Portal:Radiation astronomy

Radiation astronomy is astronomy applied to the various extraterrestrial sources of radiation, especially at night. It is also conducted above the Earth's atmosphere and at locations away from the Earth, by satellites and space probes, as a part of explorational (or exploratory) radiation astronomy.
Seeing the Sun and feeling the warmth of its rays is probably a student's first encounter with an astronomical radiation source. This will happen from a very early age, but a first understanding of the concepts of radiation may occur at a secondary educational level.
Radiation is all around us on top of the Earth's crust, regolith, and soil, where we live. The study of radiation, including radiation astronomy, usually intensifies at the university undergraduate level.
And, generally, radiation becomes hazardous, when a student embarks on graduate study.
Cautionary speculation may be introduced unexpectedly to stimulate the imagination and open a small crack in a few doors that may appear closed at present. As such, this learning resource incorporates some state-of-the-art results from the scholarly literature.
The laboratories of radiation astronomy are limited to the radiation observatories themselves and the computers and other instruments (sometimes off site) used to analyze the results.
Radiation astronomy entities
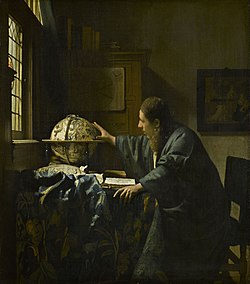
Radiation astronomy entities, radiation entities, are any astronomical persons or things that have separate and distinct existences in empirical, objective or conceptual reality.
Some of them, like the astronomers of today, or at any time in the past, are relatively known. But there are many entities that are far less known or understood, such as the observers of ancient times who suggested that deities occupied the sky or the heavens. Likewise, these alleged deities may be entities, or perhaps something a whole lot less.
Astronomical X-ray entities are often discriminated further into sources or objects when more information becomes available, including that from other radiation astronomies.
A researcher who turns on an X-ray generator to study the X-ray emissions in a laboratory so as to understand an apparent astronomical X-ray source is an astronomical X-ray entity. So is one who writes an article about such efforts or a computer simulation to possibly represent such a source.
"The X-ray luminosity of the dominant group [an entity] is an order of magnitude fainter than that of the X-ray jet."[1]
References
- ↑ A. Finoguenov, M.G. Watson, M. Tanaka, C.Simpson, M. Cirasuolo, J.S. Dunlop, J.A. Peacock, D. Farrah, M. Akiyama, Y. Ueda, V. Smolčič, G. Stewart, S. Rawlings, C.vanBreukelen, O. Almaini, L.Clewley, D.G. Bonfield, M.J. Jarvis, J.M. Barr, S. Foucaud, R.J. McLure, K. Sekiguchi, E. Egami (April 2010). "X-ray groups and clusters of galaxies in the Subaru-XMM Deep Field". Monthly Notices of the Royal Astronomical Society 403 (4): 2063-76. doi:10.1111/j.1365-2966.2010.16256.x. http://onlinelibrary.wiley.com/doi/10.1111/j.1365-2966.2010.16256.x/full. Retrieved 2011-12-09.
Theoretical astronomy

Theoretical astronomy at its simplest is the definition of terms to be applied to astronomical entities, sources, and objects.
Def. an "expanse of space that seems to be [overhead] like a dome"[1] is called a sky.
Computer simulations are usually used to represent astronomical phenomena.
Part of the fun of theory is extending the known to what may be known to see if knowing is really occurring, or is it something else.
The laboratories of astronomy are limited to the observatories themselves. The phenomena observed are located in the heavens, far beyond the reach, let alone control, of the astronomical observer.[2] “So how can one be sure that what one sees out there is subject to the same rules and disciplines of science that govern the local laboratory experiments of physics and chemistry?”[2] “The most incomprehensible thing about the universe is that it is comprehensible.” - Albert Einstein.[2]
References
- ↑ Philip B. Gove, ed (1963). Webster's Seventh New Collegiate Dictionary. Springfield, Massachusetts: G. & C. Merriam Company. pp. 1221. https://books.google.com/books?id=JtN_tgEACAAJ. Retrieved 2011-08-26.
- ↑ 2.0 2.1 2.2 Narlikar JV (1990). Pasachoff JM, Percy JR. ed. Curriculum for the Training of Astronomers ‘’In: The Teaching of astronomy. Cambridge, England: Cambridge University Press. http://adsabs.harvard.edu/abs/1990teas.conf....7N.
Backgrounds

In the figure at right, CUVOB stands for the cosmic ultraviolet and optical background.
The diffuse extragalactic background light (EBL) is all the accumulated radiation in the Universe due to star formation processes, plus a contribution from active galactic nuclei (AGNs). This radiation covers the wavelength range between ~ 0.1-1000 microns (these are the ultraviolet, optical, and infrared regions of the electromagnetic spectrum). The EBL is part of the diffuse extragalactic background radiation (DEBRA), which by definition covers the overall electromagnetic spectrum. After the cosmic microwave background, the EBL produces the second-most energetic diffuse background, thus being essential for understanding the full energy balance of the universe.
SN 185 was a supernova which appeared in the year 185, near the direction of Alpha Centauri, between the constellations Circinus and Centaurus, centered at Right ascension (RA) 14h 43m Declination (Dec) -62° 30', in Circinus. This "guest star" was observed by Chinese astronomers in the Book of Later Han.
On the right is a "combined image from the Chandra and XMM-Newton X-ray observatories of RCW 86 [in the constellation Circinus showing] the expanding ring of debris that was created after a massive star in the Milky Way collapsed onto itself and exploded. Both the Chandra and XMM images show low energy X-rays in red, medium energies in green and high energies in blue. The Chandra observations focused on the northeast (left-hand) side of RCW 86, and show that X-ray radiation is produced both by high-energy electrons accelerated in a magnetic field (blue) as well as heat from the blast itself (red)."[1]
"Properties of the shell in the Chandra image, along with the remnant's size and a basic understanding of how supernovas expand, were used to help determine the age of RCW 86. The new data revealed that RCW 86 was created by a star that exploded about 2,000 years ago. This age matches observations of a new bright star by Chinese astronomers in 185 A.D. (and possibly Romans as well) and may be the oldest known recordings of a supernova. Supernova explosions in galaxies like ours are rare, and none have been recorded in hundreds of years."[1]

The Crab Nebula is a remnant of an exploded star. This is the Crab Nebula in various energy bands, including a hard X-ray image from the HEFT data taken during its 2005 observation run. Each image is 6′ wide. Credit: NASA.
First gamma-ray source in Triangulum Australe
The first gamma-ray source in Triangulum Australe is unknown.
The field of gamma-ray astronomy is the result of observations and theories about gamma-ray sources detected in the sky above.
The first astronomical gamma-ray source discovered may have been the Sun.
But, gamma-rays from the Sun do not penetrate far enough into the Earth's atmosphere to be detected on the ground.
The early use of sounding rockets and balloons to carry detectors high enough may have detected gamma-rays from the Sun as early as the 1940s.
This is a lesson in map reading, coordinate matching, and searching. It is also a project in the history of gamma-ray astronomy looking for the first astronomical gamma-ray source discovered in the constellation of Triangulum Australe.
Nearly all the background you need to participate and learn by doing you've probably already been introduced to at a secondary level and perhaps even a primary education level.
Some of the material and information is at the college or university level, and as you progress in finding gamma-ray sources, you'll run into concepts and experimental tests that are an actual search.
Cyan astronomy quiz
Cyan astronomy is a lecture as part of the radiation astronomy department course on the principles of radiation astronomy.
You are free to take this quiz based on cyan astronomy at any time.
To improve your score, read and study the lecture, the links contained within, listed under See also, External links and in the {{principles of radiation astronomy}} template. This should give you adequate background to get 100 %.
As a "learning by doing" resource, this quiz helps you to assess your knowledge and understanding of the information, and it is a quiz you may take over and over as a learning resource to improve your knowledge, understanding, test-taking skills, and your score.
Suggestion: Have the lecture available in a separate window.
To master the information and use only your memory while taking the quiz, try rewriting the information from more familiar points of view, or be creative with association.
Enjoy learning by doing!
Electric orbits
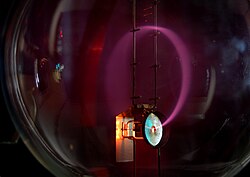
This laboratory is an activity for you to calculate an electric or magnetic orbit of an astronomical object. While it is part of the astronomy course principles of radiation astronomy, it is also independent.
Some suggested entities to consider are electric fields, magnetic fields, mass, charge, Euclidean space, Non-Euclidean space, or spacetime.
Okay, this is an astronomy orbits laboratory, specifically to try out electric/magnetic orbits and where possible compare them to those calculated using gravity.
Yes, this laboratory is structured.
I will provide an example of an electric/magnetic orbit. The rest is up to you.
Please put any questions you may have, and your laboratory results, you'd like evaluated, on the laboratory's discussion page.
Enjoy learning by doing!
Energy phantoms

Students start from specific situations of motion, determine how to calculate energy and convert units, then evaluate types of energy.
Def. a quantity that denotes the ability to do work and is measured in a unit dimensioned in mass × distance²/time² (ML²/T²) or the equivalent is called energy.
Def. a physical quantity that denotes ability to push, pull, twist or accelerate a body which is measured in a unit dimensioned in mass × distance/time² (ML/T²): SI: newton (N); CGS: dyne (dyn) is called force.
In astronomy we estimate distances and times when and where possible to obtain forces and energy.
The key values to determine in both force and energy are (L/T²) and (L²/T²). Force (F) x distance (L) = energy (E), L/T² x L = L²/T². Force and energy are related to distance and time using proportionality constants.
Every point mass attracts every single other point mass by a force pointing along the line intersecting both points. The force is proportional to the product of the two masses and inversely proportional to the square of the distance between them:[2] - ,
where:
- F is the force between the masses,
- G is the gravitational constant,
- m1 is the first mass,
- m2 is the second mass, and
- r is the distance between the centers of the masses.
The diagram shows two masses attracting one another. Credit: Dna-Dennis.
In the International System of Units (SI) units, F is measured in newtons (N), m1 and m2 in kilograms (kg), r in meters (m), and the constant G is approximately equal to 6.674×10−11
N m2 kg−2.[3]
Observationally, we may not know the origin of the force.
Coulomb's law states that the electrostatic force experienced by a charge, at position , in the vicinity of another charge, at position , in vacuum is equal to:
where is the electric constant and is the distance between the two charges.
Coulomb's constant is
where the constant is called the permittivity of free space in SI units of C2 m−2 N−1.
For reality, is the relative (dimensionless) permittivity of the substance in which the charges may exist.
The energy for this system is
where is the displacement.
References
- ↑ 1.0 1.1 J. Vink (15 June 2004). RCW 86: New Evidence Links Stellar Remains to Oldest Recorded Supernova. Cambridge, Massachusetts, USA: Harvard-Smithsonian Center for Astrophysics. http://chandra.harvard.edu/photo/2006/rcw86/. Retrieved 2016-02-12.
- ↑ - Proposition 75, Theorem 35: p.956 - I.Bernard Cohen and Anne Whitman, translators: Isaac Newton, The Principia: Mathematical Principles of Natural Philosophy. Preceded by A Guide to Newton's Principia, by I. Bernard Cohen. University of California Press 1999 ISBN 0-520-08816-6 ISBN 0-520-08817-4
- ↑ CODATA2006. http://www.physics.nist.gov/cgi-bin/cuu/Value?bg.

X-ray photo by Chandra X-ray Observatory of the Bullet Cluster. Exposure time was 140 hours. The scale is shown in megaparsecs. Redshift (z) = 0.3, meaning its light has wavelengths stretched by a factor of 1.3.
Fields associated with radiation astronomy include Astronomy, Astrogeology, Astrognosy, Astrohistory, Astrophysics, Atmospheric sciences, Charge ontology, Chemistry, Cosmogony, Fringe sciences, Geochemistry, Geochronology, Geology, Geomorphology, Geophysics, Geoseismology, Hydromorphology, Lofting technology, Mathematics, Measurements, Mining geology, Nuclear physics, Oceanography, Petrophysics, Radiation physics, Shielding, Spaceflights, Structural geology, Technology, Trigonometric-parallax astronomy, and X-ray trigonometric parallax
Other Wikiversity science-related portals: Anthropology - Archaeology - Astronomy - Biology - Biochemistry - Chemistry - Ecology - Fluid mechanics - Genetics - Hematology - Immunology - Life sciences - Materials science and engineering - Neurology - Organic chemistry - Particle physics - Quantum biology - Regional anatomy - Sciences - Technology
Wikiversity's sister projects
Wikiversity is hosted by the Wikimedia Foundation, a non-profit organization that also hosts a range of other multilingual and free-content projects: ![]() Wikipedia
Free-content encyclopedia ![]() Wikibooks
Free textbooks and manuals ![]() Commons
Shared media repository ![]() Incubator
Wikimedia incubator ![]() Wiktionary
Dictionary and thesaurus ![]() Wikiquote
Collection of quotations ![]() Wikinews
Free-content news ![]() Betawikiversity
Betawikiversity project ![]() Wikispecies
Directory of species ![]() Wikisource
Free-content library ![]() Wikivoyage
Open travel guide ![]() Phabricator
MediaWiki bug tracker ![]() Meta-Wiki
Wikimedia project coordination ![]() MediaWiki
Free software development ![]() Wikidata
Free knowledge base ![]() Wikimedia Labs
MediaWiki development Wikiversity is also available in other languages: |
Content by Subject
Arts ·
Humanities ·
Mathematics ·
Medicine ·
Science ·
Social Sciences ·
Technology
Content by Level
Pre-school Education ·
Primary Education ·
Secondary Education ·
Tertiary Education ·
Non-formal Education ·
Research