotation.
The north orbital pole of a celestial body is defined by the right-hand rule: If you curve the fingers of your right hand along the direction of orbital motion, with your thumb extended parallel to the orbital axis, the direction your thumb points is defined to be north.
At right is a snapshot of the planetary orbital poles.[99] The field of view is about 30°. The yellow dot in the centre is the Sun's North pole. Off to the side, the orange dot is Jupiter's orbital pole. Clustered around it are the other planets: Mercury in pale blue (closer to the Sun than to Jupiter), Venus in green, the Earth in blue, Mars in red, Saturn in violet, Uranus in grey partly underneath Earth and Neptune in lavender. Dwarf planet Pluto is the dotless cross off in Cepheus.
The ice giants formed close to the Sun, then they migrated out towards their current orbits after the withdrawal of the gaseous protoplanetary disk.[100] This hypothesis of migration after formation is now favored because of its ability to better explain the occupation of populations of small objects observed in the trans-Neptunian region.[101][102] The most widely accepted explanation of the details of this hypothesis is known as the Nice model, which explores the effect of a migration of Uranus and the other giant planets on the structure of the Kuiper belt.[103][104][105]
A "planetary system with initial quasi-circular, coplanar orbits would have evolved to the current orbital configuration, provided that Jupiter and Saturn crossed their 1:2 orbital resonance."[106]
This "resonance crossing could have occurred as the giant planets migrated owing to their interaction with a disk of planetesimals6,7."[106]
"After the giant planets were formed and the circumsolar gaseous nebula was dissipated, the Solar System was composed of the Sun, the planets and a debris disk of small planetesimals. The planets then started to erode the disk, by either accreting or scattering away the planetesimals. The planets migrated because of the exchange of angular momentum with the disk particles during this process6,7. Numerical simulations8 show that Jupiter was forced to move inward, while Saturn, Uranus and Neptune drifted outward. The orbital distribution of trans-neptunian objects is probably the result of such planetary migration7, and suggests that Neptune probably started migrating well inside 20 AU while the disk was extended up to 30–35 AU (refs 9–11)."[106]
"The initial semimajor axes of the ice giants (Uranus and Neptune) were varied in the ranges 11–13 AU and 13.5–17 AU, while keeping their initial orbital separation larger than 2 AU."[106]
"The secular perturbations that Jupiter and Saturn exert on Uranus and Neptune force the eccentricities of the ice giants to increase by an amount that depends on the masses and semimajor axes of all planets15. As a result of the ‘compactness’ of the system, the planetary orbits become chaotic and intersect. When this occurs, a short phase of encounters follows the resonance crossing event. These encounters increase the inclinations of the planetary orbits by 1°–7°. In addition, both ice giants are scattered outward and penetrate the disk. Thus, the flux of small bodies towards Saturn and Jupiter, and hence their rate of migration, increases abruptly. During this fast migration phase, the eccentricities and inclinations of the planets slowly decrease by dynamical friction and the planetary system is stabilized. The planets stop migrating when the disk is almost completely depleted."[106]
"The final orbits of the planets depend on the evolution of the system immediately after the resonance crossing event. Although there were many free parameters in our initial conditions, we found that the final configuration is most sensitive to the initial orbital separation between the ice giants (∆aI1,I2) and, more importantly, to the one between Saturn and the inner ice giant (∆aS,I1). [...] ∆aI1,I2 ranged from ~2 to ~6 AU, while ∆aS,I1 ranged from ~2.5 to ~5 AU."[106]
"[Saturn suffered an encounter with one or both ice giants, Uranus and Neptune, called] class B runs give aU = 19.3 ± 1.3 AU and aN = 29.9 ± 2.4 AU, the observed values being aU = 19.2 AU and aN = 30.1 AU. (Here aU and aN are the semimajor axes of Uranus and Neptune, respectively.)"[106]
Future Exporation
[edit | edit source]China has plans to explore in 2049.[107]
See also
[edit | edit source]References
[edit | edit source]- ↑ 1.0 1.1 1.2 solar system. San Francisco, California: Wikimedia Foundation, Inc. 25 May 2014. https://en.wiktionary.org/wiki/solar_system. Retrieved 2014-06-06.
- ↑ Red Prince (25 April 2004). Uranus. San Francisco, California: Wikimedia Foundation, Inc. https://en.wiktionary.org/wiki/Uranus. Retrieved 2016-03-29.
- ↑ Jacob Kegerreis (December 22, 2018). Uranus is weird and researchers think a giant collision caused it. Yahoo News. https://news.yahoo.com/uranus-weird-researchers-think-giant-153536157.html?.tsrc=daily_mail&uh_test=1_07. Retrieved 24 December 2018.
- ↑ 4.0 4.1 Kellen Beck (December 22, 2018). Uranus is weird and researchers think a giant collision caused it. Yahoo News. https://news.yahoo.com/uranus-weird-researchers-think-giant-153536157.html?.tsrc=daily_mail&uh_test=1_07. Retrieved 24 December 2018.
- ↑ 5.0 5.1 5.2 5.3 Sabine Stanley; Jeremy Bloxham (March 2004). "Convective-region geometry as the cause of Uranus' and Neptune's unusual magnetic fields". Nature 428 (6979): 151-3. doi:10.1038/nature02376. http://adsabs.harvard.edu/abs/2004Natur.428..151S. Retrieved 2014-03-29.
- ↑ 6.0 6.1 6.2 6.3 6.4 6.5 6.6 6.7 6.8 Norman F. Ness; Mario H. Acuña; Kenneth W. Behannon; Leonard F. Burlaga; John E. P. Connerney; Ronald P. Lepping; Fritz M. Neubauer (July 4, 1986). "Magnetic Fields at Uranus". Science 233 (4759): 85-9. doi:10.1126/science.233.4759.85. http://adsabs.harvard.edu/abs/1986Sci...233...85N. Retrieved 2014-03-29.
- ↑ 7.0 7.1 7.2 7.3 7.4 7.5 7.6 C.T. Russell (1993). "Planetary Magnetospheres". Rep. Prog. Phys. 56 (6): 687–732. doi:10.1088/0034-4885/56/6/001.
- ↑ S. Atreya; Egeler P.; Baines, K. (2006). "Water-ammonia ionic ocean on Uranus and Neptune?" (PDF). Geophysical Research Abstracts 8: 05179. http://www.cosis.net/abstracts/EGU06/05179/EGU06-J-05179-1.pdf.
- ↑ Sabine Stanley; Bloxham, Jeremy (2004). "Convective-region geometry as the cause of Uranus' and Neptune's unusual magnetic fields". Letters to Nature 428 (6979): 151–153. doi:10.1038/nature02376. PMID 15014493. Archived from the original on August 7, 2007. http://web.archive.org/web/20070807213745/http://mahi.ucsd.edu/johnson/ES130/stanley2004-nature.pdf. Retrieved August 5, 2007.
- ↑ 10.0 10.1 10.2 10.3 10.4 10.5 S. M. Krimigis; T. P. Armstrong; W. I. Axford; A. F. Cheng; G. Gloeckler (4 July 1986). "The magnetosphere of Uranus - Hot plasma and radiation environment". Science 233 (07): 97-102. doi:10.1126/science.233.4759.97.
- ↑ Voyager: Uranus: Magnetosphere. 2003. http://voyager.jpl.nasa.gov/science/uranus_magnetosphere.html. Retrieved June 13, 2007.
- ↑ H.S. Bridge; J.W. Belcher; B. Coppi; A. J. Lazarus; R. L. McNutt; S. Olbert; J. D. Richardson; M. R. Sands et al. (1986). "Plasma Observations Near Uranus: Initial Results from Voyager 2". Science 233 (4759): 89–93. doi:10.1126/science.233.4759.89. PMID 17812895.
- ↑ Voyager Uranus Science Summary. 1988. http://www.solarviews.com/eng/vgrur.htm. Retrieved June 9, 2007.
- ↑ 14.00 14.01 14.02 14.03 14.04 14.05 14.06 14.07 14.08 14.09 Floyd Herbert; Bill R. Sandel (August 1999). "Ultraviolet observations of Uranus and Neptune". Planetary and Space Science 47 (8-9): 1119-39. doi:10.1016/S0032-0633(98)00142-1. http://adsabs.harvard.edu/abs/1999P%26SS...47.1119H. Retrieved 2016-06-27.
- ↑ 15.0 15.1 Hoanh An Lam; Steven Miller; Robert D. Joseph; Thomas R. Geballe; Laurence M. Trafton; Jonathan Tennyson; Gilda E. Ballester (1 January 1997). "Variation in the H3+ Emission of Uranus". The Astrophysical Journal Letters 474 (1): L73-6. doi:10.1086/310424. http://iopscience.iop.org/article/10.1086/310424/fulltext/. Retrieved 2016-06-27.
- ↑ 16.00 16.01 16.02 16.03 16.04 16.05 16.06 16.07 16.08 16.09 16.10 Jonathan I. Lunine (1993). "The Atmospheres of Uranus and Neptune". Annual Review of Astronomy and Astrophysics 31: 217–63. doi:10.1146/annurev.aa.31.090193.001245.
- ↑ Nowak, Gary T. (2006). Uranus: the Threshold Planet of 2006. http://www.vtastro.org/Articles/uranus2006.html. Retrieved June 14, 2007.
- ↑ 18.0 18.1 18.2 18.3 18.4 18.5 Smith, B. A.; Soderblom, L. A.; Beebe, A.; Bliss, D.; Boyce, J. M.; Brahic, A.; Briggs, G. A.; Brown, R. H. et al (4 July 1986). "Voyager 2 in the Uranian System: Imaging Science Results". Science 233 (4759): 43–64. doi:10.1126/science.233.4759.43. PMID 17812889.
- ↑ 19.00 19.01 19.02 19.03 19.04 19.05 19.06 19.07 19.08 19.09 19.10 Sromovsky, L. A.; Fry, P. M. (December 2005). "Dynamics of cloud features on Uranus". Icarus 179 (2): 459–484. Bibcode 2005Icar..179..459S. doi:10.1016/j.icarus.2005.07.022.
- ↑ 20.0 20.1 Karkoschka, Erich (May 2001). "Uranus' Apparent Seasonal Variability in 25 HST Filters". Icarus 151 (1): 84–92. Bibcode 2001Icar..151...84K. doi:10.1006/icar.2001.6599.
- ↑ 21.0 21.1 21.2 21.3 21.4 Hammel, H. B.; de Pater, I.; Gibbard, S. G.; Lockwood, G. W.; Rages, K. (May 2005). "New cloud activity on Uranus in 2004: First detection of a southern feature at 2.2 µm". Icarus 175 (1): 284–8. doi:10.1016/j.icarus.2004.11.016.
- ↑ 22.0 22.1 22.2 Sromovsky L.; Fry P.; Hammel H.; Rages K. Hubble Discovers a Dark Cloud in the Atmosphere of Uranus. physorg.com. http://www.physorg.com/pdf78676690.pdf. Retrieved August 22, 2007.
- ↑ 23.0 23.1 23.2 23.3 23.4 Hammel H.B.; Lockwood G.W. (2007). "Long-term atmospheric variability on Uranus and Neptune". Icarus 186: 291–301. doi:10.1016/j.icarus.2006.08.027.
- ↑ Devitt, Terry (2004). Keck zooms in on the weird weather of Uranus. University of Wisconsin-Madison. http://www.news.wisc.edu/10402.html. Retrieved December 24, 2006.
- ↑ 25.0 25.1 25.2 25.3 Rages, K. A.; Hammel, H. B.; Friedson, A. J. (11 September 2004). "Evidence for temporal change at Uranus' south pole". Icarus 172 (2): 548–54. doi:10.1016/j.icarus.2004.07.009.
- ↑ 26.0 26.1 26.2 26.3 26.4 Hammel, H. B.; de Pater, I.; Gibbard, S. G.; Lockwood, G. W.; Rages, K. (June 2005). "Uranus in 2003: Zonal winds, banded structure, and discrete features". Icarus 175 (2): 534–45. doi:10.1016/j.icarus.2004.11.012.
- ↑ 27.0 27.1 27.2 Hanel, R.; Conrath, B.; Flasar, F. M.; Kunde, V.; Maguire, W.; Pearl, J.; Pirraglia, J.; Samuelson, R. et al (4 July 1986). "Infrared Observations of the Uranian System". Science 233 (4759): 70–4. doi:10.1126/science.233.4759.70. PMID 17812891.
- ↑ Hammel, H. B.; Rages, K.; Lockwood, G. W.; Karkoschka, E.; de Pater, I. (October 2001). "New Measurements of the Winds of Uranus". Icarus 153 (2): 229–35. doi:10.1006/icar.2001.6689.
- ↑ 29.0 29.1 Emily Lakdawalla (2004). No Longer Boring: 'Fireworks' and Other Surprises at Uranus Spotted Through Adaptive Optics. http://web.archive.org/web/20060525015410/http://www.planetary.org/news/2004/1111_No_Longer_Boring_Fireworks_and_Other.html. Retrieved June 13, 2007.
- ↑ Podolak, M.; Weizman, A.; Marley, M. (December 1995). "Comparative models of Uranus and Neptune". Planetary and Space Science 43 (12): 1517–1522. doi:10.1016/0032-0633(95)00061-5.
- ↑ "Is It Raining Diamonds on Uranus". SpaceDaily.com. 1 October 1999. Retrieved 17 May 2013.
- ↑ Kaplan, Sarah (25 August 2017). "It rains solid diamonds on Uranus and Neptune". The Washington Post. Retrieved 27 August 2017.
- ↑ Kraus, D. et al. (September 2017). "Formation of diamonds in laser-compressed hydrocarbons at planetary interior conditions". Nature Astronomy 1 (9): 606–611. doi:10.1038/s41550-017-0219-9. http://www.escholarship.org/uc/item/1cf3b8v4.
- ↑ Bland, Eric (18 January 2010). "Outer planets may have oceans of diamond". ABC Science. Retrieved 9 October 2017.
- ↑ Baldwin, Emily (21 January 2010). "Oceans of diamond possible on Uranus and Neptune". Astronomy Now. Archived from the original on 3 December 2013. https://web.archive.org/web/20131203065217/http://www.astronomynow.com/news/n1001/21diamond/. Retrieved 6 February 2014.
- ↑ Sean Kane (April 29, 2016). "Lightning storms make it rain diamonds on Saturn and Jupiter". Business Insider. Retrieved May 22, 2019.
- ↑ Sarah Kaplan (March 25, 2017). "It rains solid diamonds on Uranus and Neptune". The Washington Post. Retrieved May 22, 2019.
- ↑ Chushiro Hayashi (1981). "Structure of the Solar Nebula, Growth and Decay of Magnetic Fields and Effects of Magnetic and Turbulent Viscosities on the Nebula". Progress Theoretical Physics Supplement (70): 35-53. doi:10.1143/PTPS.70.35. http://ptp.ipap.jp/link?PTPS/70/35/. Retrieved 2012-08-23.
- ↑ Elizabeth Gamillo (April 12, 2021). "Scientists Detect X-Rays Radiating From Uranus". Smithsonian Magazine. Retrieved 12 April 2021.
- ↑ W. R. Dunn, J.‐U. Ness, L. Lamy, G. R. Tremblay, G. Branduardi‐Raymont, B. Snios, R. P. Kraft, Z. Yao and A. D. Wibisono (31 March 2021). "A Low Signal Detection of X‐Rays From Uranus". Journal of Geophysical Research Space Physics 126 (4): e2020JA028739. doi:10.1029/2020JA028739. https://agupubs.onlinelibrary.wiley.com/doi/10.1029/2020JA028739. Retrieved 12 April 2021.
- ↑ Ray Villard; Laurent Lamy (April 19, 2012). Hubble Spots Aurorae on the Planet Uranus. STScI. http://hubblesite.org/newscenter/archive/releases/solar%20system/2012/21/image/a/. Retrieved 2013-04-27.
- ↑ 42.0 42.1 L. Lamy (3 April 2017). "Alien aurorae on Uranus". ESA/Hubble Images. Retrieved 12 April 2021.
- ↑ Sue Lavoie (January 29, 1996). PIA00033: Uranus Rings in False Color. Pasadena, California USA: NASA/JPL. http://photojournal.jpl.nasa.gov/catalog/PIA00033. Retrieved 2013-03-31.
- ↑ 44.0 44.1 Sromovsky, L. A.; Fry, P. M.; Hammel, H. B.; Ahue, W. M.; de Pater, I.; Rages, K. A.; Showalter, M. R.; van Dam, M. A. (September 2009). "Uranus at equinox: Cloud morphology and dynamics". Icarus 203 (1): 265–286. Bibcode 2009Icar..203..265S. doi:10.1016/j.icarus.2009.04.015.
- ↑ Hubble Discovers Dark Cloud In The Atmosphere Of Uranus. Science Daily. http://www.sciencedaily.com/releases/2006/10/061001211630.htm. Retrieved April 16, 2007.
- ↑ 46.0 46.1 Lockwood, G. W.; Jerzykiewicz, Mikołaj A. (February 2006). "Photometric variability of Uranus and Neptune, 1950–2004". Icarus 180 (2): 442–452. Bibcode 2006Icar..180..442L. doi:10.1016/j.icarus.2005.09.009.
- ↑ Klein, M. J.; Hofstadter, M. D. (September 2006). "Long-term variations in the microwave brightness temperature of the Uranus atmosphere". Icarus 184 (1): 170–180. Bibcode 2006Icar..184..170K. doi:10.1016/j.icarus.2006.04.012.
- ↑ Young, Leslie A.; Bosh, Amanda S.; Buie, Marc; et al. (2001). "Uranus after Solstice: Results from the 1998 November 6 Occultation". Icarus 153 (2): 236–247. doi:10.1006/icar.2001.6698. http://www.boulder.swri.edu/~layoung/eprint/ur149/Young2001Uranus.pdf.
- ↑ 49.0 49.1 49.2 Heidi Hammel (July 31, 1997). Clouds on Uranus. Boston, Massachusetts: NASA. http://solarsystem.nasa.gov/multimedia/display.cfm?Category=Planets&IM_ID=2104. Retrieved 2012-07-21.
- ↑ Sue Lavoie (August 1, 1996). PIA00032: Uranus in True and False Color. Pasadena, California USA: NASA/JPL. http://photojournal.jpl.nasa.gov/catalog/PIA00032. Retrieved 2014-03-04.
- ↑ G.W. Lockwood; Mikołaj Jerzykiewicz (2006). "Photometric variability of Uranus and Neptune, 1950–2004". Icarus 180: 442–452. doi:10.1016/j.icarus.2005.09.009.
- ↑ M.J. Klein; M.D. Hofstadter (2006). "Long-term variations in the microwave brightness temperature of the Uranus atmosphere". Icarus 184: 170–180. doi:10.1016/j.icarus.2006.04.012.
- ↑ Leslie A. Young; Amanda S. Bosh; Marc Buie; et al. (2001). "Uranus after Solstice: Results from the 1998 November 6 Occultation". Icarus 153: 236–247. doi:10.1006/icar.2001.6698.
- ↑ Erimus (February 28, 2011). File:Arieluranus.jpg. San Francisco, California: Wikimedia Foundation, Inc. http://commons.wikimedia.org/wiki/File:Arieluranus.jpg. Retrieved 2012-07-21.
- ↑ Samantha Harvey (August 19, 2004). Uranus from Earth. NASA and Keck Observatory. http://solarsystem.nasa.gov/multimedia/display.cfm?Category=Planets&IM_ID=3163. Retrieved 2012-07-21.
- ↑ 56.0 56.1 56.2 Stephen Smith (November 10, 2011). Sturm und Drang. The Thunderbolts Project. http://www.thunderbolts.info/wp/2011/11/10/sturm-und-drang/. Retrieved 2014-03-29.
- ↑ Wal Thornhill (November 10, 2011). Sturm und Drang. The Thunderbolts Project. http://www.thunderbolts.info/wp/2011/11/10/sturm-und-drang/. Retrieved 2014-03-29.
- ↑ 58.0 58.1 58.2 58.3 58.4 Sue Lavoie (2 August 1998). PIA01280: Hubble Captures Detailed Image of Uranus' Atmosphere. Pasadena, California USA: NASA/JPL. https://photojournal.jpl.nasa.gov/catalog/PIA01280. Retrieved 2017-05-03.
- ↑ David Jewitt; Jane Luu (November 1992). "Submillimeter Continuum Emission from Comets". Icarus 108 (1): 187-96. http://www.sciencedirect.com/science/article/pii/0019103592900286. Retrieved 2013-10-22.
- ↑ Ann14065b (10 September 2014). ALMA short wavelength image of Uranus. Chile: ALMA (ESO/NAOJ/NRAO). http://www.eso.org/public/images/ann14065b/. Retrieved 2015-04-09.
- ↑ Stone, E. C. (December 30, 1987). "The Voyager 2 Encounter with Uranus". Journal of Geophysical Research 92 (A13): 14,873–14,876. Bibcode 1987JGR....9214873S. doi:10.1029/JA092iA13p14873
- ↑ Fegley, Bruce Jr.; Gautier, Daniel; Owen, Tobias; Prinn, Ronald G. (1991). "Spectroscopy and chemistry of the atmosphere of Uranus". In Bergstrahl, Jay T.; Miner, Ellis D.; Matthews, Mildred Shapley (PDF). Uranus. University of Arizona Press. ISBN 978-0-8165-1208-9. OCLC 22625114.
- ↑ B. Conrath; R. Hanel; D. Gautier; A. Marten; G. Lindal (30 December 1987). "The helium abundance of Uranus from Voyager measurements". Journal of Geophysical Research 92 (12): 15003-10. doi:10.1029/JA092iA13p15003.
- ↑ 64.0 64.1 64.2 J. C. Pearl; B. J. Conrath; R. A. Hanel; J. A. Pirraglia (March 1990). "The albedo, effective temperature, and energy balance of Uranus, as determined from Voyager IRIS data". Icarus 84 (03): 12-28. doi:10.1016/0019-1035(90)90155-3. http://adsabs.harvard.edu/abs/1990Icar...84...12P.
- ↑ G. F. Lindal; J. R. Lyons; D. N. Sweetnam; V. R. Eshleman; D. P. Hinson; G. L. Tyler (30 December 1987). "The atmosphere of Uranus: Results of radio occultation measurements with Voyager 2". Journal of Geophysical Research Space Physics 92 (A13): 14987-15001. doi:10.1029/JA092iA13p14987. http://onlinelibrary.wiley.com/doi/10.1029/JA092iA13p14987/abstract.
- ↑ 66.0 66.1 66.2 66.3 Tyler, J.L.; Sweetnam, D.N.; Anderson, J.D.; Campbell, J. K.; Eshleman, V. R.; Hinson, D. P.; Levy, G. S.; Lindal, G. F. et al. (1986). "Voyger 2 Radio Science Observations of the Uranian System: Atmosphere, Rings, and Satellites". Science 233 (4759): 79–84. doi:10.1126/science.233.4759.79. PMID 17812893.
- ↑ 67.0 67.1 67.2 67.3 67.4 J. Bishop; S. K. Atreya; F. Herbert; P. Romani (December 1990). "Reanalysis of Voyager 2 UVS occultations at Uranus - Hydrocarbon mixing ratios in the equatorial stratosphere". Icarus 88 (12): 448-64. doi:10.1016/0019-1035(90)90094-P. http://adsabs.harvard.edu/abs/1990Icar...88..448B.
- ↑ 68.0 68.1 68.2 Michael E. Summers; Darrell F. Strobel (1 November 1989). "Photochemistry of the atmosphere of Uranus". The Astrophysical Journal 346 (11): 495-508. doi:10.1086/168031. http://adsabs.harvard.edu/abs/1989ApJ...346..495S.
- ↑ 69.0 69.1 69.2 69.3 69.4 Martin Burgdorf; Glenn Orton; Jeffrey van Cleve; Victoria Meadows; James Houck (October 2006). "Detection of new hydrocarbons in Uranus' atmosphere by infrared spectroscopy". Icarus 184 (02): 634-7. doi:10.1016/j.icarus.2006.06.006. http://adsabs.harvard.edu/abs/2006Icar..184..634B. Retrieved 2016-06-28.
- ↑ 70.0 70.1 70.2 Thérèse Encrenaz (February 2003). "ISO observations of the giant planets and Titan: what have we learnt?". Planetary and Space Science 51 (2): 89-103. doi:10.1016/S0032-0633(02)00145-9. http://adsabs.harvard.edu/abs/2003P%26SS...51...89E. Retrieved 2016-06-28.
- ↑ 71.0 71.1 Th. Encrenaz; E. Lellouch; P. Drossart; H. Feuchtgruber; G. S. Orton; S. K. Atreya (January 2004). "First detection of CO in Uranus". Astronomy and Astrophysics 413 (01): L5-9. doi:10.1051/0004-6361:20034637. http://adsabs.harvard.edu/abs/2004A%26A...413L...5E. Retrieved 2016-06-28.
- ↑ 72.0 72.1 Imke de Pater (14 November 2014). Astronomers Spot Large Storms on Uranus. Sci-News.com. http://www.sci-news.com/astronomy/science-large-storms-uranus-02272.html. Retrieved 2014-11-25.
- ↑ Heidi Hammel (14 November 2014). Astronomers Spot Large Storms on Uranus. Sci-News.com. http://www.sci-news.com/astronomy/science-large-storms-uranus-02272.html. Retrieved 2014-11-25.
- ↑ Imke de Pater; Paul N. Romani; Sushil K. Atreya (December 1989). "Uranus deep atmosphere revealed". Icarus 82 (12): 288-313. doi:10.1016/0019-1035(89)90040-7. http://adsabs.harvard.edu/abs/1989Icar...82..288D. Retrieved 2016-06-28.
- ↑ 75.0 75.1 75.2 75.3 75.4 75.5 Herbert, Floyd; Sandel, B. R.; Yelle, R. V.; Holberg, J. B.; Broadfoot, A. L.; Shemansky, D. E.; Atreya, S. K.; Romani, P. N. (December 30, 1987). "The Upper Atmosphere of Uranus: EUV Occultations Observed by Voyager 2". Journal of Geophysical Research 92 (A13): 15,093–15,109. doi:10.1029/JA092iA13p15093. http://www-personal.umich.edu/~atreya/Articles/1987_Upper_Atm_Uranus.pdf.
- ↑ 76.0 76.1 Young, Leslie A.; Bosh, Amanda S.; Buie, Marc; Elliot, J. L.; Wasserman, Lawrence H. (2001). et al. 2001Uranus.pdf "Uranus after Solstice: Results from the 1998 November 6 Occultation". Icarus 153 (2): 236–247. doi:10.1006/icar.2001.6698. http://www.boulder.swri.edu/~layoung/eprint/ur149/Young et al. 2001Uranus.pdf.
- ↑ L. M. Trafton; S. Miller; T. R. Geballe; J. Tennyson; G. E. Ballester (October 1999). "H2 Quadrupole and H+3 Emission from Uranus: The Uranian Thermosphere, Ionosphere, and Aurora". The Astrophysical Journal 524 (2): 1059-83. doi:10.1086/307838. http://adsabs.harvard.edu/abs/1999ApJ...524.1059T. Retrieved 2016-06-28.
- ↑ Th. Encrenaz; P. Drossart; G. Orton; H. Feuchtgruber; E. Lellouch; S. K. Atreya (December 2003). "The rotational temperature and column density of H3+ in Uranus". Planetary and Space Science 51 (14-15): 1013-6. doi:10.1016/j.pss.2003.05.010. http://adsabs.harvard.edu/abs/2003P%26SS...51.1013E. Retrieved 2016-06-28.
- ↑ 79.0 79.1 79.2 79.3 79.4 Floyd Herbert; Doyle T. Hall (May 1996). "Atomic hydrogen corona of Uranus". Journal of Geophysical Research 101 (A5): 10,877–10,885. doi:10.1029/96JA00427.
- ↑ Eso0237b (20 December 2002). Uranus with rings and moons. Chile: ESO. http://www.eso.org/public/images/eso0237b/. Retrieved 2015-04-09.
- ↑ Daphne Stam; Markus Hartung; Mark Showalter; Imke de Pater (23 August 2007). "Peering at Uranus's rings as they swing edge-on to Earth for the first time since their discovery in 1977". Paranal, Chile: European Southern Observatory (ESO). Retrieved 12 April 2021.
- ↑ 82.0 82.1 NASA/JPL (January 24, 1986). Miranda - Highest Resolution Color Picture. Pasadena, California USA: NASA/JPL. http://www.ciclops.org/view/3454/Miranda_-_Highest_Resolution_Color_Picture. Retrieved 2013-03-31.
- ↑ Sailormoon Terms and Information. The Sailor Senshi Page. http://www.eternalsailormoon.org/help.html#myth. Retrieved March 5, 2006.
- ↑ "Asian Astronomy 101". Hamilton Amateur Astronomers 4 (11). 1997. http://amateurastronomy.org/EH/Oct97.txt. Retrieved August 5, 2007.
- ↑ MIRA's Field Trips to the Stars Internet Education Program, In: Monterey Institute for Research in Astronomy. http://www.mira.org/fts0/planets/101/text/txt001x.htm. Retrieved August 27, 2007.
- ↑ Immanuel Velikovsky. Uranus. The Immanuel Velikovsky Archive. http://www.varchive.org/itb/uranus.htm#f_1. Retrieved 2013-01-14.
- ↑ Planet symbols, In: NASA Solar System exploration. http://solarsystem.nasa.gov/multimedia/display.cfm?IM_ID=167. Retrieved August 4, 2007.
- ↑ AETHER: Greek protogenos god of upper air & light ; mythology : AETHER. Theoi.com. http://www.theoi.com/Protogenos/Aither.html.
- ↑ AETHER: Greek protogenos god of upper air & light ; mythology : AETHER. Theoi.com. http://www.theoi.com/Protogenos/Aither.html.
- ↑ Varro, De lingua Latina 5.58.
- ↑ Cicero, De natura deorum 3.44, as cited by E.J. Kenney, Apuleius: Cupid and Psyche (Cambridge University Press, 1990, 2001), note to 6.6.4, p. 198; Hyginus, preface. This is not the theogony that Hesiod presents.
- ↑ Cicero, De natura Deorum 3.56; also Arnobius, Adversus Nationes 4.14.
- ↑ Ennius, Annales 27 (edition of Vahlen); Varro, as cited by Nonius Marcellus, p. 197M; Cicero, Timaeus XI; Arnobius, Adversus Nationes 2.71, 3.29.
- ↑ Arnobius, Adversus Nationes 4.14.
- ↑ Doro Levi, "Aion," Hesperia 13.4 (1944), p. 274.
- ↑ Levi, "Aion," p. 274.
- ↑ Levi, "Aion," p.
- ↑ P. Kenneth Seidelmann; B. A. Archinal; M. F. A'hearn; A. Conrad; G. J. Consolmagno D. Hestroffer, J. L. Hilton, G. A. Krasinsky, G. Neumann (2007). "Report of the IAU/IAG Working Group on cartographic coordinates and rotational elements: 2006". Celestial Mechanics and Dynamical Astronomy 98 (3): 155-80. doi:10.1007/s10569-007-9072-y.
- ↑ J. Herschel (June 1918). "The poles of planetary orbits". The Observatory 41: 255-7. http://adsabs.harvard.edu/full/1918Obs....41..255H. Retrieved 2013-07-10.
- ↑ Thommes, E. W.; Duncan, M. J.; Levison, H. F. (May 2002). "The Formation of Uranus and Neptune among Jupiter and Saturn". The Astronomical Journal 123 (5): 2862-2883. doi:10.1086/339975. https://iopscience.iop.org/article/10.1086/339975. Retrieved 12 April 2021.
- ↑ "Une prédiction forte du « Modèle de Nice » validée par la sonde Rosetta". Retrieved 2020-09-04.
- ↑ Hansen, Kathryn (July 6, 2005). "Orbital shuffle for early solar system". Retrieved 26 August 2007.
- ↑ A. Crida (2009). "Solar System formation". Reviews in Modern Astronomy 21: 3008. doi:10.1002/9783527629190.ch12. ISBN 978-3-527-62919-0.
- ↑ Desch, S.J. (2007). "Mass Distribution and Planet Formation in the Solar Nebula". The Astrophysical Journal 671 (1): 878–93. doi:10.1086/522825. http://pdfs.semanticscholar.org/054e/675d459212463f2ec9770f858eaaf3c4505b.pdf.
- ↑ Smith, R.; L.J. Churcher; M.C. Wyatt; M.M. Moerchen (2009). "Resolved debris disc emission around η Telescopii: a young solar system or ongoing planet formation?". Astronomy and Astrophysics 493 (1): 299–308. doi:10.1051/0004-6361:200810706.
- ↑ 106.0 106.1 106.2 106.3 106.4 106.5 106.6 K. Tsiganis, R. Gomes, A. Morbidelli & H. F. Levison (26 May 2005). "Origin of the orbital architecture of the giant planets of the Solar System". Nature 435-461: 459-. doi:10.1038/nature03539. https://www-n.oca.eu/morby/papers/nature-papers-5-26-05.pdf. Retrieved 12 April 2021.
- ↑ tweet about chinese plans
External links
[edit | edit source]- Google Books
- Google scholar Advanced Scholar Search
- International Astronomical Union
- NASA's National Space Science Data Center
- SAGE journals online
- The SAO/NASA Astrophysics Data System
- Scirus for scientific information only advanced search
- SIMBAD Astronomical Database
- SIMBAD Web interface, Harvard alternate
- Spacecraft Query at NASA
- Universal coordinate converter