Stars/Astronomy

A star is a massive, luminous sphere of plasma held together by gravity.
Def.# of "or pertaining to a large mass; weighty, heavy, or bulky",[1]
is called massive.
Astronomy
[edit | edit source]A nomy (Latin nomia) is a "system of laws governing or [the] sum of knowledge regarding a (specified) field."[3]
When any effort to acquire a system of laws or knowledge focusing on an astr, aster, or astro, that is, any natural body in the sky especially at night,[3] succeeds even in its smallest measurement, astronomy is the name of the effort and the result.
While natural objects in the sky, especially at night, may be sensed by sight, sound, smell, taste, or touch (vibration), many have been seen from the light they emit, absorb, reflect, transmit, or fluoresce.
Some of the natural emitters are stars.
Stars
[edit | edit source]Def. a "luminous celestial body, made up of plasma (particularly hydrogen and helium) and having a spherical shape"[4] is called a star.
"Depending on context the sun may or may not be included."[4]
Def.
- 1.a: "any natural luminous body visible in the sky [especially] at night",[3]
- 1.b: "a self-luminous gaseous celestial body of great mass whose shape is [usually] spheroidal and whose size may be as small as the earth or larger than the earth's orbit".[3]
is called a star.
Colors
[edit | edit source]

"Our Sun itself frequently has sunspots, relatively cool dark magnetic depressions that move across its surface. HD 12545, however, exhibits the largest starspots yet observed. Doppler imaging - the use of slight changes in color caused by the rotation of the star - was used to create this false-color image. The vertical bar on the right gives a temperature scale in kelvins. This giant, binary, RS CVn star, also known as XX Trianguli, is visible with binoculars in the constellation of Triangulum. The starspot is thought to be caused by large magnetic fields that inhibit hot matter from flowing to the surface."[5]
"A giant starspot was revealed on the K0 giant star XX Triangulum, also known as HD12545, using Doppler imaging on the Kitt Peak National Observatory's 0.9m Coude Feed telescope. This picture shows a series of views around the star".[6]
"To observe spots on the surfaces of other stars, astronomers need to "resolve" the stellar disk. This cannot be done directly with the largest telescopes even planned, but Doppler imaging can be used to obtain a map of inhomogeneities on a star's surface. The principle is similar to medical tomography, but instead of a scanner rotating around a fixed object, a rotating star is observed with a fixed telescope. A cool starspot rotating into view at the preceding limb of the star causes a blue-shifted asymmetry in each spectral line profile. This asymmetry moves into the line center at the time of meridian passage, and turns into a red-shifted asymmetry after meridian passage. The asymmetry fades away when the spot disappears at the receding limb. The higher the latitude of the spot, the shorter will be its visible path across the projected disk of the star, or the spot may even be circumpolar if the stellar rotation axis is inclined. All this information is hidden in the variation of the spectral line profiles and is reconstructed by mathematical inversion to create a true picture of the stellar surface. For a successful application, the telescope needs to "see" the entire stellar surface during at least one stellar rotation."[7]
"XX Triangulum is an active K0 giant binary star, approximately 10 times larger and twice as massive as the Sun. Its rotation period is 24 days, so that 24 consecutive (clear) nights of telescope time with an excellent high-resolution optical spectrograph are needed to obtain a good Doppler image. Because starspots vary on the same (short) time scales as Sunspots do (they are stable for about one stellar rotation), all the observations must be made on one rotation cycle. NSF's Kitt Peak National Observatory is one of the few facilities worldwide that offers this capability with the 0.9-m coude feed telescope."[7]
"During the observations, XX Triangulum had its brightest magnitude since the discovery of its light variability in 1985 and showed the largest photometric amplitude so far (0.63 magnitudes in V). The large photometric amplitude was explained when the Doppler-imaging inversion algorithm also recovered a not-quite-as-large equatorial warm spot (350 K above the photospheric temperature) in the hemisphere opposite the dark spot. Strassmeier speculates that the warm spot harbors the same magnetic field as the cool spot but opposite polarity. Surprisingly, the fact that the star was brighter at a time of high spot activity is in agreement with the solar analogy despite the "unsolar" dimension of the gigantic spot."[7]
Theoretical stellar astronomy
[edit | edit source]As stars are defined as luminous balls of plasma, the Sun may not qualify as its photosphere has a plasma concentration of approximately 10-4. The rest is composed of neutral atoms at about 5800 K.
Photospheres
[edit | edit source]
Def. a "visible surface layer of a star, and especially that of a sun"[8] is called a photosphere.
"When we speak of the surface of the Sun, we normally mean the photosphere."[9] "[T]he photosphere may be thought of as the imaginary surface from which the solar light that we see appears to be emitted. The diameter quoted for the Sun usually refers to the diameter of the photosphere."[9] The photosphere emits visual, or visible, radiation.
The solar photosphere is a "weakly ionized [ni/(ni + na)] ~ 10-4, relatively cold and dense plasma".[10]
Stellar active regions
[edit | edit source]
A stellar active region is "[a] localized, transient volume of [a stellar] atmosphere in which plages, [star]spots, faculae, flares, etc., may be observed. Active regions are the result of enhanced magnetic fields; they are bipolar and may be complex if the region contains two or more bipolar groups."[11]
A stellar active region on a star's surface can form a bright spot which intensifies and grows. An active region may have a coronal portion.
Most stellar flares and coronal mass ejections originate in magnetically active regions around visible sunspot groupings. Similar phenomena indirectly observed on stars are commonly called starspots and both light and dark spots have been measured.[12]
Hypervelocity stars
[edit | edit source]



"To date, all of the reported hypervelocity stars (HVSs), which are believed to be ejected from the Galactic center, are blue and therefore almost certainly young.”[13]
Def. a high-velocity star moving through space with an abnormally high velocity relative to the surrounding interstellar medium is called a runaway star.
"Of particular importance has been access to high resolution R~40,000-100,000 echelle spectra providing an ability to study the dynamics of hot plasma and separate multiple stellar and interstellar absorption components."[14]
At left is a radiated object, the binary star Mira, and its associated phenomena.
"Ultra-violet studies of Mira by NASA's Galaxy Evolution Explorer (Galex) space telescope have revealed that it sheds a trail of material from the outer envelope, leaving a tail 13 light-years in length, formed over tens of thousands of years.[15][16] It is thought that a hot bow-wave of compressed plasma/gas is the cause of the tail; the bow-wave is a result of the interaction of the stellar wind from Mira A with gas in interstellar space, through which Mira is moving at an extremely high speed of 130 kilometres/second (291,000 miles per hour).[17][18] The tail consists of material stripped from the head of the bow-wave, which is also visible in ultra-violet observations. Mira's bow-shock will eventually evolve into a planetary nebula, the form of which will be considerably affected by the motion through the interstellar medium (ISM).[19]
At second right is the only available X-ray image, by the Chandra X-ray Observatory, of Mira A on the right and Mira B (left). "Mira A is losing gas rapidly from its upper atmosphere [apparently] via a stellar wind. [Mira B is asserted to be a white dwarf. In theory] Mira B exerts a gravitational tug that creates a gaseous bridge between the two stars. Gas from the wind and bridge accumulates in an accretion disk around Mira B and collisions between rapidly moving particles in the disk produce X-rays."[20]
Mira A, spectral type M7 IIIe[21], has an effective surface temperature of 2918–3192[22]. Mira A is not a known X-ray source according to SIMBAD, but here is shown to be one.
Neutron stars
[edit | edit source]
It is a type of stellar remnant [(a compact star)] that can result from the gravitational collapse of a massive star during a Type II, Type Ib or Type Ic supernova event. Such stars are composed almost entirely of neutrons
Neutron stars are theorized as the radiation source for anomalous X-ray pulsars (AXPs), binary pulsars, high-mass X-ray binaries, intermediate-mass X-ray binaries, low-mass X-ray binaries (LMXB), pulsars, and soft gamma-ray repeaters (SGRs).
"The [image on the right] shows two observations of [the] neutron star [RX J0822-4300] obtained with the Chandra X-ray Observatory over the span of five years, between December 1999 [on the left] and April 2005 [on the right]. By combining how far it has moved across the sky with its distance from Earth [at about 7,000 light years], astronomers determined the cosmic cannonball is moving at over 3 million miles per hour, one of the fastest moving stars ever observed. At this rate, RX J0822-4300 [at (J2000) RA 08h 23m 08.16s Dec -42° 41' 41.40" in Puppis] is destined to escape from the Milky Way after millions of years, even though it has only traveled about 20 light years so far."[23]
Electron stars
[edit | edit source]"IN the standard model for type Ia supernovae1, a massive white dwarf in a binary system accretes matter from the companion star until it reaches the Chandrasekhar mass (the stability limit for degenerate-electron stars, corresponding to ~1.4 solar masses), and a runaway thermonuclear explosion ensues."[24]
Neutrino stars
[edit | edit source]"In the 1980s two early water-Cherenkov experiments were built. The Irvine-Michigan-Brookhaven detector in an Ohio salt mine and the Kamiokande detector in a Japanese zinc mine were tanks containing thousands of tons of purified water, monitored with phototubes. The two detectors launched the field of neutrino astronomy by detecting some 20 low-energy (about 10 MeV) neutrinos from Supernova 1987A—the first supernova since the 17th century that was visible to the naked eye."[25]
"The water-based detectors Kamiokande II and IMB detected 11 and 8 antineutrinos of thermal origin,[26] respectively, while the scintillator-based Baksan detector found 5 neutrinos (lepton number = 1) of either thermal or electron-capture origin, in a burst lasting less than 13 seconds.
"In 1987, astronomers counted 19 neutrinos from an explosion of a star in the nearby Large Magellanic Cloud, 19 out of the billion trillion trillion trillion trillion neutrinos that flew from the supernova."[27]
Gamma-ray stars
[edit | edit source]Gamma-ray stars have surface temperatures starting at 300,000,000 K (300 MK) corresponding to a peak wavelength of 0.010 nm (10 pm) for the beginning of super soft gamma-ray sources.
X-ray stars
[edit | edit source]
X-ray stars have surface temperatures starting at 300,000 K corresponding to a peak wavelength of 10 nm for the beginning of super soft X-ray sources.
Ultraviolet stars
[edit | edit source]
Stellar class O stars have surface temperatures high enough that most of their luminescence is in the ultraviolet.
Violet stars
[edit | edit source]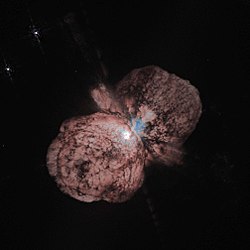
"The “Purple Haze” is a diffuse blueish/purple glow within a few arcseconds of the central star in HST images of the Homunculus (Morse et al. 1998; Smith et al. 2000, 2004). This emission is seen in excess of violet starlight scattered by dust, and the strength of the excess increases into the far UV (Smith et al. 2004; hereafter Paper I)."[28]
Notation: let the symbol LH stand for the Little Homunculus.
"The LH has no outstanding correspondence with any of the clumps and filaments seen in scattered light in normal UV or visual-wavelength images of η Car, although it does match the spatial extent of the "Purple Haze"".[29] Bold added.
The Fe II emission line at 489.1 nm occurs in the Little Homunculus (Eta Carinae)[29]
Mass "loss at the η Carinae rate produces considerable changes of Teff on a human timescale. As an example, for the 120 Mʘ model, a change from about 20,000 K to 34,000 K was obtained over a time of 50 yr as a result of the secular bluewards evolution following the rapid ejection."[30]
Blue stars
[edit | edit source]
Stars are often referred to by their predominant color. For example, blue stragglers are found among the galactic halo globular clusters.[32] Blue main sequence stars, that are metal poor, ([Fe/H] ≤ -1.0) are most likely not analogous to blue stragglers.[32]
Cyan stars
[edit | edit source]
Theta Ursae Majoris is a spectral type F7V star.[33] It has a surface temperature of 6300 ± 33 K.[34] Such an effective surface temperature has a Planckian black body peak wavelength of 476 nm which places this star at the high temperature end of the cyan band.
Yellow stars
[edit | edit source]
A subgiant star is a star that is slightly brighter than a normal main-sequence (dwarf) star of the same spectral class, but not as bright as true giant stars. Although certain subgiants appear to be simply unusually bright metal-rich hydrogen-fusing stars (in the same way subdwarfs are unusually dim metal-poor hydrogen-fusing stars), they are generally believed to be stars that are ceasing or have already ceased fusing hydrogen in their cores.
"Many subgiants are rich in metals, and commonly host orbiting planets.
At right is a visual image in close to true color of V972 Scorpii, which is a variable star of the delta Sct type. It has spectral type G2IV and is a star in a cluster. The system includes components CCDM J16234-2622 A and CCDM J16234-2622 B. Component A is a dwarf star in a double star system with component B. Component A is apparently V972 Scuti.
Orange stars
[edit | edit source]
The variability of BD +50 961 (SY Persei, an orange star) is confirmed.[35]
"ESO Photo Ambassador Babak Tafreshi snapped this remarkable image [at right] of the antennas of the Atacama Large Millimeter/submillimeter Array (ALMA), set against the splendour of the Milky Way. The richness of the sky in this picture attests to the unsurpassed conditions for astronomy on the 5000-metre-high Chajnantor plateau in Chile’s Atacama region."[36]
"This view shows the constellations of Carina (The Keel) and Vela (The Sails). The dark, wispy dust clouds of the Milky Way streak from middle top left to middle bottom right. The bright orange star in the upper left is Suhail in Vela, while the similarly orange star in the upper middle is Avior, in Carina. Of the three bright blue stars that form an “L” near these stars, the left two belong to Vela, and the right one to Carina. And exactly in the centre of the image below these stars gleams the pink glow of the Carina Nebula"[36]
Red stars
[edit | edit source]
“A red giant is a luminous giant star The outer atmosphere is inflated and tenuous, making the radius immense and the surface temperature low, somewhere from 5,000 K and lower. The appearance of the red giant is from yellow orange to red, including the spectral types K and M, but also class S stars and most carbon stars. The most common red giants are the so-called red giant branch stars (RGB stars) ... Another case of red giants are the asymptotic giant branch stars (AGB) ... To the AGB stars belong the carbon stars of type C-N and late C-R. ... The stellar limb of a red giant is not sharply-defined, as depicted in many illustrations. Instead, due to the very low mass density of the envelope, such stars lack a well-defined photosphere. The body of the star gradually transitions into a 'corona' with increasing radii.[37]
Infrared stars
[edit | edit source]
"Many celestial objects that can’t be seen by humans immediately become visible when using an infrared camera. The picture on the [right] – of the Trapezium star cluster in the Orion Nebula – was taken by Philip Lucas (Univ. Hertfordshire) and Patrick Roche (Univ. Oxford) with an IR camera at wavelengths roughly twice as long as visible light in the electromagnetic spectrum. [...] Without infrared radiation this star cluster would not have been visible, along with other objects such as clouds of particles around stars, infrared galaxies, interstellar molecules, cool stars, brown dwarfs and planets."[38]
A stars
[edit | edit source]
Vega is a nearby optical spectral type A0V star at about 25 lyrs. Its polar effective temperature is near 10,000 K, while its equatorial effective temperature is 7,600 K.
B stars
[edit | edit source]Notation: Let sdB stand for subdwarf B stars.
The "pulsating sdB star KPD 1930 +2752 [...] is a binary. The radial velocities measured from the Hα and He I 6678-Å spectral lines vary sinusoidally with the same period (2 h 17 min) as the ellipsoidal variability seen by Billéres et al. The amplitude of the orbital motion (349.3 ± 2.7 km s-1) combined with the canonical mass for sdB stars (0.5 M⊙) implies a total mass for the binary of 1.47 ± 0.01 M⊙. The unseen companion star is almost certainly a white dwarf star. The binary will merge within ~200 million years because of gravitational wave radiation. The accretion of helium and other elements heavier than hydrogen on to the white dwarf, which then exceeds the Chandrasekhar mass (1.4 M⊙), is a viable model for the cause of Type Ia supernovae."[39]
C stars
[edit | edit source]
"A bright star [in the image at left] is surrounded by a tenuous shell of gas in this unusual image from the NASA/ESA Hubble Space Telescope. U Camelopardalis, or U Cam for short, is a star nearing the end of its life. As it begins to run low on fuel, it is becoming unstable. Every few thousand years, it coughs out a nearly spherical shell of gas as a layer of helium around its core begins to fuse. The gas ejected in the star’s latest eruption is clearly visible in this picture as a faint bubble of gas surrounding the star."[40]
"U Cam is an example of a carbon star. This is a rare type of star whose atmosphere contains more carbon than oxygen. Due to its low surface gravity, typically as much as half of the total mass of a carbon star may be lost by way of powerful stellar winds."[40]
"Located in the constellation of Camelopardalis (The Giraffe), near the North Celestial Pole, U Cam itself is actually much smaller than it appears in Hubble’s picture. In fact, the star would easily fit within a single pixel at the centre of the image. Its brightness, however, is enough to overwhelm the capability of Hubble’s Advanced Camera for Surveys making the star look much bigger than it really is."[40]
"The shell of gas, which is both much larger and much fainter than its parent star, is visible in intricate detail in Hubble’s portrait. While phenomena that occur at the ends of stars’ lives are often quite irregular and unstable (see for example Hubble’s images of Eta Carinae, potw1208a), the shell of gas expelled from U Cam is almost perfectly spherical."[40]
"The image was produced with the High Resolution Channel of the Advanced Camera for Surveys [using the 606 nm and 814 nm filters]."[40]
D stars
[edit | edit source]
EG 5 is a yellow degenerate [DZ8].[41] EG 5 is another designation for Van Maanen's star.[42]
"Van Maanen's star (van Maanen 2) is a white dwarf star. Out of the white dwarfs known, it is the third closest to the Sun, after Sirius B and Procyon B, in that order, and the closest known solitary white dwarf.[43][44] Van Maanen's star has a radius of 9,000 ± 1,400 km.[45] It's effective surface temperature is 6,220 ± 240 K.[46]
Degenerate stars are white dwarfs of spectral luminosity class VII.
Some yellow degenerate stars are of white dwarf spectral type DC (which show no detectable lines) mostly below Teff < 10,000 K.[41]
E stars
[edit | edit source]A B(e)-type star is a B-type star with distinctive forbidden neutral or low ionization emission lines in its spectrum.
F stars
[edit | edit source]
"The bright southern hemisphere star RS Puppis, at the center of the image, is swaddled in a gossamer cocoon of reflective dust illuminated by the glittering star. The super star is ten times more massive than our sun and 200 times larger."[47]
"RS Puppis rhythmically brightens and dims over a six-week cycle. It is one of the most luminous in the class of so-called Cepheid variable stars. Its average intrinsic brightness is 15,000 times greater than our sun’s luminosity."[47]
"The nebula flickers in brightness as pulses of light from the Cepheid propagate outwards. Hubble took a series of photos of light flashes rippling across the nebula in a phenomenon known as a "light echo." Even though light travels through space fast enough to span the gap between Earth and the moon in a little over a second, the nebula is so large that reflected light can actually be photographed traversing the nebula."[47]
"By observing the fluctuation of light in RS Puppis itself, as well as recording the faint reflections of light pulses moving across the nebula, astronomers are able to measure these light echoes and pin down a very accurate distance. The distance to RS Puppis has been narrowed down to 6,500 light-years (with a margin of error of only one percent)."[47]
G stars
[edit | edit source]
HD209458 is the brightest star in the image on the right and it is a G0V.
H stars
[edit | edit source]H stars, or C-H stars, are carbon stars with very strong CH absorption.
I stars
[edit | edit source]Population I stars are metal-rich stars. They have the highest metallicity of all stars.
Population II stars are metal-poor stars. They have the lowest metallicity of all stars.
Population III stars have no metals. They have no metallicity.
J stars
[edit | edit source]J stars are carbon stars with enhanced 13C.[48]
K stars
[edit | edit source]
The image on the right is centered on GQ Lupi which is a K7V star. The feeble point of light to the right of the star is the newly found cold companion. It is 250 times fainter than the star itself and it is located 0.73" west. At the distance of GQ Lupi, this corresponds to a distance of roughly 100 AU. North is up and East is to the left.
L stars
[edit | edit source]
A "new spectral class L [has the major spectroscopic signatures of] metallic oxides [...] replaced by metallic hydrides and neutral alkali metals [...]."[49]
"At least five of the 20 2MASS L dwarfs show the 6708 Å lithium doublet at low resolution, the strongest having an equivalent width of 18.5 Å."[49]
"The Ks filter is a modified K filter that reduces the thermal background for warm ground-based telescopes."[50]
The J band is centered on 1.25 µm in the near-infrared. The K band covers 1.67 – 1.11 cm in the microwaves. R band refers to the red band of the visible spectrum.
M stars
[edit | edit source]N stars
[edit | edit source]Y Canum Venaticorum is a spectral type C54J(N3) giant red carbon star.
O stars
[edit | edit source]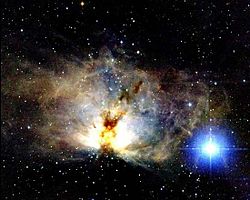
O stars are the hottest stars.
The peaks of their Planckian spectra start at about 38,000 K and increase, and 79 nm and decrease in the ultraviolet.
Alnitak is the blue star in lower right corner of the image on the right. It is an O9.7Ib.
P stars
[edit | edit source]Primordial stars, P stars, have "low Na/Al/N and high O/Mg/C".[51]
Q stars
[edit | edit source]Q stars are stars "in which high-density, electrically neutral baryonic matter is a coherent classical solution to an effective field theory of strong forces and is bound in the absence of gravity."[52]
R stars
[edit | edit source]"The R stars, on the other hand [to the N-type carbon stars], have warmer temperatures, and blue/violet light is accessible to observation, and atmospheric analysis."[53]
In "the warm R0—R4 stars, C is overabundant on average by approximately 0.7 dex relative to the sun and to normal G and K giants. On the other hand, [...] the O abundance is normally near solar, and the N abundance just slightly enhanced. At CNO cycle equilibrium both C and O are depleted substantially. The lack of oxygen and carbon depletion, [leads to] the CNO cycle operating near equilibrium is not responsible for the fact that C/O > 1 in these stars. The excess carbon has to come from some other process."[53]
The "s process element abundances are nearly solar [...] This is in sharp contrast with most other carbon and carbon-related stars (see reviews by McClure 1984a, 1985). The N, S, barium, CH, sgCH, and dwarf carbon stars all have significantly enhanced abundances of the s process elements relative to iron (e.g., Lambert 1985; Green and Margon 1994). The N stars and many of the S stars are assumed to have undergone helium-shell flashing on the asymptotic giant branch (AGB), and the third dredge-up phase has brought carbon and s-process elements to the surface (e.g., see Iben and Renzini 1983)."[53]
"Like many of the barium, CH, sgCH, and dwarf carbon stars, the warmer R stars are too faint to be on the AGB."[53]
The "R0—R4 stars lie in a similar temperature/luminosity domain as the barium stars. They are well below the lower-luminosity boundary of the onset of helium-shell flashing. The lack of s process enhancement and low luminosity led Dominy (1984) to conclude that the R stars probably received their enhanced carbon from the helium-core flash at the tip of the first ascent giant branch."[53]
"Eight stars in the sample are classified as R5-R9 [...] These stars again are well up on the giant branch, and they exhibit a similar velocity jitter. The average of the standard deviations for the individual observations in this case, is ±1.60 km s-1. Again, there is no convincing evidence for binary motion. The star with the largest deviations, HD 59643, is the coolest star in the sample, with a classification of R9."[53]
"Many R stars also have quite strong CH, however (see Yamashita 1972, Fig. 10e), and at low dispersion where the strengths of narrow tines are difficult to estimate, a CH star may look very similar to an R star. The CH stars are mostly high-velocity-halo objects, whereas the R stars belong to the low-velocity-old-disk population (McLeod 1947)."[53]
S stars
[edit | edit source]Stars "of spectral type S are characterized by unusual photospheric abundances which imply enrichment of the stellar surface by nucleosynthesis products. Spectroscopically, S stars are identified by bands of ZrO and LaO, replacing the TiO bands found in M stars. The spectra of S stars indicate strong enhancement of s-process elements in the photosphere (an accident of nomenclature - when the S spectral type was introduced, the slow neutron capture process was unknown). Abundance analyses show that in S stars, the C/O ratio is very close to unity [...], which also implies the presence of the products of nucleosynthesis at the stellar surface."[54]
The "extrinsic S stars, includes stars which have elemental abundances which appear to have been altered by mass transfer from a binary companion."[54]
The "intrinsic S stars, includes stars which have high luminosity and lie on the asymptotic giant branch (AGB). They show evidence that their compositional abnormalities are a result of nucleosynthesis and [perhaps] convective mixing to the surface. In particular, a defining characteristic which distinguishes the two types is that the intrinsic S stars contain technetium, while the extrinsic S stars do not."[54]
"Both HCN and SiO have readily observable lines at 3 mm."[54] χ Cyg is at a distance of 170 pc, but parallax measurements put it at D = 144 ± 25 pc (Stein 1991), parallax of 5.53 mas (198 ± 38) pc as of 2007 according to SIMBAD.
"All of these stars are bright at 2 µm and therefore have circumstellar dust [...] In one observing session, we obtained a 5 x 5 cross at HPBW spacings for the star χ Cyg in the CO J = 2-1 line. The data were relatively noisy because of limited integration time and weather conditions but do indicate that the envelope is extended with respect to the 25" telescope beam."[54]
χ Cyg was "detected in the SiO v = 1 J = 2-1 maser emission line [...] χ Cyg has an unusually large dust/gas ratio of 9.0 x 10-3 [The dust-to-gas ratio for S stars detected in CO J = 1-0 is] 9.0 x 10-3 [...] For one star in our sample namely χ Cyg, the SiO J = 2-1, v = 0 emission has been mapped interferometrically [...] the SiO abundance at the base of the expanding envelope must be ~ 2 x 10-5 to explain the observed intensity distribution of the SiO emission. Thus, a substantial fraction (30%-50%) of all silicon atoms are in the form of gas phase SiO at the point where molecules are injected into the stellar wind. As the gas moves away from the star, the SiO is depleted from the gas, presumably by the process of grain formation, such that at radii of several x 1015 cm, the SiO gas phase abundance has fallen by > 90%. [...] χ Cyg, which has a relatively low mass-loss rate and hence low envelope opacity to UV photons."[54]
T stars
[edit | edit source]"Gl 229B--is the prototype of a methane-dominated spectral class, which we propose as class "T". [...] Gl 229B [is a] T dwarf [...] Its spectrum is dominated by methane, much like the Jovian planets."[49]
"As for T dwarfs--which we will define as dwarfs exhibiting methane absorption at the K-band--Oppenheimer et al. (1998) have shown that Gl 229B has few distinguishable features in the far-optical--the two Cs I lines, a weak CH4 band near 8900 Å, and an H2O band around 9300 Å. Moreover, even Gl 229B, at a distance of only 5.8 pc, is exceedingly dim at these wavelengths (R ≈ 23.4 and I ≈ 19.5; Golimowski et al. 1998). Thus, for the T dwarfs we will be forced to rely on near-infrared spectra. Fortunately, the distinguishing feature of T-class objects--methane--has very prominent bands in both the H and K spectral regions."[49]
The derived temperature for Gl 229B is 900-1000 K.[49]
"Gl 229B [shows] strong absorption by CH4."[49]
U stars
[edit | edit source]"[U]ranium and thorium [have been detected] in the very-metal poor halo giant BPS CS 31082-001 [...] From the observed present abundances of Th and U in the star, the expected amount of Pb produced by the decay of 232Th, and 238U alone, over 12-15 Gyr is -0.73±0.17 dex."[55]
These are "very metal-poor stars born in the early days of the Galaxy, and strongly enhanced in r-process elements."[55]
"There is now a general agreement that the fraction of Pb produced by the r-process in the solar system it practically unknown, after the discovery that zero-metal stars can produce a lot of s-lead (Goriely & Siess 2001; Van Eck et al. 2003). This has modified the estimates of the amount of s-lead produced in the Galaxy before the birth of the Sun (Gallino et al 1998), and of the r-lead, obtained by subtracting the s-lead from the total lead. If the r-lead of the solar system were mainly produced by the decay of the actinides, as for CS 31082-001, it is easy to verify that the r-lead in the solar system would be of the order of only 1 to 3% of the total lead."[55]
"This [lead, Pb] abundance is very low, −0.55 ± 0.15 dex in CS 31082-001, about one dex below the former upper limits in CS 22892-052 and BD +17◦ 3248. Also, our result shows that, in the purely r-process enriched photosphere of CS 31082-001, most of lead results from the decay of 232Th, 235U, and 238U. This places a limit on the amount of 235U which has contributed to the production of 207Pb, as well as on that of 234U."[55]
The "newly discovered, very r-process–enhanced metal-poor star HE 1523-0901 ([Fe/H] = -2.95) [has age estimates] based on the radioactive decay of Th and U. The bright (V = 11.1) giant was found among a sample of bright metal-poor stars selected from the Hamburg/ESO Survey. From an abundance analysis of a high-resolution (R = 75,000) VLT/UVES spectrum, we find HE 1523-0901 to be strongly overabundant in r-process elements ([r/Fe] = 1.8). The abundances of heavy neutron-capture elements (Z > 56) measured in HE 1523-0901 match the scaled solar r-process pattern extremely well. [The] strongest optical U line [is] at 3859.57 Å."[56]
U, B, V stars
[edit | edit source]The UBV photometric system, also called the Harold Johnson system (or Johnson-Morgan system), a wide band photometric system for classifying stars according to their colors is the first known standardized photoelectric photometric system, where the letters U, B, and V stand for ultraviolet, blue, and visual magnitudes, which are measured for a star then two subtractions are performed in a specific order to classify it in the system.[57]
A 13 in (330 mm) telescope and the Otto Struve Telescope, the 82 in (2,100 mm) telescope at McDonald Observatory were used to define the system.[57]
"In the 1950–51 winter, Johnson had commenced photometry in three passbands (designated U, V, and V) on the McDonald 13- and 82-inch reflectors [54]."[58]
The filters are selected so that the mean wavelengths of response functions (at which magnitudes are measured to mean precision) are 364 nm for U, 442 nm for B, 540 nm for V with zero points calibrated in the B−V (B minus V) and U−B (U minus B) color indices selecting such A0 main sequence stars which are not affected by interstellar reddening.[57]
These stars correspond with a mean effective temperature (Teff (K)) of between 9727 and 9790 Kelvin, the latter being stars with class A0V.
The UBV system has some disadvantages: the short wavelength cutoff that is the U filter is defined mainly by the terrestrial atmosphere rather than the filter itself; thus, it (and observed magnitudes) can vary with altitude and atmospheric conditions.[59] However, a large number of measurements have been made in this system, including many of the bright stars.[60]
The Johnson-Cousins UBVRI photometric system is a common extension of Johnson's original system that provides redder passbands.[61]
Class | B–V | U–B | V–R | R–I | Teff (K) |
---|---|---|---|---|---|
O5V | –0.33 | –1.19 | –0.15 | –0.32 | 42,000 |
B0V | –0.30 | –1.08 | –0.13 | –0.29 | 30,000 |
A0V | –0.02 | –0.02 | 0.02 | –0.02 | 9,790 |
F0V | 0.30 | 0.03 | 0.30 | 0.17 | 7,300 |
G0V | 0.58 | 0.06 | 0.50 | 0.31 | 5,940 |
K0V | 0.81 | 0.45 | 0.64 | 0.42 | 5,150 |
M0V | 1.40 | 1.22 | 1.28 | 0.91 | 3,840 |
The color index is a simple numerical expression that determines the color of an object, which in the case of a star gives its temperature. To measure the index, one observes the magnitude of an object successively through two different filters, such as U and B, or B and V, where U is sensitive to ultraviolet rays, B is sensitive to blue light, and V is sensitive to visible (green-yellow) light (see also: UBV system). The set of passbands or filters is called a photometric system. The difference in magnitudes found with these filters is called the U-B or B–V color index, respectively. The smaller the color index, the more blue (or hotter) the object is. Conversely, the larger the color index, the more red (or cooler) the object is. This is a consequence of the logarithmic magnitude scale, in which brighter objects have smaller (more negative) magnitudes than dimmer ones. For comparison, the yellowish Sun has a B–V index of 0.656 ± 0.005,[63] while the bluish Rigel has B–V –0.03 (its B magnitude is 0.09 and its V magnitude is 0.12, B–V = –0.03).[64] The passbands most optical astronomers use are the UBVRI filters, where the U, B, and V filters are as mentioned above, the R filter passes red light, and the I filter passes infrared light. ... These filters were specified as particular combinations of glass filters and photomultiplier tubes.
A Photometric system is a set of well-defined passbands (or filters), with a known sensitivity to incident radiation. The sensitivity usually depends on the optical system, detectors and filters used. For each photometric system a set of primary standard stars is provided.
Filter Letter | Effective Wavelength Midpoint λeff For Standard Filter[65] | Full Width Half Maximum[65] | Variant(s) | Description |
---|---|---|---|---|
Ultraviolet | ||||
U | 365nm | 66nm | u, u', u* | "U" stands for ultraviolet. |
Visible | ||||
B | 445nm | 94nm | b | "B" stands for blue. |
V | 551nm | 88nm | v, v' | "V" stands for visual. |
G | g, g' | "G" stands for green (visual). | ||
R | 658nm | 138nm | r, r', R', Rc, Re, Rj | "R" stands for red. |
Near-Infrared | ||||
I | 806nm | 149nm | i, i', Ic, Ie, Ij | "I" stands for infrared. |
W stars
[edit | edit source]"Three filamentary nebulae with shell structure and that apparently contain Wolf-Rayet (W) stars are now known."[66]
"Wolf-Rayet Stars: were classified as W, WN, and WC stars. Class W includes objects showing only a wide emission band at λ 4650 with no obvious variation of intensity within the band."[67]
X stars
[edit | edit source]X-ray stars as noted in that section have photospheric effective surface temperatures ≥ 300,000 K. The X-ray emitting stars in this section are stars with much lower photospheric effective surface temperatures.
"A cluster of young, X-ray-emitting stars is found in the vicinity of η Chamaeleontis from a deep ROSAT high-resolution imager observation. The 12 X-ray sources have prominent (R=5-14) stellar counterparts, including two early-type stars (η Cha and RS Cha) and 10 Li-rich, Hα emission-line, late-type stars (K3-M5)."[68]
"Low-mass pre–main-sequence (PMS) stars are traditionally found in the vicinity of star-forming molecular clouds; e.g., the T Tauri stars clustered around the Orion, ρ Oph, and Tau-Aur clouds. However, recent studies of X-ray–luminous stars found in the ROSAT All-Sky Survey (RASS) have revealed large numbers of young Li-rich, magnetically active, late-type stars that resemble weak-lined T Tauri (WTT) stars but are dispersed over the celestial sphere (Neuhäuser 1997). PMS stars have X-ray emission 10–104 times that seen in most main-sequence stars as a result of enhanced magnetic activity."[68]
Y stars
[edit | edit source]"The proposed spectral class Y encompasses brown dwarfs that have cooled below Teff ~ 500 K; a handful of these cool objects have recently been discovered (Cushing et al. 2011; Kirkpatrick et al. 2012). At these temperatures, NH3 begins to play a more significant role in shaping the near-infrared spectra, and sodium and potassium wane in importance in the optical because they condense into clouds. Appreciable amounts of H2O and NH3 will condense into clouds at Teff ~ 350 K and ~200 K, respectively, and will further alter Y dwarf spectra."[69]
Z stars
[edit | edit source]New "opacity data have been employed in the calculation of an extensive grid of theoretical stellar models for masses ranging from 0.1 to 0.75 M⊙ on the assumption of heavy-element abundances Z = 10-5, 10-4, 10-3, 10-2, and 0.02. The calculations predict that the lowest Z stars should be appreciably hotter than the high Z stars of the same mass, implying that metal-poor low-mass stars will be considerably subluminous compared to their metal-rich counterparts at the same effective temperature."[70]
Hypergiants
[edit | edit source]
"ρ Cas, HR 8752 and IRC+10420, three well-studied yellow hypergiants, are situated at or close to the red border of the [yellow evolutionary] void."[71]
Generally speaking, a yellow hypergiant is a massive star with an extended atmosphere, which can be classified as spectral class from late A to K, with a mass of as much as 20-50 solar masses. Yellow hypergiants, such as Rho Cassiopeiae in the constellation Cassiopeia, have been observed to experience periodic eruptions, resulting in periodic or continuous dimming of the star, respectively. Yellow hypergiants appear to be extremely rare in the universe. Due to their extremely rapid rate of consumption of nuclear fuel, yellow hypergiants generally only remain on the main sequence for a few million years before destroying themselves in a massive supernova or hypernova. Yellow hypergiants are post-red supergiants, rapidly evolving toward the blue supergiant phase.
According to the current physical models of stars, a yellow hypergiant should possess a convective core surrounded by a radiative zone, as opposed to a sun-sized star, which consists of a radiative core surrounded by a convective zone (Seeds, 2005). Due to the extremely high pressures which exist at the core of a yellow hypergiant, portions of the core or perhaps the entire core may be composed of degenerate matter.
These stars have "powerful magnetic fields.
Supergiants
[edit | edit source]
In the image on the right, "Alnitak, Alnilam, and Mintaka, are the bright bluish stars from east to west (left to right) along the diagonal in this gorgeous cosmic vista. Otherwise known as the Belt of Orion, these three blue supergiant stars are hotter and much more massive than the Sun. They lie about 1,500 light-years away, born of Orion's well-studied interstellar clouds. In fact, clouds of gas and dust adrift in this region have intriguing and some surprisingly familiar shapes, including the dark Horsehead Nebula and Flame Nebula near Alnitak at the lower left. The famous Orion Nebula itself lies off the bottom of this star field that covers about 4.5x3.5 degrees on the sky. This image was taken last month with a digital camera attached to a small telescope in Switzerland, and better matches human color perception than a more detailed composite taken over 15 years ago."[72]
Giants
[edit | edit source]
Alpha Microscopii is a spectral type G7III yellow giant star in a double system.
This star has an optical visual companion, CCDM J20500-3347B, of apparent visual magnitude 10.0 approximately 20.4 arcseconds away at a position angle of 166°. It has no physical connection to the star described above.[73]
Subgiants
[edit | edit source]

HD 93250 is a class O4 IV(fc).[74]
Main sequence stars
[edit | edit source]
The closest G2V yellow main sequence star is the Sun.
At right is a visual image in close to true color of the main sequence single star HD 86226. It has a parallax of 22.20 mas, but is not an X-ray source. A substellar companion HD 86226b has been detected.
Dwarf stars
[edit | edit source]
"DH Tauri's companion [...] is a brown dwarf with only 40 times the mass of Jupiter. DH Tauri is a young star only one million years old in the constellation Taurus. It is so young it will not begin nuclear fusion for another one hundred million years. It is 460 light years away and two thirds as massive as the Sun. It's companion is among the coolest and lightest of known brown dwarfs orbiting young stars. If the companion had been less massive it probably would have been a planet."[75]
"Planets weigh less than 13 times the mass of Jupiter. Brown dwarfs are 13 to 80 times more massive than Jupiter."[75]
"An image of the star DH Tauri (abbreviated as DH Tau) [contained] an object 250 times fainter 2.3 arcseconds away. At the distance of DH Tauri (460 light years), this separation is equivalent to 330 [AU]. Although the object was in older images of DH Tauri, its location in the new image revealed that it was not an unrelated background object, but a companion that orbits DH Tauri."[75]
The "surface temperature of the companion is about 2700 to 2800 degrees Kelvin, its surface gravity is 4 times that of Jupiter and its mass is only 40 times larger than Jupiter. This puts the companion in the brown dwarf category."[75]
Subdwarfs
[edit | edit source]Subdwarfs are in luminosity class VI. "[Y]ellow high-velocity subdwarfs are easily confused with white dwarfs in a proper-motion selection."[41]
HD 64090 is a color class G0 subdwarf.
Sun
[edit | edit source]
A natural division of astronomical objects, between rocky objects, astronomical objects with solid surfaces, or solids and liquids predominately on the surface, and gas objects, astronomical objects with gases predominately detected and apparently constituting a surface, may be an informative approach toward stellar science. The Earth is an apparent rocky object that has a gaseous envelope. When viewed under certain conditions in radiation astronomy, the Earth appears as a gas object.
Depending primarily upon gas temperature, the presence of gas may be used to determine the composition of the gas object observed, at least the outer layer. Early spectroscopy[76] of the Sun using estimates of "the line intensities of several lines by eye [to derive] the abundances of ... elements ... [concluded] that the Sun [is] largely made of hydrogen."[77]
At right is an image from the GOES 14 Solar X-ray Imager during the most recent quiet period on or above the Sun. Except for X-ray emission that suggests a circular disc with some isolated X-ray sources at specific locations, the Sun is almost invisible. X-rays are primarily emitted from plasmas near 106 K.
IRC +10420
[edit | edit source]"IRC +10420 ... is a peculiar F8I+ hypergiant with a large far-infrared excess attributed to circumstellar dust"[78]. The Brα, Pfγ, and Brγ lines are in emission, "with Hα showing a double peaked profile".[78] A Teff range of 6000 K to 6500 K fit the spectral photometry.[78] A Teff range of ~6060 K to ~6300 K is a cyan star. "Based on the model fit, the contribution of the photosphere at the observed wavelengths is: ... Brγ (60%), Pfγ (8%), and Brα (6%), the rest of the continuum emission is due to thermally radiating dust."[78]
Beta Virginis
[edit | edit source]
Beta Virginis has a surface Teff = 6,132 ± 26.[79] According to SIMBAD, beta Virginis is a high proper-motion star with spectral type equal to F9V. Beta Virginis is also known as LHS 2465, GJ 449, HD 102870, and HR 4540. It is an X-ray source per 2E,RBS,RX,1RXS, and is a double star.
Alpha Centauri A
[edit | edit source]
Alpha Centauri A is the principal member, or primary, of the binary system, being slightly larger and more luminous [151.9% the luminosity of the Sun] than the Sun. It is a solar-like main sequence star with a similar yellowish color,[80] [surface temperature of 5790 K][81] whose stellar classification is spectral type G2 V.[82] From the determined mutual orbital parameters, Alpha Centauri A is about 10% more massive than the Sun, with a radius about 23% larger.[81] The projected rotational velocity ( v·sin i ) of this star is 2.7 ± 0.7 km·s−1, resulting in an estimated rotational period of 22 days,[83] which gives it a slightly faster rotational period than the Sun's 25 days.
Capella B
[edit | edit source]
Capella B has a surface temperature of approximately 5700 K, a radius of approximately 9 solar radii, a mass of approximately 2.6 solar masses, and a luminosity, again measured over all wavelengths, approximately 78 times that of the Sun.[84]
Capella B is spectral type G0III star and an X-ray source from the catalog [FS2003] 0255 by ROSAT. Its surface temperature has an uncertainty of 100 K. It is part of a binary star with Capella A a G8III. From SIMBAD, the orbital period is 104.0217 d with an eccentricity is 0.001 and inclination of 137.2° to the line of sight.
Although the binary star Capella is not an eclipsing binary, it is a RS Canum Venaticorum variable. These are close binary stars[85] having active chromospheres which can cause large stellar spots. These spots are believed to cause variations in their observed luminosity. Systems can exhibit variations on timescales of years due to variation in the spot surface coverage fraction, as well as periodic variations which are, in general, close to the orbital period of the binary system. Typical brightness fluctuation is around 0.2 magnitudes.
TW Hydrae
[edit | edit source]"TW Hydrae, a [K8V spectral type] star 176 light-years from Earth in the constellation Hydra ... which has about the same mass as the sun, is surrounded by a dense ring of gas and dust. ... Its circumstellar disk is estimated to between 3 million and 10 million years old, and most protoplanetary disks are thought to last only 2 million to 3 million years. ... [Using the] ESA's Herschel Space Telescope, which is sensitive to the required infrared wavelengths [to measure the amount of deuterium] ... The ratio of deuterium to hydrogen appears constant in Earth's region of space, which means ... measuring hydrogen deuteride [yields] how much regular molecular hydrogen is present. ... TW Hydrae's disk is at least 16,650 times the mass of the Earth. Considering the planets in the solar system may have arisen from a disk only as little as 3,300 times the mass of the Earth, the matter in TW Hydrae's disk would be ample to form a planetary system. "This ... seems to point towards different systems finding disparate pathways to making planets." "If there's no chance your project can fail, you're probably not doing very interesting science," ... Signs of hydrogen deuteride remain difficult to detect around distant stars"[86].
SY Persei
[edit | edit source]
The variability of BD +50 961 (SY Persei, an orange star, spectral type C6,4e) is confirmed.[35]
Astrophysics
[edit | edit source]Def. "any object forming on a dynamical timescale, by gravitational instability", is called a star.[87]
Sciences
[edit | edit source]Stellar classification is a classification of stars based on their spectral characteristics. The spectral class of a star is a designated class of a star describing the ionization of its chromosphere, what atomic excitations are most prominent in the light, giving an objective measure of the temperature in this chromosphere.>
"[T]he luminosity class [is] expressed by the Roman numbers I, II, III, IV and V, expressing the width of certain absorption lines in the star's spectrum.
Class | Temperature[88] K |
Conventional color | Apparent color[89][90][91] | Mass[88] (solar masses, Mʘ) |
Radius[88] (solar radii, Rʘ) |
Luminosity[88] (bolometric, Lʘ) |
Hydrogen lines |
Fraction of all main sequence stars[92] |
---|---|---|---|---|---|---|---|---|
O | ≥ 33,000 K | blue | blue | ≥ 16 | ≥ 6.6 | ≥ 30,000 | Weak | ~0.00003% |
B | 10,000–33,000 K | blue to blue white | blue white | 2.1–16 | 1.8–6.6 | 25–30,000 | Medium | 0.13% |
A | 7,500–10,000 K | white | white to blue white | 1.4–2.1 | 1.4–1.8 | 5–25 | Strong | 0.6% |
F | 6,000–7,500 K | yellowish white | white | 1.04–1.4 | 1.15–1.4 | 1.5–5 | Medium | 3% |
G | 5,200–6,000 K | yellow | yellowish white | 0.8–1.04 | 0.96–1.15 | 0.6–1.5 | Weak | 7.6% |
K | 3,700–5,200 K | orange | yellow orange | 0.45–0.8 | 0.7–0.96 | 0.08–0.6 | Very weak | 12.1% |
M | ≤ 3,700 K | red | orange red | ≤ 0.45 | ≤ 0.7 | ≤ 0.08 | Very weak | 76.45%. |
Hypotheses
[edit | edit source]- Any astronomical object with a luminescent photosphere is a star.
See also
[edit | edit source]References
[edit | edit source]- ↑ 1.0 1.1 SemperBlotto (3 April 2007). massive. San Francisco, California: Wikimedia Foundation, Inc. https://en.wiktionary.org/wiki/massive. Retrieved 2017-09-04.
- ↑ Iharoldz (21 May 2007). massive. San Francisco, California: Wikimedia Foundation, Inc. https://en.wiktionary.org/wiki/massive. Retrieved 2017-09-04.
- ↑ 3.0 3.1 3.2 3.3 Philip B. Gove, ed (1963). Webster's Seventh New Collegiate Dictionary. Springfield, Massachusetts: G. & C. Merriam Company. pp. 1221.
- ↑ 4.0 4.1 star. San Francisco, California: Wikimedia Foundation, Inc. June 22, 2012. http://en.wiktionary.org/wiki/star. Retrieved 2012-07-05.
- ↑ Robert Nemiroff & Jerry Bonnell (November 2, 2003). Astronomy Picture of the Day. Washington, DC USA: NASA. http://apod.nasa.gov/apod/ap031102.html. Retrieved 2014-08-31.
- ↑ K. Strassmeier (November 2, 2003). Giant starspot. NOAO. http://www.noao.edu/image_gallery/html/im0571.html. Retrieved 2014-08-31.
- ↑ 7.0 7.1 7.2 Caty Pilachowski (December 1999). "XX Marks the Spot". NOAO Newsletter (NOAO) (60). http://www.noao.edu/noao/noaonews/dec99/node2.html. Retrieved 2014-08-31.
- ↑ photosphere. San Francisco, California: Wikimedia Foundation, Inc. August 30, 2012. http://en.wiktionary.org/wiki/photosphere. Retrieved 2012-11-23.
- ↑ 9.0 9.1 Mike Guidry (1999-04-16). The Photosphere of the Sun. University of Tennessee. http://csep10.phys.utk.edu/astr162/lect/sun/photosphere.html. Retrieved 2006-10-12.
- ↑ M. L. Khodachenko and V. V. Zaitsev (March 01, 2002). "Formation of Intensive Magnetic Flux Tubes in a Converging Flow of Partially Ionized Solar Photospheric Plasma". Astrophysics and Space Science 279 (4): 389-410. doi:10.1023/A:1015162131331. http://link.springer.com/article/10.1023/A:1015162131331. Retrieved 2013-07-17.
- ↑ Space Weather Prediction Center (October 15, 2009). GLOSSARY OF SOLAR-TERRESTRIAL TERMS. NOAA / Space Weather Prediction Center. http://www.swpc.noaa.gov/info/Glossary.pdf. Retrieved 2012-04-18.
- ↑ press release 990610, K. G. Strassmeier, 1999-06-10, University of Vienna, "starspots vary on the same (short) time scales as Sunspots do", "HD 12545 had a warm spot (350 K above photospheric temperature; the white area in the picture)"
- ↑ Juna A. Kollmeier and Andrew Gould (July 20, 2007). "Where Are the Old-Population Hypervelocity Stars?". The Astrophysical Journal 664 (1): 343-8. doi:10.1086/518405. http://iopscience.iop.org/0004-637X/664/1/343. Retrieved 2012-03-05.
- ↑ Martin A. Barstow; L. Binette; Noah Brosch; F.Z. Cheng; Michel Dennefeld; A.I. G. de Castro; H. Haubold; K.A. van der Hucht et al. (February 26, 2003). J. Chris Blades. ed. The WSO: a world-class observatory for the ultraviolet, In: Future EUV/UV and Visible Space Astrophysics Missions and Instrumentation. 4854. The International Society for Optical Engineering. doi:10.1117/12.459779. http://proceedings.spiedigitallibrary.org/proceeding.aspx?articleid=876587. Retrieved 2013-07-15.
- ↑ Martin, Christopher; Seibert, M; Neill, JD; Schiminovich, D; Forster, K; Rich, RM; Welsh, BY; Madore, BF et al. (August 17, 2007). "A turbulent wake as a tracer of 30,000 years of Mira's mass loss history". Nature 448 (7155): 780–783. doi:10.1038/nature06003. PMID 17700694.
- ↑ Minkel, JR."Shooting Bullet Star Leaves Vast Ultraviolet Wake", "The Scientific American", August 15, 2007 Accessed August 21, 2007.
- ↑ Wareing, Christopher; Zijlstra, A. A.; O'Brien, T. J.; Seibert, M. (November 6, 2007). "It's a wonderful tail: the mass-loss history of Mira". Astrophysical Journal Letters 670 (2): L125–L129. doi:10.1086/524407. http://www.iop.org/EJ/article/1538-4357/670/2/L125/22252.html.
- ↑ W. Clavin (August 15, 2007). GALEX finds link between big and small stellar blasts. California Institute of Technology. http://web.archive.org/web/20070827103038/http://www.galex.caltech.edu/MEDIA/2007-04/images.html. Retrieved 2007-08-16.
- ↑ Christopher Wareing (December 13, 2008). "Wonderful Mira". Philosophical Transactions of the Royal Society A 366 (1884): 4429–40. doi:10.1098/rsta.2008.0167. PMID 18812301.
- ↑ M. Karovska (April 28, 2005). More Images of Mira. NASA/CXC/SAO/M. Karovska, et al.. http://chandra.harvard.edu/photo/2005/mira/more.html. Retrieved 2012-12-22.
- ↑ Castelaz, Michael W.; Luttermoser, Donald G. (1997). "Spectroscopy of Mira Variables at Different Phases.". The Astronomical Journal 114: 1584–1591. doi:10.1086/118589.
- ↑ Woodruff, H. C.; Eberhardt, M.; Driebe, T.; Hofmann, K.-H.; Ohnaka, K.; Richichi, A.; Schert, D.; Schöller, M.; Scholz, M.; Weigelt, G.; Wittkowski, M.; Wood, P. R. (2004). "Interferometric observations of the Mira star o Ceti with the VLTI/VINCI instrument in the near-infrared". Astronomy & Astrophysics 421 (2): 703–714. doi:10.1051/0004-6361:20035826. http://www.eso.org/~mwittkow/publications/conferences/SPIECWo5491199.pdf. Retrieved 2007-12-07.
- ↑ F. Winkler (December 21, 1999). RX J0822-4300 in Puppis A: Chandra Discovers Cosmic Cannonball. Cambridge, Massachusetts, USA: Harvard-Smithsonian Center for Astrophysics. http://chandra.harvard.edu/photo/2007/puppis/. Retrieved 2016-12-16.
- ↑ Pilar Ruiz-Lapuente, David J. Jeffery, Peter M. Challis, Alexei V. Filippenko, Robert P. Kirshner, Luis C. Ho, Brian P. Schmidt, Francisco Sanchez & Ramon Canal (21 October 1993). "A possible low-mass type Ia supernova". Nature 365 (6448): 728 - 730. doi:10.1038/365728a0. http://www.nature.com/nature/journal/v365/n6448/abs/365728a0.html. Retrieved 2017-05-02.
- ↑ Francis Halzen and Spencer R. Klein (May 2008). "Astronomy and astrophysics with neutrinos". Physics Today: 29-35. http://www.lbl.gov/today/2008/Jun/06-Fri/PTNuAstronomy.pdf. Retrieved 2012-07-28.
- ↑ A.K. Mann (1997). Shadow of a star: The neutrino story of Supernova 1987A. W. H. Freeman. p. 122. ISBN 0-7167-3097-9. http://www.whfreeman.com/GeneralReaders/book.asp?disc=TRAD&id_product=1058001008&@id_course=1058000240.
- ↑ KENNETH CHANG (April 26, 2005). Tiny, Plentiful and Really Hard to Catch, In: The New York Times. http://www.nytimes.com/2005/04/26/science/26neut.html?pagewanted=print&position=. Retrieved 2011-06-16.
- ↑ Nathan Smith, Jon A. Morse, Nicholas R. Collins, and Theodore R. Gull (August 2004). "The Purple Haze of eta Carinae: Binary-induced Variability?". The Astrophysical Journal 610 (2): L105-8. doi:10.1086/423341.
- ↑ 29.0 29.1 Nathan Smith (March 2005). "Doppler tomography of the Little Homunculus: High‐resolution spectra of [Fe II λ16 435 around Eta Carinae"]. Monthly Notices of the Royal Astronomical Society 357 (4): 1330-6. doi:10.1111/j.1365-2966.2005.08750.x. http://onlinelibrary.wiley.com/doi/10.1111/j.1365-2966.2005.08750.x/full. Retrieved 2012-02-27.
- ↑ A. Maeder (April 1983). "Evolution of chemical abundances in massive stars. I - OB stars, Hubble-Sandage variables and Wolf-Rayet stars - Changes at stellar surfaces and galactic enrichment by stellar winds. II - Abundance anomalies in Wolf-Rayet stars in relation with cosmic rays and 22/Ne in meteorites". Astronomy and Astrophysics 120 (1): 113-35. http://adsabs.harvard.edu/full/1983A%26A...120..113M. Retrieved 2013-09-19.
- ↑ Too Close for Comfort. NASA. August 7, 2003. http://hubblesite.org/newscenter/archive/releases/2003/21/. Retrieved 2010-01-21.
- ↑ 32.0 32.1 Preston, G. W.; Beers, T. C.; Shectman, S. A. (December 1993). "The Space Density and Kinematics of Metal-Poor Blue Main Sequence Stars Near the Solar Circle". Bulletin of the American Astronomical Society 25 (12): 1415.
- ↑ Helmut A. Abt (January 2009). "MK Classifications of Spectroscopic Binaries". The Astrophysical Journal Supplement 180 (1): 117–8. doi:10.1088/0067-0049/180/1/117.
- ↑ Tabetha S. Boyajian,; McAlister, Harold A.; van Belle, Gerard; Gies, Douglas R.; ten Brummelaar, Theo A.; von Braun, Kaspar; Farrington, Chris; Goldfinger, P. J. et al. (February 2012). "Stellar Diameters and Temperatures. I. Main-sequence A, F, and G Stars". The Astrophysical Journal 746 (1): 101. doi:10.1088/0004-637X/746/1/101.. See Table 10.
- ↑ 35.0 35.1 T. W. Backhouse (July 1899). "Confirmed or New Variable Stars". The Observatory 22 (281): 275-6. http://adsabs.harvard.edu//abs/1899Obs....22..276. Retrieved 2012-02-01.
- ↑ 36.0 36.1 Babak Tafreshi (May 28, 2012). The Southern Milky Way Above ALMA. Chajnantor plateau in Chile’s Atacama region. http://www.eso.org/public/images/potw1222a/. Retrieved 2014-03-01.
- ↑ orange sphere of the sun
- ↑ Noah Ashurov (2017). Chapter 1 – Introduction to infrared photography – Applications & Uses – Astronomy. LifePixel. https://www.lifepixel.com/infrared-photography-primer/ch1-applications-uses-astronomy. Retrieved 2017-05-01.
- ↑ P. F. L. Maxted, T. R. Marsh and R. C. North (2000). "KPD 1930+2752: a candidate Type Ia supernova progenitor". Monthly Notices of the Royal Astronomical Society 317 (3): L41-L44. doi:10.1046/j.1365-8711.2000.03856.x. http://mnras.oxfordjournals.org/content/317/3/L41.full.pdf+html. Retrieved 2016-12-19.
- ↑ 40.0 40.1 40.2 40.3 40.4 H. Olofsson (July 2, 2012). Red giant blows a bubble. Maryland USA: SpaceTelescope Organization. http://www.spacetelescope.org/images/potw1227a/. Retrieved 2013-12-24.
- ↑ 41.0 41.1 41.2 Jesse L. Greenstein (September 1974). "Photometry of a Pleiades candidate and composite white dwarfs". The Astronomical Journal 79 (9): 964-6. doi:10.1086/111638.
- ↑ Strasbourg astronomical Data Center (July 19, 2012). NAME VAN MAANEN STAR -- White Dwarf. Strasbourg, France: Centre de Données astronomiques de Strasbourg. http://simbad.u-strasbg.fr/simbad/sim-basic?Ident=EG+5&submit=SIMBAD+search. Retrieved 2012-07-18.
- ↑ The One Hundred Nearest Star Systems. RECONS. 2008-01-01. http://www.chara.gsu.edu/~thenry/RECONS/TOP100.posted.htm. Retrieved 2008-12-08.
- ↑ Holberg, J. B.; Oswalt, Terry D.; Sion, E. M. (May 2002). "A Determination of the Local Density of White Dwarf Stars". The Astrophysical Journal 571 (1): 512–518. doi:10.1086/339842.
- ↑ Gatewood, G.; Russell, J. (July 1974). "Astrometric determination of the gravitational redshift of van Maanen 2 (EG 5)". Astronomical Journal 79: 815–818. doi:10.1086/111613.
- ↑ Edward M. Sion; Holberg, J. B.; Oswalt, Terry D.; McCook, George P.; Wasatonic, Richard (December 2009). "The White Dwarfs Within 20 Parsecs of the Sun: Kinematics and Statistics". The Astronomical Journal 138 (6): 1681–1689. doi:10.1088/0004-6256/138/6/1681.
- ↑ 47.0 47.1 47.2 47.3 Donna Weaver and Ray Villard (17 December 2013). Hubble Watches Super Star Create Holiday Light Show. Washington, DC USA: NASA. http://www.nasa.gov/content/goddard/hubble-watches-super-star-create-holiday-light-show. Retrieved 2016-03-27.
- ↑ T. Lloyd Evans (15 March 1990). "Carbon stars with silicate dust shells - I. Carbon stars with enhanced 13C (J stars)". Monthly Notices of the Royal Astronomical Society 243: 336-348. http://adsabs.harvard.edu/full/1990MNRAS.243..336L. Retrieved 2017-04-29.
- ↑ 49.0 49.1 49.2 49.3 49.4 49.5 J. Davy Kirkpatrick, I. Neill Reid, James Liebert, Roc M. Cutri, Brant Nelson, Charles A. Beichman, Conard C. Dahn, David G. Monet, John E. Gizis, and Michael F. Skrutskie (10 July 1999). "Dwarfs Cooler than "M": The Definition of Spectral Type "L" Using Discoveries from the 2-Micron All-Sky Survey (2MASS)". The Astrophysical Journal 519 (2): 802-33. http://iopscience.iop.org/article/10.1086/307414/pdf. Retrieved 2017-04-29.
- ↑ S. E. Persson, D. C. Murphy, W. Krzeminski, M. Roth, and M. J. Reike (November 1998). "A New System of Faint Near-Infrared Standard Stars". The Astronomical Journal 116 (5): 2475. http://iopscience.iop.org/article/10.1086/300607/pdf. Retrieved 2017-04-29.
- ↑ Valentina D'Orazi, Raffaele Gratton, Sara Lucatello, Eugenio Carretta, Angela Bragaglia, and Anna F. Marino (5 August 2010). "Ba stars and other binaries in first and second generation stars in globular clusters". The Astrophysical Journal Letters 719 (2): L213-7. doi:10.1088/2041-8205/719/2/L213. http://iopscience.iop.org/article/10.1088/2041-8205/719/2/L213/meta. Retrieved 2017-04-29.
- ↑ Safl Bahcall, Bryan W. Lynn, and Stephen B. Selipsky (10 October 1990). "New models for neutron stars". The Astrophysical Journal 362 (10): 251-5. doi:10.1086/169261. http://adsabs.harvard.edu/full/1990ApJ...362..251B. Retrieved 2017-04-29.
- ↑ 53.0 53.1 53.2 53.3 53.4 53.5 53.6 Robert D. McClure (March 1997). "The R Stars: Carbon Stars of a Different Kind". Publications of the Astronomical Society of the Pacific 109: 256-263. http://iopscience.iop.org/article/10.1086/133882/pdf. Retrieved 2017-04-30.
- ↑ 54.0 54.1 54.2 54.3 54.4 54.5 John H. Bieging and William B. Latter (February 20, 1994). "A Millimeter-Wavelength Survey of S Stars for Mass Loss and Chemistry". The Astrophysical Journal 422 (2): 765-82. doi:10.1086/173769. http://adsabs.harvard.edu/full/1994ApJ...422..765B. Retrieved 2014-04-18.
- ↑ 55.0 55.1 55.2 55.3 B. Plez, V. Hill, R. Cayrel, M. Spite, B. Barbuy, T. C. Beers, P. Bonifacio, F. Primas and B. Nordström (11 December 2004). "Lead abundance in the uranium star CS 31082-001". Astronomy & Astrophysics 428 (1): L9–L12. doi:10.1051/0004-6361:200400094. http://www.aanda.org/articles/aa/pdf/2004/46/aagg211.pdf. Retrieved 2017-04-30.
- ↑ Anna Frebel, Norbert Christlieb, John E. Norris, Christopher Thom, Timothy C. Beers, and Jaehyon Rhee (10 May 2007). "Discovery of HE 1523–0901, a Strongly r-Process-enhanced Metal-poor Star with Detected Uranium". The Astrophysical Journal Letters 660: L117–L120. http://iopscience.iop.org/article/10.1086/518122/pdf. Retrieved 2017-04-30.
- ↑ 57.0 57.1 57.2 Johnson, H. L.; Morgan, W. W. (1953). "Fundamental stellar photometry for standards of spectral type on the revised system of the Yerkes spectral atlas". The Astrophysical Journal 117 (3): 313–352. doi:10.1086/145697.
- ↑ Hearnshaw, J. B. (1996). The Measurement of Starlight: Two Centuries of Astronomical Photometry. Cambridge University Press. p. 421. ISBN 9780521403931.
- ↑ Hearnshaw, J. B. (1996). The Measurement of Starlight: Two Centuries of Astronomical Photometry. Cambridge University Press. p. 425. ISBN 9780521403931.
- ↑ Iriarte, Braulio, Johnson, Harold L., Mitchell, Richard I., and Wisniewski, Wieslaw K. (1965), Five-Color Photometry of Bright Stars, Sky & Telescope, vol. 30, p. 21
- ↑ Landolt, Arlo U (2009). "Ubvriphotometric Standard Stars Around the Celestial Equator: Updates and Additions". The Astronomical Journal 137 (5): 4186. doi:10.1088/0004-6256/137/5/4186.
- ↑ Martin V. Zombeck (1990). "Calibration of MK spectral types". Handbook of Space Astronomy and Astrophysics (2nd ed.). Cambridge University Press. p. 105. ISBN 0-521-34787-4.
- ↑ David F. Gray (1992), The Inferred Color Index of the Sun, Publications of the Astronomical Society of the Pacific, vol. 104, no. 681, pp. 1035-1038 (November 1992)
- ↑ The Simbad Astronomical Database' Rigel page
- ↑ 65.0 65.1 James Binney; Merrifield M. Galactic Astronomy, Princeton University Press, 1998, ch. 2.3.2, pp. 53
- ↑ H. M. Johnson & D. E. Hogg (October 1965). "NGC 2359, NGC 6888, and Wolf-Rayet Stars". The Astrophysical Journal 142 (10): 1033-40. doi:10.1086/148373. http://adsabs.harvard.edu/full/1965ApJ...142.1033J. Retrieved 2017-04-30.
- ↑ B. E. Westerlund & A. W. Rodgers (August 1959). "Wolf-Rayet stars and planetary nebulae in the Large Magellanic Cloud". The Observatory 79 (08): 132-134. http://adsabs.harvard.edu/full/1959Obs....79..132W. Retrieved 2017-04-30.
- ↑ 68.0 68.1 Eric E. Mamajek, Warrick A. Lawson, and Eric D. Feigelson (10 May 1999). "The η Chamaeleontis Cluster: A Remarkable New Nearby Young Open Cluster". The Astrophysical Journal Letters 516 (2): L77–L80. doi:10.1086/312005. http://iopscience.iop.org/article/10.1086/312005/pdf. Retrieved 2017-05-01.
- ↑ Caroline V. Morley, Jonathan J. Fortney, Mark S. Marley, Channon Visscher, Didier Saumon, and S. K. Leggett (24 August 2012). "Neglected Clouds in T and Y Dwarf Atmospheres". The Astrophysical Journal 756 (2): 172. http://iopscience.iop.org/article/10.1088/0004-637X/756/2/172/meta. Retrieved 2017-04-30.
- ↑ D. A. Vandenberg, F. D. A. Hartwick, P. Dawson, & D. R. Alexander (15 March 1983). "Studies of late-type dwarfs. V - Theoretical models for lower main-sequence stars". The Astrophysical Journal 266 (03): 747-54. doi:10.1086/160821. http://adsabs.harvard.edu/full/1983ApJ...266..747V. Retrieved 2017-04-30.
- ↑ H. Nieuwenhuijzen & C. de Jager (January 2000). "Checking the yellow evolutionary void. Three evolutionary critical Hypergiants: HD 33579, HR 8752 & IRC +10420". Astronomy and Astrophysics 353 (1): 163-76.
- ↑ Martin Mutti (January 2009). Orion's Belt. Astronomical Image Data Archive. http://apod.nasa.gov/apod/ap090210.html. Retrieved 2016-12-16.
- ↑ Alpha Mic, Jim Kaler, Stars. Accessed on line September 4, 2008.
- ↑ J. Maíz Apellániz, A. Sota, J. I. Arias, R. H. Barbá, N. R. Walborn, S. Simón-Díaz, I. Negueruela, A. Marco, J. R. S. Leão, A. Herrero, R. C. Gamen, E. J. Alfaro (2016). "The Galactic O-Star Spectroscopic Survey (GOSSS). III. 142 Additional O-type Systems". The Astrophysical Journal Supplement Series 224: 4. doi:10.3847/0067-0049/224/1/4.
- ↑ 75.0 75.1 75.2 75.3 Yoichi Itoh (9 January 2004). Young Star's Companion Has Only Forty Times the Mass of Jupiter. SubaruTelescope.org. http://subarutelescope.org/Pressrelease/2005/02/24/index.html. Retrieved 2016-10-18.
- ↑ H. N. Russell (1929). The Astrophysical Journal 70: 11-82.
- ↑ Sarbani Basu and H. M. Antia (March 2008). "HelioseismologyandSolarAbundances". Physics Reports 457 (5-6): 217-83. doi:10.1016/j.physrep.2007.12.002.
- ↑ 78.0 78.1 78.2 78.3 René D. Oudmaijer, T.R. Geballe, L.B.F.M. Walters, and K.C. Sahu. "Discovery of near-infrared hydrogen line emission in the peculiar F8 hypergiant IRC +10420". Astronomy and Astrophysics 281 (1): L33-6.
- ↑ Tabetha S. Boyajian, Harold A. McAlister, Gerard van Belle, Douglas R. Gies, Theo A. ten Brummelaar, Kaspar von Braun, Chris Farrington, P. J. Goldfinger, David O'Brien, J. Robert Parks, Noel D. Richardson, Stephen Ridgway, Gail Schaefer, Laszlo Sturmann, Judit Sturmann, Yamina Touhami , Nils H. Turner, Russel White (February 2012). "Stellar Diameters and Temperatures. I. Main-sequence A, F, and G Stars". The Astrophysical Journal 746 (1): 101. doi:10.1088/0004-637X/746/1/101.. See Table 10.
- ↑ The Colour of Stars, In: Australia Telescope, Outreach and Education. Commonwealth Scientific and Industrial Research Organisation. December 21, 2004. http://outreach.atnf.csiro.au/education/senior/astrophysics/photometry_colour.html. Retrieved 2012-01-16.
- ↑ 81.0 81.1 Pierre Kervella and Frederic Thevenin (March 15, 2003). A Family Portrait of the Alpha Centauri System. ESO. http://web.archive.org/web/20080616125016/http://www.eso.org/public/outreach/press-rel/pr-2003/pr-05-03.html. Retrieved 2008-06-06.
- ↑ Research Consortium on Nearby Stars (2007-09-17). The One Hundred Nearest Star Systems. Georgia State University. http://web.archive.org/web/20071112173559/http://www.chara.gsu.edu/RECONS/TOP100.posted.htm. Retrieved 2007-11-06.
- ↑ Bazot, M. (2007). "Asteroseismology of α Centauri A. Evidence of rotational splitting". Astronomy and Astrophysics 470 (1): 295–302. doi:10.1051/0004-6361:20065694.
- ↑ C. A. Hummel (May 1994). "Very high precision orbit of Capella by long baseline interferometry". The Astronomical Journal 107 (5): 1859–67. doi:10.1086/116995.
- ↑ Berdyugina 2.4 RS CVn stars
- ↑ Charles Q. Choi (January 30, 2013). Star Not Too Old to Have Planets After All. Yahoo! News. http://news.yahoo.com/star-not-too-old-planets-180737690.html;_ylt=AkPIf6zH1eZaHpCJS.mN.pSHgsgF;_ylu=X3oDMTRmbHZzMHJkBG1pdANUb3BTdG9yeSBTY2llbmNlU0YgU3BhY2VBc3Ryb25vbXlTU0YEcGtnAzg4M2I4ZTIwLWFlMzQtMzk2ZS1hZWU0LTcxZmI4MDViZmFlZgRwb3MDMTQEc2VjA3RvcF9zdG9yeQR2ZXIDOGUyMjUwMjEtNmIwOC0xMWUyLWJmMmUtMTczMjNmNzgwOTAz;_ylg=X3oDMTI1MG9icjRhBGludGwDdXMEbGFuZwNlbi11cwRwc3RhaWQDBHBzdGNhdANzY2llbmNlfHNwYWNlLWFzdHJvbm9teQRwdANzZWN0aW9ucw--;_ylv=3. Retrieved 2013-01-31.
- ↑ Anthony Whitworth, Dimitri Stamatellos, Steffi Walch, Murat Kaplan, Simon Goodwin, David Hubber and Richard Parker (2009). R. de Grijs. ed. The formation of brown dwarfs, In: Star clusters: basic galactic building blocks, Proceedings IAU Symposium No. 266. International Astronomical Union. pp. 264-71. doi:10.1017/S174392130999113X. http://journals.cambridge.org/download.php?file=%2FIAU%2FIAU5_S266%2FS174392130999113Xa.pdf&code=410ba8317302679d01f7ea49a2a202a6. Retrieved 2011-10-30.
- ↑ 88.0 88.1 88.2 88.3 Tables VII, VIII, Empirical bolometric corrections for the main-sequence, G. M. H. J. Habets and J. R. W. Heinze, Astronomy and Astrophysics Supplement Series 46 (November 1981), pp. 193–237, bibcode=1981A&AS...46..193H. Luminosities are derived from Mbol figures, using Mbol(ʘ)=4.75.
- ↑ The Guinness book of astronomy facts & feats, Patrick Moore, 1992, 0-900424-76-1
- ↑ The Colour of Stars. Australia Telescope Outreach and Education. 2004-12-21. http://outreach.atnf.csiro.au/education/senior/astrophysics/photometry_colour.html. Retrieved 2007-09-26. — Explains the reason for the difference in color perception.
- ↑ What color are the stars?, Mitchell Charity. Accessed online March 19, 2008.
- ↑ Glenn LeDrew (February 2001). "The Real Starry Sky". Journal of the Royal Astronomical Society of Canada 95 (1 (whole No. 686, February 2001), pp. 32–33. Note: Table 2 has an error and so this article will use 824 as the assumed correct total of main-sequence stars). http://adsabs.harvard.edu/abs/2001JRASC..95...32L.
External links
[edit | edit source]- Bing Advanced search
- Google Books
- Google scholar Advanced Scholar Search
- International Astronomical Union
- JSTOR
- Lycos search
- NASA/IPAC Extragalactic Database - NED
- NASA's National Space Science Data Center
- Office of Scientific & Technical Information
- PubChem Public Chemical Database
- Questia - The Online Library of Books and Journals
- SAGE journals online
- The SAO/NASA Astrophysics Data System
- Scirus for scientific information only advanced search
- SDSS Quick Look tool: SkyServer
- SIMBAD Astronomical Database
- SIMBAD Web interface, Harvard alternate
- Spacecraft Query at NASA
- SpringerLink
- Taylor & Francis Online
- Universal coordinate converter
- Wiley Online Library Advanced Search
- Yahoo Advanced Web Search
{{Radiation astronomy resources}}