Object astronomy
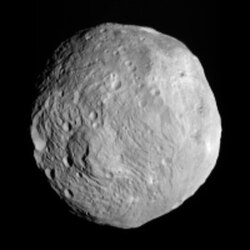
A natural object in any sky may be the subject of object astronomy.
Def. a natural object in the sky especially at night is called an astronomical object.
Planetary sciences
[edit | edit source]

The true-color image on the right shows North and South America as they would appear from space 35,000 km (22,000 miles) above the Earth.
The second image down on the right shows the Earth and Moon as seen by the Mars Global Surveyor on May 8, 2003, at 12:59:58 UTC.
Theoretical object astronomy
[edit | edit source]Here's a theoretical definition:
Def. astronomy of an object is called object astronomy.
Objects
[edit | edit source]The SIMBAD reference database "contains identifications, 'basic data', bibliography, and selected observational measurements for several million astronomical objects."[1]
"The specificity of the SIMBAD database is to organize the information per astronomical object".[1] "Building a reference database for ... all astronomical objects outside the Solar System – has been the first goal of the CDS".[1] "The only astronomical objects specifically excluded from SIMBAD are the Sun and Solar System bodies."[1]
Def. a natural object in the sky especially at night is called an astronomical object.
A celestial object is any astronomical object except the Earth.
Some objects seem to wander around in the night sky relative to many of the visual points of light. At least one occasionally is present in the early morning before sunrise as the Morning Star and after sunset as the Evening Star, the planet Venus. These wanderers and related objects are subjects for observation and some are meteors.
Others only make an appearance after decades, sometimes spectacularly.
High-velocity clouds
[edit | edit source]
Def. any cloud having a velocity "inconsistent with simple Galactic rotation models that generally fit the stars and gas in the Milky Way disk" is called a high-velocity cloud.[2]
"The leading edge of this cloud [shown in the image on the right] is already interacting with gas from our Galaxy."[3]
"The cloud, called Smith's Cloud, after the astronomer who discovered it in 1963, contains enough hydrogen to make a million stars like the Sun. Eleven thousand light-years long and 2,500 light-years wide, it is only 8,000 light-years from our Galaxy's disk. It is careening toward our Galaxy at more than 150 miles per second, aimed to strike the Milky Way's disk at an angle of about 45 degrees."[4]
"This is most likely a gas cloud left over from the formation of the Milky Way or gas stripped from a neighbor galaxy. When it hits, it could set off a tremendous burst of star formation. Many of those stars will be very massive, rushing through their lives quickly and exploding as supernovae. Over a few million years, it'll look like a celestial New Year's celebration, with huge firecrackers going off in that region of the Galaxy."[3]
"If you could see this cloud with your eyes, it would be a very impressive sight in the night sky. From tip to tail it would cover almost as much sky as the Orion constellation. But as far as we know it is made entirely of gas -- no one has found a single star in it."[3]
"Its shape, somewhat similar to that of a comet, indicates that it's already hitting gas in our Galaxy's outskirts. It is also feeling a tidal force from the gravity of the Milky Way and may be in the process of being torn apart. Our Galaxy will get a rain of gas from this cloud, then in about 20 to 40 million years, the cloud's core will smash into the Milky Way's plane."[3]
Hypervelocity stars
[edit | edit source]
"To date, all of the reported hypervelocity stars (HVSs), which are believed to be ejected from the Galactic center, are blue and therefore almost certainly young.”[5]
Def. a high-velocity star moving through space with an abnormally high velocity relative to the surrounding interstellar medium is called a runaway star.
Meteors
[edit | edit source]
A magnetic cloud is a transient event observed in the solar wind. It was defined in 1981 by Burlaga et al. 1981 as a region of enhanced magnetic field strength, smooth rotation of the magnetic field vector and low proton temperature [6]. Magnetic clouds are a possible manifestation of a Coronal Mass Ejection (CME). The association between CMEs and magnetic clouds was made by Burlaga et al. in 1982 when a magnetic cloud was observed by Helios-1 two days after being observed by SMM[7]. However, because observations near Earth are usually done by a single spacecraft, many CMEs are not seen as being associated with magnetic clouds. The typical structure observed for a fast CME by a satellite such as ACE is a fast-mode shock wave followed by a dense (and hot) sheath of plasma (the downstream region of the shock) and a magnetic cloud.
Other signatures of magnetic clouds are now used in addition to the one described above: among other, bidirectional superthermal electrons, unusual charge state or abundance of iron, helium, carbon and/or oxygen. The typical time for a magnetic cloud to move past a satellite at the L1 point is 1 day corresponding to a radius of 0.15 AU with a typical speed of 450 km s−1 and magnetic field strength of 20 nT.[8]
Def. a "massive burst of solar wind, other light isotope plasma, and magnetic fields rising above the solar corona or being released into space"[9] is called a coronal mass ejection (CME).
An explosive limb flare occurred above 30,000 km in the corona of the Sun.[10] "So the aftermath of the flare explosion, usually visible in disk pictures as extensive Hα brightening, but hidden from us in this case, was seen by the ionosphere as an intense flux of ionizing radiation from the coronal cloud created by the explosion."[10] "The November 20, 1960, event is very similar to that of February 10, 1956, which was observed at Sacramento Peak. A bright ball appears above the surface, grows in size and Hα brightness, and explodes upward and outward."[10] "The great breadth and intensity of the Hα emission from the suspended ball at 2013 U.T. testify to the large amount of energy stored there, as no corresponding macroscopic motion was observed until the explosion at 2023 U.T."[10] "[T]he great energy of the preflare cloud was released into the corona by the explosion of 2023 U.T., and Hα radiation disappeared by 2035 U.T."[10]
"On 16 June 1972, the Naval Research Laboratory's coronagraph aboard OSO-7 tracked a huge coronal cloud moving outward from the Sun."[11]
A coronal mass ejection (CME) is an ejected plasma consisting primarily of electrons and protons (in addition to small quantities of heavier elements such as helium, oxygen, and iron), plus the entraining coronal closed magnetic field regions. Evolution of these closed magnetic structures in response to various photospheric motions over different time scales (convection, differential rotation, meridional circulation) somehow leads to the CME.[12] Small-scale energetic signatures such as plasma heating (observed as compact soft X-ray brightening) may be indicative of impending CMEs.
The soft X-ray sigmoid (an S-shaped intensity of soft X-rays) is an observational manifestation of the connection between coronal structure and CME production.[12]
"Relating the sigmoids at X-ray (and other) wavelengths to magnetic structures and current systems in the solar atmosphere is the key to understanding their relationship to CMEs."[12]
Fireballs
[edit | edit source]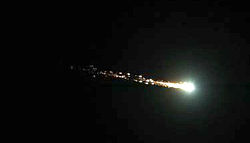
A fireball is a brighter-than-usual meteor. The International Astronomical Union defines a fireball as "a meteor brighter than any of the planets" (magnitude −4 or greater).[13] The International Meteor Organization (an amateur organization that studies meteors) has a more rigid definition. It defines a fireball as a meteor that would have a magnitude of −3 or brighter if seen at zenith. This definition corrects for the greater distance between an observer and a meteor near the horizon. For example, a meteor of magnitude −1 at 5 degrees above the horizon would be classified as a fireball because if the observer had been directly below the meteor it would have appeared as magnitude −6.[14]
For 2011 there are 4589 fireballs records at the American Meteor Society.[15]
At right is a cell phone camera image of the green fireball over San Mateo, California, that left meteorite fragments. "The asteroid entered at a speed of 14 km/s, typical but on the slow side of other meteorite falls for which orbits were determined. ... The orbit in space is also rather typical: perihelion distance close to Earth's orbit (q = 0.987 AU) and a low-inclination orbit (about 5 degrees). ... 2012, October 17 - At 7:44:29 pm PDT this evening, a bright fireball was seen in the San Francisco Bay Area."[16]
"At 66 kilometers (41 miles) per second, they appear as fast streaks, faster by a hair than their sisters, the Eta Aquarids of May. And like the Eta Aquarids, the brightest of family tend to leave long-lasting trains. Fireballs are possible three days after maximum."[17]
Lithometeors
[edit | edit source]
"This infrared image [at right] from NASA's Spitzer Space Telescope shows the Orion nebula, our closest massive star-making factory, 1,450 light-years from Earth. The nebula is close enough to appear to the naked eye as a fuzzy star in the sword of the popular hunter constellation."[18]
"The nebula itself is located on the lower half of the image, surrounded by a ring of dust. It formed in a cold cloud of gas and dust and contains about 1,000 young stars. These stars illuminate the cloud, creating the beautiful nebulosity, or swirls of material, seen here in infrared."[18]
"This image shows infrared light captured by Spitzer's infrared array camera. Light with wavelengths of 8 and 5.8 microns (red and orange) comes mainly from dust that has been heated by starlight. Light of 4.5 microns (green) shows hot gas and dust; and light of 3.6 microns (blue) is from starlight."[18]
Micrometeors
[edit | edit source]
A micrometeoroid is a tiny meteoroid; a small particle of rock in space, usually weighing less than a gram. A micrometeorite is such a particle that survives passage through the Earth's atmosphere and reaches the Earth's surface.
Micrometeoroids are extremely common in space. [These tiny] particles are a major contributor to space weathering processes. When they hit the surface of the Moon, or any airless body (Mercury, the asteroids, etc.), the resulting melting and vaporization causes darkening and other optical changes in the regolith.
Micrometeoroids have less stable orbits than meteoroids, due to their greater surface area to mass ratio.
Micrometeoroids pose a significant threat to space exploration.[19] Their velocities relative to a spacecraft in orbit average 10 kilometers per second (22,500 mph),[19] and resistance to micrometeoroid impact is a significant design challenge for spacecraft and space suit designers (See Thermal Micrometeoroid Garment). While the tiny sizes of most micrometeoroids limits the damage incurred, the high velocity impacts will constantly degrade the outer casing of spacecraft in a manner analogous to sandblasting. Long term exposure can threaten the functionality of spacecraft systems.
Cosmic rays
[edit | edit source]
"[C]oronal magnetic bottles, produced by flares, [may] serve as temporary traps for solar cosmic rays ... It is the expansion of these bottles at velocities of 300–500 km/s which allows fast azimuthal propagation of solar cosmic rays independent of energy. A coronagraph on Os 7 observed a coronal cloud which was associated with bifurcation of the underlying coronal structure."[20]
"A persistent problem of solar cosmic-ray research has been the lack of observations bearing on the timing and conditions in which protons that escape to the interplanetary medium are first accelerated in the corona."[21]
"A persistent problem of solar cosmic-ray research has been the lack of observations bearing on the timing and conditions in which protons that escape to the interplanetary medium are first accelerated in the corona."[21]
"For solar cosmic-rays, the apparent lack of proton acceleration in the corona seems justified, in contrast to the electrons, proton bremsstrahlung and gyrosynchrotron emission are negligible. This suggests a transit time anomaly, ΔTA, defined as follows:
- ΔTA = ΔTonset - 11 min,
where ΔTonset is the deduced Sun-Earth transit time for the first arriving relativistic protons and 11 min is the nominal transit time for a ~2 GeV proton traversing a 1.3 AU Archimedes spiral path."[21]
"The solar wind is a stream of charged particles ejected from the upper atmosphere of the Sun. It mostly consists of electrons and protons with energies usually between 1.5 and 10 keV. ΔTA may have values from "7-19 min for a small sample of well-connected ... cosmic-ray flares."[21] The transit time anomaly may be explained by a rise time associated with the ground-level events (GLEs). "The average GLE rise time ... for well-connected ... events ... defined to be the time from event onset to maximum as measured by the neutron monitor station showing the largest increase and whose asymptotic cone of acceptance ... includes the nominal direction of the Archimedean spiral path, is 21.3 min."[21]
"Data from an extensive air shower detector of ultrahigh-energy cosmic rays shows shadowing of the cosmic-ray flux by the Moon and the Sun with significance of 4.9 standard deviations. This is the first observation of such shadowing."[22]
"The ... solar proton flare on 20 April 1998 at W 90° and S 43° (9:38 UT) was measured by the GOES-9-satellite (Solar Geophysical Data 1998), as well as by other experiments on WIND ... and GEOTAIL. Protons were accelerated up to energies > 110 MeV and are therefore able to hit the surface of Mercury."[23] {{clear}]
Neutrons
[edit | edit source]"[G]alactic (X-ray pulsars, binary systems - black hole candidates) and extragalactic (blasars) objects [emit] gamma-rays [in periodical processes] and high-energy neutral radiation (gamma-rays, neutrons) [is emitted from] solar flares."[24]
Protons
[edit | edit source]"On 16 June 1972, the Naval Research Laboratory's coronagraph aboard OSO-7 tracked a huge coronal cloud moving outward from the Sun. ... This event is of great interest in its own right because it is the probable source of the solar proton event (Solar-Geophysical Data, NOAA Boulder) and shock-produced interplanetary scintillations (Dennison, 1972) which began early on 16 June."[11]
Electrons
[edit | edit source]"The density of the coronal cloud deduced in this case is about 2 x 1011 electrons per cubic centimeter."[25]
Positrons
[edit | edit source]"It is possible that the X-ray continuum is primary while the radio and optical emission are secondary for all BL Lac objects when the effect of relativistic beaming is considered. Pair production is a possible mechanism for producing X-ray emissions, while the optical and radio emission would be a consequence of this model (Zdziarski & Lightman 1985; Svensson 1986; Fabian et al. 1986). Barr & Mushotzky (1986) showed a significant correlation between the X-ray luminosity and timescale of X-ray variability for Seyfert galaxies and quasars and interpreted this as evidence that the emitting plasma is near the limit of being dominated by electron-positron pairs."[26]
Neutrinos
[edit | edit source]Since many neutrinos are assumed to come from stellar cores and supernovae, they are released at great temperature/energy. As neutrinos do not interact with matter electromagnetically, they are by definition dark matter.
Gamma rays
[edit | edit source]Over the entire celestial sphere, SIMBAD currently records 3253 gamma-ray objects. Some of these, like 4U 1705-44, are low-mass X-ray binaries (LMXBs). Some, like V779 Centauri, are high-mass X-ray binaries (HXMBs). Others are quasi-stellar objects (PKS 1326-697), supernova remnants (SNRs) like Messier 1 (M 1), and Seyfert galaxies like M 98. Some gamma-ray emitting objects have not been sufficiently resolved to determine what they are.
X-rays
[edit | edit source]Many astronomical objects when studied with visual astronomy may not appear to also be X-ray objects.
The SIMBAD database "contains identifications, 'basic data', bibliography, and selected observational measurements for several million astronomical objects."[1] Among these are some 209,612 astronomical X-ray objects. This information is found by going to the SIMBAD cite listed under 'External links', clicking on "Criteria query" and entering into the box "otype='X'", without the quotes, for an 'object count', and clicking on 'submit query'.
Ultraviolets
[edit | edit source]A PG 1159 star, often also called a pre-degenerate,[28] is a star with a hydrogen-deficient atmosphere which is in transition between being the central star of a planetary nebula and being a hot white dwarf. These stars are hot, with surface temperatures between 75,000 K and 200,000 K,[29] and are characterized by atmospheres with little hydrogen and absorption lines for helium, carbon and oxygen. The PG 1159 stars are named after their prototype, PG 1159-035. This star, found in the Palomar-Green survey of ultraviolet-excess stellar objects,[30] was the first PG 1159 star discovered.
Opticals
[edit | edit source]
"The Pleiades star cluster is one of the jewels of the northern sky. To the unaided eye it appears as an alluring group of stars in the constellation Taurus, while telescopic views reveal cluster stars surrounded by delicate blue wisps of dust-reflected starlight. To the X-ray telescopes on board the orbiting ROSAT observatory, the cluster also presents an impressive, but slightly altered, appearance. This false color image [at right] was produced from ROSAT observations by translating different X-ray energy bands to visual colors - the lowest energies are shown in red, medium in green, and highest energies in blue. (The green boxes mark the position of the seven brightest visual stars.) The Pleiades stars seen in X-rays have extremely hot, tenuous outer atmospheres called coronas and the range of colors corresponds to different coronal temperatures."[31]
Visuals
[edit | edit source]
"NASA gave people a front row seat to today's total solar eclipse, thanks to a partnership with the University of California at Berkeley and the Exploratorium. A streaming webcast brought the eclipse -- visible along a path from South America to Africa to Asia -- to schools and museums and computer desktops worldwide. ... The sun's corona, or outer atmosphere, is visible during totality -- when the sun is totally obscured by the [M]oon's shadow."[32]
Violets
[edit | edit source]Calcium has a line occurring in the solar corona at 408.63 nm of Ca XIII.[33]
Iron has a line occurring in the solar corona in the violet at 398.69 nm of Fe XI.[33]
Nickel has three emission lines occurring in the solar corona at 380.08 nm of Ni XIII and 423.14 nm and 431.1 of Ni XII.[33]
Blues
[edit | edit source]The Edinburgh-Cape Blue Object Survey is an astronomical catalog included in the list of astronomical catalogues. These catalogs are lists or tabulations of astronomical objects. They are grouped together because they share a common type, morphology, origin, means of detection, or method of discovery.
Cyans
[edit | edit source]
At right is a visual astronomy image of IC 1396 using narrowband filters: sulfur is red, oxygen blue and hydrogen in green. The image was captured using a Ritchey Chretien 12.5" with a 2180 mm focal length.
Greens
[edit | edit source]
"During its mission, the Galileo spacecraft returned a number of images of Earth's only natural satellite. Galileo surveyed the moon on Dec. 7, 1992, on its way to explore the Jupiter system in 1995-1997."[34]
"This color mosaic was assembled from 18 images taken by Galileo's imaging system through a green filter. On the upperleft is the dark, lava-filled Mare Imbrium, Mare Serenitatis (middle left), Mare Tranquillitatis (lower left), and Mare Crisium, the dark circular feature toward the bottom of the mosaic. Also visible in this view are the dark lava plains of the Marginis and Smythii Basins at the lower right. The Humboldtianum Basin, a 400-mile impact structure partly filled with dark volcanic deposits, is seen at the center of the image."[34]
Yellows
[edit | edit source]"An abundance analysis of the yellow symbiotic system AG Draconis reveals it to be a metal-poor K-giant ([Fe/H]=-1.3) which is enriched in the heavy s-process elements. ... the other yellow symbiotic stars are probably low-metallicity objects as well."[35]
The image on the right captures the color of the Sun at its photosphere peak in the yellow-green.
Oranges
[edit | edit source]
"Spring has finally come to the northern hemisphere of Uranus. The newest images, both the visible-wavelength ones described here and those taken a few days earlier with the Near Infrared and Multi-Object Spectrometer (NICMOS) by Erich Karkoschka (University of Arizona), show a planet with banded structure and detectable clouds."[36]
"The "red" image (on the right) is taken at 6,190 Angstroms, and is sensitive to absorption by methane molecules in the planet's atmosphere. The banded structure of Uranus is evident, and the small cloud near the northern limb is now visible."[36]
Reds
[edit | edit source]"The nature of extremely red objects (EROs) remains an open question in understanding the faint galaxy population at z > 1."[37]
Infrareds
[edit | edit source]

"NASA's new Spitzer Space Telescope has captured [the image right] of an unusual comet that experiences frequent outbursts, which produce abrupt changes in brightness. Periodic comet Schwassmann-Wachmann I (P/SW-1) has a nearly circular orbit just outside that of Jupiter, with an orbital period of 14.9 years. It is thought that the outbursts arise from the build-up of internal gas pressure as the heat of the Sun slowly evaporates frozen carbon dioxide and carbon monoxide beneath the blackened crust of the comet nucleus. When the internal pressure exceeds the strength of the overlying crust, a rupture occurs, and a burst of gas and dust fragments is ejected into space at speeds of 450 miles per hour (200 meters per second)."[38]
"This 24-micron image of P/SW-1 was obtained with Spitzer's multiband imaging photometer. The image shows thermal infrared emission from the dusty coma and tail of the comet. The nucleus of the comet is about 18 miles (30 kilometers) in diameter and is too small to be resolved by Spitzer. The micron-sized dust grains in the coma and tail stream out away from the Sun. The dust and gas comprising the comet's nucleus is part of the same primordial materials from which the Sun and planets were formed billions of years ago. The complex carbon-rich molecules they contain may have provided some of the raw materials from which life originated on Earth."[38]
"Schwassmann-Wachmann 1 is thought to be a member of a relatively new class of objects called "Centaurs," of which 45 objects are known. These are small icy bodies with orbits between those of Jupiter and Neptune. Astronomers believe that Centaurs are recent escapees from the Kuiper Belt, a zone of small bodies orbiting in a cloud at the distant reaches of the solar system."[38]
"Two asteroids, 1996 GM36 (left) and 5238 Naozane (right) were serendipitously captured in the comet image. Because they are closer to us than the comet and have faster orbital velocities, they appear to move relative to the comet and background stars, thereby producing a slight elongated appearance. The Spitzer data have allowed astronomers to use thermal measurements, which reduce the uncertainties of visible-light albedo (reflectivity) measurements, to determine their size. With radii of 1.4 and 3.0 kilometers, these are the smallest main-belt asteroids yet measured by infrared means."[38]
In the second image pair, "NASA's Spitzer Space Telescope captured the picture on the left of comet Holmes in March 2008, five months after the comet suddenly erupted and brightened a millionfold overnight. The contrast of the picture has been enhanced on the right to show the anatomy of the comet."[39]
"Every six years, comet 17P/Holmes speeds away from Jupiter and heads inward toward the sun, traveling the same route typically without incident. However, twice in the last 116 years, in November 1892 and October 2007, comet Holmes mysteriously exploded as it approached the asteroid belt. Astronomers still do not know the cause of these eruptions."[39]
"Spitzer's infrared picture at left reveals fine dust particles that make up the outer shell, or coma, of the comet. The nucleus of the comet is within the bright whitish spot in the center, while the yellow area shows solid particles that were blown from the comet in the explosion. The comet is headed away from the sun, which lies beyond the right-hand side of the picture."[39]
"The contrast-enhanced picture on the right shows the comet's outer shell, and strange filaments, or streamers, of dust. The streamers and shell are a yet another mystery surrounding comet Holmes. Scientists had initially suspected that the streamers were small dust particles ejected from fragments of the nucleus, or from hyperactive jets on the nucleus, during the October 2007 explosion. If so, both the streamers and the shell should have shifted their orientation as the comet followed its orbit around the sun. Radiation pressure from the sun should have swept the material back and away from it. But pictures of comet Holmes taken by Spitzer over time show the streamers and shell in the same configuration, and not pointing away from the sun. The observations have left astronomers stumped."[39]
"The horizontal line seen in the contrast-enhanced picture is a trail of debris that travels along with the comet in its orbit."[39]
"The Spitzer picture was taken with the spacecraft's multiband imaging photometer at an infrared wavelength of 24 microns."[39]
Submillimeters
[edit | edit source]"On the whole the emission strength is low in the submillimeter for astronomical objects."[40]
Microwaves
[edit | edit source]"Passive microwave satellite observations [in the image on the right] indicate that surface melt occurred during one or more days over a broad sector of West Antarctica (termed Ross sector hereafter) in January 2016, with up to 15 melt days over parts of the eastern Ross Ice Shelf and Siple Coast."[41]
"January 2016 was one of the three largest melt events in the Ross sector since 1978 (second behind 1991–92 for [melt index (MI) (melt area weighted by duration of the melting)] MI, and a virtual tie for first with January 2005 for melt extent)."[41]
"The satellite observations were corroborated on the ground by a number of automatic weather stations (AWSs) that recorded near-surface temperatures near or above 0 °C for several consecutive days during 10–21 January [...]. The onset of the melt event on 10 January was accompanied by an abrupt temperature increase at WAIS Divide and Byrd, in central West Antarctica. The temperature time series from these two sites highlight roughly two phases: Phase 1 (10–14 January), during which the temperatures were at their warmest; and Phase 2 (15–21 January), during which the temperatures gradually decreased towards their pre-event levels. The transition from Phase 1 to Phase 2 is characterized by a shift of the melt pattern towards the Transantarctic Mountains apparent in the AWS temperature time series and in the sequence of daily melt maps [...]."[41]
"[R]ain was witnessed by a field party on 12 January (Dr Huw Horgan, Victoria University of Wellington, personal communication)."[41]
Radars
[edit | edit source]
On the right is a composite of hourly radar images. These wind gusts averaged ~75 mph over about 450 miles. This is referred to as the Derecho event.
Radios
[edit | edit source]At right is a radio image of the Sun at 4.6 GHz. "The brightest discrete radio source is the Sun, but it is much less dominant than it is in visible light. The radio sky is always dark, even when the Sun is up, because atmospheric dust doesn't scatter radio waves, whose wavelengths are much longer than the dust particles."[42]
"The quiet Sun at 4.6 GHz imaged by the [Very Large Array] VLA with a resolution of 12 arcsec, or about 8400 km on the surface of the Sun. The brightest features (red) in this false-color image have brightness temperatures ~ 106 K and coincide with sunspots. The green features are cooler and show where the Sun's atmosphere is very dense. At this frequency the radio-emitting surface of the Sun has an average temperature of 3 x 104 K, and the dark blue features are cooler yet. The blue slash crossing the bottom of the disk is a feature called a filament channel, where the Sun's atmosphere is very thin: it marks the boundary of the South Pole of the Sun. The radio Sun is somewhat bigger than the optical Sun: the solar limb (the edge of the disk) in this image is about 20000 km above the optical limb."[42]
Superluminals
[edit | edit source]"Tracking the features within small-scale jets has revealed interesting complications. The paths are not always radial to the nucleus, usually taken as the source with the flattest spectrum in ambiguous cases. This is based on the general principle that synchrotron spectra are flattened at lower frequencies by self-absorption, so the densest plasma will have a flat or inverted spectrum. An interesting case is 3C 345 [at right], in which emission features repeatedly appear off the core and follow fairly consistent nonradial paths. [...] a new component appears in late 1985, brightens, and moves outward changing its relative position angle in the process".[43]
Plasma objects
[edit | edit source]In physics and chemistry, plasma is a state of matter similar to gas in which a certain portion of the particles are ionized.
The image on the right shows the coronal clouds around and near to the Sun.
Gaseous objects
[edit | edit source]
In orange astronomy, Titan is a gaseous object.
Titan like Venus is another gas dwarf when viewed in visible light. Much as with Venus prior to the Space Age, the dense, opaque atmosphere prevented understanding of Titan's surface until new information accumulated with the arrival of the Cassini–Huygens mission in 2004, including the discovery of liquid hydrocarbon lakes in the polar regions.
The atmosphere of Titan is largely composed of nitrogen; minor components lead to the formation of methane and ethane clouds and nitrogen-rich organic smog.
Titan has a mean radius of 2576 ± 2 km.[44]
Liquid objects
[edit | edit source]
A division of astronomical objects between rocky objects, liquid objects, gaseous objects (including gas giants and stars), and plasma objects may be natural and informative.
The astronomy of liquid objects may be called liquid-object astronomy.
The image at right is a detailed, photo-like view of Earth based largely on observations from the Moderate Resolution Imaging Spectroradiometer (MODIS) on NASA’s Terra satellite.
Rocky objects
[edit | edit source]
A division of astronomical objects between rocky objects, liquid objects, gaseous objects (including gas giants and stars), and plasma objects may be natural and informative.
This division allows moons like Io to be viewed as rocky objects like Earth, rather than as a satellite around Jupiter.
The astronomy of such objects may be called rocky-object astronomy.
Sun
[edit | edit source]
Depending primarily upon gas temperature, the presence of gas may be used to determine the composition of the gas object observed, at least the outer layer. Early spectroscopy[45] of the Sun using estimates of "the line intensities of several lines by eye [to derive] the abundances of ... elements ... [concluded] that the Sun [is] largely made of hydrogen."[46]
Mercury
[edit | edit source]
"[H]igh-resolution spectral measurements of Mercury show emission in sodium D lines (Potter and Morgan 1985a). This suggests a substantial sodium population in Mercury's atmosphere ... possibly due to photo-sputtering of the planetary surface"[47]. At least in emission yellow astronomy, Mercury is a dwarf gaseous object.
In "1991, the Arecibo radio telescope in Puerto Rico detected unusually radar-bright patches at Mercury's poles, spots that reflected radio waves in the way one would expect if there were water ice [denoted in the image at right by yellow areas]. Many of these patches corresponded to the location of large impact craters mapped by the Mariner 10 spacecraft in the 1970s."[48]
"Images from the spacecraft's Mercury Dual Imaging System taken in 2011 and earlier this year confirmed that radar-bright features at Mercury's north and south poles are within shadowed regions on Mercury's surface, findings that are consistent with the water-ice hypothesis."[48]
Venus
[edit | edit source]
The right image is the first X-ray image ever made of Venus. It "shows a half crescent due to the relative orientation of the Sun, Earth and Venus. The X-rays from Venus are produced by fluorescent radiation from oxygen and other atoms in the atmosphere between 120 and 140 kilometers above the surface of the planet."[49] The fluorescent source of the X-rays places Venus in the gas dwarf category even though a rocky object lies some 120 km beneath this layer.
Earth
[edit | edit source]The Earth's atmosphere is a relatively bright source of gamma rays produced in interactions of ordinary cosmic ray protons with air atoms. In gamma-ray and X-ray astronomy, Earth is a dwarf gaseous object.
Mars
[edit | edit source]
From the point of view of X-ray astronomy, Mars is a dwarf gaseous object.
At right is an X-ray image of Mars. X-radiation from the Sun excites oxygen atoms in the Martian upper atmosphere, about 120 km above its surface, to emit X-ray fluorescence. A faint X-ray halo that extends out to 7,000 km above the surface of Mars has also been found.[50] The Chandra X-ray Observatory image on the right is the first look at X-rays from Mars.
In X-ray astronomy, Mars is a gas dwarf.
"Four hydrogen (H2) lines have been detected in a spectrum of Mars observed with the Far Ultraviolet Spectroscopic Explorer. ... The line intensities correspond to [an] H2 abundance ... above 140 kilometers on Mars. ... Analysis of [deuterium] fractionation among a few reservoirs of ice, water vapor, and molecular hydrogen on Mars implies that a global ocean more than 30 meters deep was lost since the end of hydrodynamic escape. Only 4% of the initially accreted water remained on the planet at the end of hydrodynamic escape, and initially Mars could have had even more water (as a proportion of mass) than Earth."[51]
Vesta
[edit | edit source]
In the full image of Vesta at right, the rocky-object appears to have suffered from meteor damage.
Vesta, minor-planet designation 4 Vesta, is one of the largest asteroids in the Solar System. It lost some 1% of its mass less than a billion years ago in a collision that left an enormous crater occupying much of its southern hemisphere. Debris from this event has fallen to Earth as howardite–eucrite–diogenite (HED) meteorites, a rich source of information about the asteroid.[52][53]
Asteroids
[edit | edit source]"The advantages of radar in planetary astronomy result from (1) the observer's control of all the attributes of the coherent signal used to illuminate the target, especially the wave form's time/frequency modulation and polarization; (2) the ability of radar to resolve objects spatially via measurements of the distribution of echo power in time delay and Doppler frequency; (3) the pronounced degree to which delay-Doppler measurements constrain orbits and spin vectors; and (4) centimeter-to-meter wavelengths, which easily penetrate optically opaque planetary clouds and cometary comae, permit investigation of near-surface macrostructure and bulk density, and are sensitive to high concentrations of metal or, in certain situations, ice."[54]
Jupiter
[edit | edit source]
Jupiter is a larger gaseous object in X-ray and ultraviolet astronomy.
Jupiter is seen as a gaseous object in orange, red and infrared astronomy.
Io
[edit | edit source]
Green astronomy indicates Io is a dwarf gaseous object.
At right is an "eerie view of Jupiter's moon Io in eclipse ... acquired by NASA's Galileo spacecraft while the moon was in Jupiter's shadow. Gases above the satellite's surface produced a ghostly glow that could be seen at visible wavelengths (red, green, and violet). The vivid colors, caused by collisions between Io's atmospheric gases and energetic charged particles trapped in Jupiter's magnetic field, had not previously been observed. The green and red emissions are probably produced by mechanisms similar to those in Earth's polar regions that produce the aurora, or northern and southern lights. Bright blue glows mark the sites of dense plumes of volcanic vapor, and may be places where Io is electrically connected to Jupiter."[55]
Saturn
[edit | edit source]
Saturn is a larger gaseous object from X-ray and ultraviolet astronomy.
Saturn is a gas giant.
Uranus
[edit | edit source]
In optical and visual astronomy, Uranus is a gaseous object in orbit around the Sun.
Uranus's atmosphere has a primary composition of hydrogen and helium and contains more "ices" such as water, ammonia, and methane, along with traces of hydrocarbons.[56]
The helium molar fraction, i.e. the number of helium atoms per molecule of gas, is 0.15 ± 0.03[57] in the upper troposphere, which corresponds to a mass fraction 0.26 ± 0.05.[56][58]
The third most abundant constituent of the Uranian atmosphere is methane (CH4).[56] Methane molecules account for 2.3% of the atmosphere by molar fraction below the methane cloud deck at the pressure level of 1.3 bar (130 kPa); this represents about 20 to 30 times the carbon abundance found in the Sun.[56][59][60] The mixing ratio is much lower in the upper atmosphere owing to its extremely low temperature, which lowers the saturation level and causes excess methane to freeze out.[61] The abundances of less volatile compounds such as ammonia, water and hydrogen sulfide in the deep atmosphere are poorly known. Along with methane, trace amounts of various hydrocarbons are found in the stratosphere of Uranus, which are thought to be produced from methane by photolysis induced by the solar ultraviolet (UV) radiation.[62] They include ethane (C2H6), acetylene (C2H2), methylacetylene (CH3C2H), and diacetylene (C2HC2H).[61][63][64]
Spectroscopy has also uncovered traces of water vapor, carbon monoxide and carbon dioxide in the upper atmosphere, which can only originate from an external source such as infalling dust and comets.[63][64][65]
Neptune
[edit | edit source]
In optical and visual astronomy, Neptune is a gaseous-object in orbit around the Sun.
Neptune's atmosphere is composed primarily of hydrogen and helium, along with traces of hydrocarbons and possibly nitrogen, contains a higher proportion of "ices" such as water, ammonia, and methane. Traces of methane in the outermost regions in part account for the planet's blue appearance.[66]
At high altitudes, Neptune's atmosphere is 80% hydrogen and 19% helium.[67] A trace amount of methane is also present. Prominent absorption bands of methane occur at wavelengths above 600 nm, in the red and infrared portion of the spectrum. As with Uranus, this absorption of red light by the atmospheric methane is part of what gives Neptune its blue hue,[68] although Neptune's vivid azure differs from Uranus's milder cyan. Since Neptune's atmospheric methane content is similar to that of Uranus, some unknown atmospheric constituent is thought to contribute to Neptune's colour.[66]
Comets
[edit | edit source]The image at the right is an optical astronomy image of the comet 67P/Churyumov-Gerasimenko. Rosetta's OSIRIS narrow-angle camera made the image on 3 August 2014 from a distance of 285 km. The image resolution is 5.3 metres/pixel.
The left image is rotated 90° from the right. The location of the right image is the front view of the left side just out of view in the left image. The object rotates by the right hand rule from the left image to the right.
Note that due to the evaporation of volatiles, the surface of the rocky object appears pitted or cratered.
The 1910 Halley's comet approach, which came into naked-eye view around 10 April[69] and came to perihelion on 20 April,[69] was notable for several reasons: it was the first approach of which photographs exist, and the first for which spectroscopic data were obtained.[70] Furthermore, the comet made a relatively close approach of 0.15AU,[69] making it a spectacular sight. Indeed, on 19 May, the Earth actually passed through the tail of the comet.[71][72] One of the substances discovered in the tail by spectroscopic analysis was the toxic gas cyanogen,[73] which led astronomer Camille Flammarion to claim that, when Earth passed through the tail, the gas "would impregnate the atmosphere and possibly snuff out all life on the planet."[74] His pronouncement led to panicked buying of gas masks and quack "anti-comet pills" and "anti-comet umbrellas" by the public.[75] In reality, as other astronomers were quick to point out, the gas is so diffuse that the world suffered no ill effects from the passage through the tail.[74]
"It is quite possible that [faint streamers preceding the main tail and lying nearly in the prolonged radius vector] may have touched the Earth, probably between May 19.0 and May 19.5, [1910,] but the Earth must have passed considerably to the south of the main portion of the tail [of Halley's comet]."[76]
Kuiper belts
[edit | edit source]
The Kuiper belt is a region of the solar system extending from the orbit of Neptune (at 30 AU to approximately 60 AU from the Sun.[77] It consists mainly of small bodies.
"[B]roadband optical photometry of Centaurs and Kuiper Belt objects from the Keck 10 m, the University of Hawaii 2.2 m, and the Cerro Tololo InterAmerican (CTIO) 1.5 m telescopes [shows] a wide dispersion in the optical colors of the objects, indicating nonuniform surface properties. The color dispersion [may] be understood in the context of the expected steady reddening due to bombardment by the ubiquitous flux of cosmic rays."[78]
In the image at right, objects in the main part of the Kuiper belt are coloured green, while scattered objects are coloured orange. The four outer planets are blue. Neptune's few known trojans are yellow, while Jupiter's are pink. The scattered objects between Jupiter's orbit and the Kuiper belt are known as centaurs. The scale is in astronomical units. The pronounced gap at the bottom is due to difficulties in detection against the background of the plane of the Milky Way.
Legend:
- Red = The Sun
- Aquamarine = Giant Planet
- Lime Green = Kuiper belt object
- Orange = Scattered disc object or Centaur
- Magenta = Trojan of Jupiter
- Yellow = Trojan of Neptune
Axes list distances in AU, projected onto the ecliptic, with ecliptic longitude zero being to the right, along the "x" axis).
Positions are accurate for January 1st, 2000 (J2000 epoch) with some caveats:
For planets, positions should be exact.
For minor bodies, positions are extrapolated from other epochs assuming purely Keplerian motion. As all data is from an epoch between 1993 and 2007, this should be a reasonable approximation.
Data from the Minor Planet Center[79] or Murray and Dermott[80] as needed.
Radial "spokes" of higher density in this image, or gaps in particular directions are due to observational bias (i.e. where objects were searched for), rather than any real physical structure. The pronounced gap at the bottom is due to obscuration by the band of the Milky Way.
Stars
[edit | edit source]"Some of these stars are intended spectroscopic targets: there are standards of various kinds, stars targeted for stellar science and/or Galactic structure science, and objects that are assigned spectroscopic fibers due to their unusual location in 5-color space."[81]
Star spots
[edit | edit source]
"Our Sun itself frequently has sunspots, relatively cool dark magnetic depressions that move across its surface. HD 12545, however, exhibits the largest starspots yet observed. Doppler imaging - the use of slight changes in color caused by the rotation of the star - was used to create this false-color image. The vertical bar on the right gives a temperature scale in kelvins. This giant, binary, RS CVn star, also known as XX Trianguli, is visible with binoculars in the constellation of Triangulum. The starspot is thought to be caused by large magnetic fields that inhibit hot matter from flowing to the surface."[82]
"A giant starspot was revealed on the K0 giant star XX Triangulum, also known as HD12545, using Doppler imaging on the Kitt Peak National Observatory's 0.9m Coude Feed telescope. This picture shows a series of views around the star".[83]
"To observe spots on the surfaces of other stars, astronomers need to "resolve" the stellar disk. This cannot be done directly with the largest telescopes even planned, but Doppler imaging can be used to obtain a map of inhomogeneities on a star's surface. The principle is similar to medical tomography, but instead of a scanner rotating around a fixed object, a rotating star is observed with a fixed telescope. A cool starspot rotating into view at the preceding limb of the star causes a blue-shifted asymmetry in each spectral line profile. This asymmetry moves into the line center at the time of meridian passage, and turns into a red-shifted asymmetry after meridian passage. The asymmetry fades away when the spot disappears at the receding limb. The higher the latitude of the spot, the shorter will be its visible path across the projected disk of the star, or the spot may even be circumpolar if the stellar rotation axis is inclined. All this information is hidden in the variation of the spectral line profiles and is reconstructed by mathematical inversion to create a true picture of the stellar surface. For a successful application, the telescope needs to "see" the entire stellar surface during at least one stellar rotation."[84]
"XX Triangulum is an active K0 giant binary star, approximately 10 times larger and twice as massive as the Sun. Its rotation period is 24 days, so that 24 consecutive (clear) nights of telescope time with an excellent high-resolution optical spectrograph are needed to obtain a good Doppler image. Because starspots vary on the same (short) time scales as Sunspots do (they are stable for about one stellar rotation), all the observations must be made on one rotation cycle. NSF's Kitt Peak National Observatory is one of the few facilities worldwide that offers this capability with the 0.9-m coude feed telescope."[84]
"During the observations, XX Triangulum had its brightest magnitude since the discovery of its light variability in 1985 and showed the largest photometric amplitude so far (0.63 magnitudes in V). The large photometric amplitude was explained when the Doppler-imaging inversion algorithm also recovered a not-quite-as-large equatorial warm spot (350 K above the photospheric temperature) in the hemisphere opposite the dark spot. Strassmeier speculates that the warm spot harbors the same magnetic field as the cool spot but opposite polarity. Surprisingly, the fact that the star was brighter at a time of high spot activity is in agreement with the solar analogy despite the "unsolar" dimension of the gigantic spot."[84]
Blue stragglers
[edit | edit source]
Blue stragglers (BSS) are main sequence stars in open or globular clusters that are more luminous and bluer than stars at the main sequence turn-off point for the cluster. Standard theories of stellar evolution hold that the position of a star on the Hertzsprung–Russell diagram should be determined almost entirely by the initial mass of the star and its age. In a cluster, stars all formed at approximately the same time, and thus in an H–R diagram for a cluster, all stars should lie along a clearly defined curve set by the age of the cluster, with the positions of individual stars on that curve determined solely by their initial mass. With masses two to three times that of the rest of the main sequence cluster stars, blue stragglers seem to be exceptions to this rule.[86] The resolution of this problem is likely related to interactions between two or more stars in the dense confines of the clusters in which blue stragglers are found.
Recurrent novas
[edit | edit source]
RS Ophiuchi (RS Oph) is a recurrent nova system approximately 5,000 light-years away in the constellation Ophiuchus. In its quiet phase it has an apparent magnitude of about 12.5. It erupted in 1898, 1933, 1958, 1967, 1985, and 2006 and reached about magnitude 5 on average. The recurrent nova is produced by a white dwarf star and a red giant circling about each other in a close orbit. About every 20 years, enough material from the red giant builds up on the surface of the white dwarf to produce a thermonuclear explosion. The white dwarf orbits close to the red giant, with an accretion disc concentrating the overflowing atmosphere of the red giant onto the white dwarf. If the white dwarf accretes enough mass to reach the Chandrasekhar limit, about 1.4 solar mass, it may explode as a Type Ia supernova.
Stellar groupings
[edit | edit source]

Def. two "stars that appear to be one when seen with the naked eye"[87] is called a double star.
Def. a "star that appears as a double due to an optical illusion; in reality, the stars may be far apart from each other"[88] is called an optical double.
Def. two and only two stars orbiting around their apparent barycenter is called a binary star.
Def. a "binary star whose components can be visually resolved"[89] is called a visual binary.
Def. a "group of gravitationally bound stars"[90] is called a star cluster.
Def. "a more or less irregular star cluster containing tens to thousands of stars"[91] is called an open cluster.
Def. "a spherical star cluster containing thousands to millions of stars"[92] is called a globular cluster.
Def. a "large group of many stars spread over a very many light-years of space [a region of greater than average stellar density]"[93] is called a star cloud.
Def. a group of stars moving together through space is called a stellar association.
Def. an association of stars stretched out along its orbit of a galaxy is called a stellar stream.
Def. a stellar association drifting through the galaxy as a somewhat coherent assemblage is called a moving group.
Def. any "of the collections of many millions of stars ... existing as independent and coherent systems"[94] is called a galaxy.
Def. any "galaxy, considerably smaller than the Milky Way, that has only several billions of stars"[95] is called a dwarf galaxy.
Def. a "small group of stars that forms a visible pattern but is not an official constellation"[96] is called an asterism.
Def. any "of the [89] officially recognized regions of the sky, including all stars"[97] is called a constellation.
Galaxies
[edit | edit source]
The galaxy, called NGC 5010, is in a period of transition. The aging galaxy is moving on from life as a spiral galaxy, like our Milky Way, to an older, less defined type called an elliptical galaxy. In this in-between phase, astronomers refer to NGC 5010 as a lenticular galaxy, which has features of both spirals and ellipticals.
"Schweizer [1978] defined five observational characteristics that unambiguously identified NGC 7252 as a late-stage merger ... One of these new characteristics is the blue portion of the optical spectrum of the main body."[98]
Active galactic nuclei
[edit | edit source]There is "a correlation between the arrival directions of cosmic rays with energy above 6 x 1019 electron volts and the positions of active galactic nuclei (AGN) lying within ~75 megaparsecs."[99]
Some low energy cosmic rays originate or are associated with solar flares. Even these cosmic rays have too high an energy to originate from the solar photosphere. The coronal cloud in close proximity to the Sun may be a source or create them as it bombards the chromosphere from above.
"In particular we recognize a first trace of Vela, brightest gamma and radio galactic source, and smeared sources along Galactic Plane and Center [as a source of ultra high energy cosmic rays (UHECR)]."[100]
"The main correlated map is the 408 MHz one. The first astronomical source that seem to correlate is the main multiplet along CenA. This AGN source, the nearest extragalactic one, sits in the same direction of a far Centaurus Cluster (part of the Super-Galactic Plane). The blurring by random galactic magnetic field might spread the nearest AGN event along the same Super-Galactic Plane, explaining the AUGER group miss-understanding [3]."[100]
Craters
[edit | edit source]
Def. a hemispherical pit a basinlike opening or mouth about which a cone is often built up any large roughly circular depression or hole is called a crater.
The image at right shows a chain of 13 craters (Enki Catena) on Ganymede measuring 161.3 km in length. "The Enki craters formed across the sharp boundary between areas of bright terrain and dark terrain, delimited by a thin trough running diagonally across the center of this image. The ejecta deposit surrounding the craters appears very bright on the bright terrain. Even though all the craters formed nearly simultaneously, it is difficult to discern any ejecta deposit on the dark terrain.
Hypotheses
[edit | edit source]- Objects/Astronomy and Astronomy/Objects are the same things: astronomical objects.
See also
[edit | edit source]References
[edit | edit source]- ↑ 1.0 1.1 1.2 1.3 1.4 Marc Wenger; François Ochsenbein; Daniel Egret; Pascal Dubois; François Bonnarel; Suzanne Borde; Françoise Genova; Gérard Jasniewicz et al. (April 2000). "The SIMBAD astronomical database The CDS Reference Database for Astronomical Objects". Astronomy and Astrophysics 143 (4): 9-22. doi:10.1051/aas:2000332. http://arxiv.org/pdf/astro-ph/0002110. Retrieved 2011-10-31.
- ↑ Hugo van Woerden; Ulrich J. Schwarz; Reynier F. Peletier; Bart P. Wakker; Peter M. W. Kalberla (8 July 1999). "A confirmed location in the Galactic halo for the high-velocity cloud 'chain A'". Nature 400 (6740): 138-41. http://www.nature.com/nature/journal/v400/n6740/abs/400138a0.html. Retrieved 2015-10-03.
- ↑ 3.0 3.1 3.2 3.3 Felix J. Lockman (11 January 2008). Massive Gas Cloud Speeding Toward Collision With Milky Way. National Radio Astronomy Observatory (NRAO). http://www.nrao.edu/pr/2008/smithscloud/. Retrieved 2015-10-03.
- ↑ Dave Finley (11 January 2008). Massive Gas Cloud Speeding Toward Collision With Milky Way. National Radio Astronomy Observatory (NRAO). http://www.nrao.edu/pr/2008/smithscloud/. Retrieved 2015-10-03.
- ↑ Juna A. Kollmeier; Andrew Gould (July 20, 2007). "Where Are the Old-Population Hypervelocity Stars?". The Astrophysical Journal 664 (1): 343-8. doi:10.1086/518405. http://iopscience.iop.org/0004-637X/664/1/343. Retrieved 2012-03-05.
- ↑ Burlaga, L. F., E. Sittler, F. Mariani, and R. Schwenn, "Magnetic loop behind an interplanetary shock: Voyager, Helios and IMP-8 observations" in "Journal of Geophysical Research", 86, 6673, 1981
- ↑ Burlaga, L. F. et al., "A magnetic cloud and a coronal mass ejection" in "Geophysical Research Letter"s, 9, 1317-1320, 1982
- ↑ Lepping, R. P. et al. "Magnetic field structure of interplanetary magnetic clouds at 1 AU" in "Journal of Geophysical Research", 95, 11957-11965, 1990.
- ↑ coronal mass ejection. San Francisco, California: Wikimedia Foundation, Inc. 21 June 2013. http://en.wiktionary.org/wiki/coronal_mass_ejection. Retrieved 2013-07-07.
- ↑ 10.0 10.1 10.2 10.3 10.4 Harold Zirin (October 1964). "The Limb Flare of November 20, 1960: a Coronal Phenomenon". Astrophysical Journal 140 (10): 1216-35. doi:10.1086/148019.
- ↑ 11.0 11.1 Martin Koomen; Russell Howard; Richard Hansen; Shirley Hansen (February 1974). "The coronal transient of 16 June 1972". Solar Physics 34 (2): 447-52. doi:10.1007/BF00153680. http://link.springer.com/article/10.1007/BF00153680. Retrieved 2013-07-10.
- ↑ 12.0 12.1 12.2 Gopalswamy N; Mikic Z; Maia D; Alexander D; Cremades H; Kaufmann P; Tripathi D; Wang YM. "The pre-CME Sun". Space Science Reviews 2006 123 (1–3): 303. doi:10.1007/s11214-006-9020-2.
- ↑ MeteorObs Explanations and Definitions (states IAU definition of a fireball). Meteorobs.org. 1999-07-09. http://www.meteorobs.org/maillist/msg13871.html. Retrieved 2011-09-16.
- ↑ International Meteor Organization - Fireball Observations. Imo.net. 2004-10-12. http://www.imo.net/fireball. Retrieved 2011-09-16.
- ↑ Fireball Report: 4589 records found between 2011-01-01 and 2011-12-31. American Meteor Society. http://www.amsmeteors.org/fireball2/public.php?start_date=2011-01-01&end_date=2011-12-31&submit=Find+Reports. Retrieved 2012-04-24.
- ↑ Petrus M. Jenniskens (October 20, 2012). 2012, October 20 - FIRST METEORITE FOUND!. San Francisco, California: NASA Ames Research Center. http://cams.seti.org/. Retrieved 2012-10-22.
- ↑ David Levy; Stephen Edberg. Observe: Meteors. Astronomical League.
- ↑ 18.0 18.1 18.2 Tom Megeath; Rob Gutermuth; Joe Hora; Lori Allen; Kevin Flaherty; John Stauffer; Lee Hartmann; James Muzerolle et al. (August 14, 2006). Orion's Inner Beauty. Pasadena, California USA: NASA/JPL/California Institute of Technology. http://www.spitzer.caltech.edu/images/1643-ssc2006-16a-Orion-s-Inner-Beauty. Retrieved 2014-03-06.
- ↑ 19.0 19.1 Micrometeoroids and Orbital Debris (MMOD) - NASA - White Sands Test Facility, Las Cruces, NM [1]
- ↑ K. H. Schatten; D. J. Mullan (December 1, 1977). "Fast azimuthal transport of solar cosmic rays via a coronal magnetic bottle". Journal of Geophysical Research 82 (35): 5609-20. doi:10.1029/JA082i035p05609. http://www.agu.org/pubs/crossref/1977/JA082i035p05609.shtml. Retrieved 2013-07-07.
- ↑ 21.0 21.1 21.2 21.3 21.4 E. W. Cliver; S. W. Kahler; M. A. Shea; D. F. Smart (1 September 1982). "Injection onsets of ~2 GeV protons, ~1 MeV electrons, and ~100 keV electrons in solar cosmic ray flares". The Astrophysical Journal 260 (9): 362-70.
- ↑ D.E. Alexandreas; R.C. Allen; D. Berley; S.D. Biller; R.L. Burman; D.R. Cady; C.Y. Chang; B.L. Dingus et al. (March 1, 1991). "Observation of shadowing of ultrahigh-energy cosmic rays by the moon and the sun". Physical Review, D (Particles Fields) 43 (5): 1735-8. http://www.osti.gov/energycitations/product.biblio.jsp?osti_id=6399949. Retrieved 2012-08-22.
- ↑ E. Kirsch; U.A. Mall; B. Wilken; G. Gloeckler; A.B. Galvin; K. Cierpka (August 17, 1999). D. Kieda. ed. Detection of Pickup- and Sputter Ions by Experiment SMS on the WIND-S/C After a Mercury Conjunction, In: Proceedings of the 26th International Cosmic Ray Conference. Salt Lake City, Utah, USA: International Union of Pure and Applied Physics (IUPAP). pp. 212-5. Bibcode: 1999ICRC....6..212K.
- ↑ Kudryavtsev M. I.; Pankov V. M.; Bogomolov A. V.; Bogomolov V. V.; Denisov Yu. I.; Kolesov G. Ya.; Logachev Yu. I.; Svertilov S. I. (1995). N. Iucci and E. Lamanna. ed. The MIR-SPECTR Gamma-Astronomy Experiment. 3. Rome, Italy: International Union of Pure and Applied Physics. pp. 567-70. http://adsabs.harvard.edu/abs/1995ICRC....3..567K. Retrieved 2013-11-01.
- ↑ H. Zinn (1965). C. C. Chang and S. S. Huang. ed. Solar Flares and Concurrent Phenomena in the Solar Atmosphere, In: Proceedings of the Plasma Space Science Symposium. 3. Netherlands: Springer. pp. 38-51. doi:10.1007/978-94-011-7542-5_5. ISBN 978-94-011-7544-9. http://link.springer.com/chapter/10.1007/978-94-011-7542-5_5. Retrieved 2013-07-07.
- ↑ G. Z. Xie; B. F. Liu; J. C. Wang (November 20, 1995). "A Signature of Relativistic Electron-Positron Beams in BL Lacertae Objects". The Astrophysical Journal 454 (11): 50-4. doi:10.1086/176463. http://adsabs.harvard.edu/full/1995ApJ...454...50X. Retrieved 2013-08-13.
- ↑ Ronald L. Gilliland; Andrea K. Dupree (May 1996). "First Image of the Surface of a Star with the Hubble Space Telescope". Astrophysical Journal Letters 463 (1): L29-32. doi:10.1086/310043. http://iopscience.iop.org/1538-4357/463/1/L29/pdf/5023.pdf. Retrieved 1 August 2010.
- ↑ Jaschek & Jaschek: CARBON C
- ↑ Observational constraints on the evolutionary connection between PG 1159 stars and DO white dwarfs, S. D. Huegelmeyer, S. Dreizler, K. Werner, J. Krzesinski, A. Nitta, and S. J. Kleinman. arXiv:astro-ph/0610746.
- ↑ The Palomar-Green catalog of ultraviolet-excess stellar objects, R. F. Green, M. Schmidt, and J. Liebert, Astrophysical Journal Supplement 61 (June 1986), pp. 305–352. Centre de Données astronomiques de Strasbourg (CDS) ID II/207.
- ↑ Robert Nemiroff; Jerry Bonnell (August 28, 1999). X-Ray Pleiades. Greenbelt, Maryland USA: NASA/GSFC. http://apod.nasa.gov/apod/ap990828.html. Retrieved 2013-07-07.
- ↑ Lynn Jenner (November 30, 2007). "NASA Shares Solar Eclipse With the World". Washington, DC USA: NASA. Retrieved 2013-07-08.
- ↑ 33.0 33.1 33.2 P. Swings (July 1943). "Edlén's Identification of the Coronal Lines with Forbidden Lines of Fe X, XI, XIII, XIV, XV; Ni XII, XIII, XV, XVI; Ca XII, XIII, XV; a X, XIV". The Astrophysical Journal 98 (07): 116-28. doi:10.1086/144550.
- ↑ 34.0 34.1 Yvette Smith (December 8, 2009). Earth's Moon. NASA. http://www.nasa.gov/multimedia/imagegallery/image_feature_1538.html. Retrieved 2012-07-22.
- ↑ V. V. Smith; K. Cunha; A. jorissen; H. M. J. Boffin (December 1996). "Abundances in the symbiotic star AG Draconis: the barium-symbiotic connection". Astronomy and Astrophysics 315 (11): 179-93. http://adsabs.harvard.edu/abs/1996A%26A...315..179S. Retrieved 2013-09-17.
- ↑ 36.0 36.1 Heidi Hammel (July 31, 1997). Clouds on Uranus. Boston, Massachusetts: NASA. http://solarsystem.nasa.gov/multimedia/display.cfm?Category=Planets&IM_ID=2104. Retrieved 2012-07-21.
- ↑ Michael C. Liu; Arjun Dey; James R. Graham; Charles C. Steidel; Kurt Adelberger (1999). Andrew J. Bunker and Wil J. M. van Breugel. ed. Extremely Red Galaxies in the Field of QSO 1213-0017: A Galaxy Concentration at z = 1.31, In: The Hy-Redshift Universe: Galaxy Formation and Evolution at High Redshift. 193. Berkeley, California USA: American Society of Physics. pp. 344-7. ISBN 1-58381-019-6. Bibcode: 1999ASPC..193..344L. http://adsabs.harvard.edu/full/1999ASPC..193..344L. Retrieved 2013-07-30.
- ↑ 38.0 38.1 38.2 38.3 Dale Cruikshank (December 18, 2003). Comet Schwassmann-Wachmann 1. Pasadena, California, USA: NASA, JPL, California Institute of Technology. http://www.spitzer.caltech.edu/images/1115-ssc2003-06j-Comet-Schwassmann-Wachmann-1. Retrieved 2012-11-26.
- ↑ 39.0 39.1 39.2 39.3 39.4 39.5 W. Reach (October 10, 2008). Anatomy of a Busted Comet. Pasadena, California, USA: NASA, JPL, California Institute of Technology. http://www.spitzer.caltech.edu/images/2742-ssc2008-18a-Anatomy-of-a-Busted-Comet. Retrieved 2012-11-26.
- ↑ T.G. Phillips; J. Keene (November 1992). "Submillimeter astronomy [heterodyne spectroscopy"]. Proceedings of the IEEE 80 (11): 1662-78. doi:10.1109/5.175248. http://ieeexplore.ieee.org/xpls/abs_all.jsp?arnumber=175248. Retrieved 2013-10-21.
- ↑ 41.0 41.1 41.2 41.3 Julien P. Nicolas; Andrew M. Vogelmann; Ryan C. Scott; Aaron B. Wilson; Maria P. Cadeddu; David H. Bromwich; Johannes Verlinde; Dan Lubin et al. (2017). "January 2016 extensive summer melt in West Antarctica favoured by strong El Niño". Nature 8: 15799. doi:10.1038/ncomms15799. https://www.nature.com/articles/ncomms15799. Retrieved 2017-06-17.
- ↑ 42.0 42.1 S.G. Djorgovski. A Tour of the Radio Universe. National Radio Astronomy Observatory. http://www.cv.nrao.edu/course/astr534/Tour.html. Retrieved 2014-03-16.
- ↑ Bill Keel (October 2003). Jets, Superluminal Motion, and Gamma-Ray Bursts. Tucson, Arizona USA: University of Arizona. http://www.astr.ua.edu/keel/galaxies/jets.html. Retrieved 2014-03-19.
- ↑ R. A. Jacobson; P.G. Anreasian; J.J. Bordi; K.E. Criddle; R. Ionasescu; J.B. Jones; R. A. MacKenzie; M.C. Meek et al. (December 2006). "The Gravity Field of the Saturnian System from Satellite Observations and Spacecraft Tracking Data". The Astronomical Journal 132 (6): 2520-6. doi:10.1086/508812. http://iopscience.iop.org/1538-3881/132/6/2520/fulltext. Retrieved 2012-07-08.
- ↑ H. N. Russell (1929). The Astrophysical Journal 70: 11-82.
- ↑ Sarbani Basu; H. M. Antia (March 2008). "HelioseismologyandSolarAbundances". Physics Reports 457 (5-6): 217-83. doi:10.1016/j.physrep.2007.12.002.
- ↑ C. T. Russell; D. N. Baker; J. A. Slavin (January 1, 1988). Faith Vilas. ed. The Magnetosphere of Mercury, In: Mercury. Tucson, Arizona, United States of America: University of Arizona Press. pp. 514-61. ISBN 0816510857. Bibcode: 1988merc.book..514R. http://www-ssc.igpp.ucla.edu/personnel/russell/papers/magMercury.pdf. Retrieved 2012-08-23.
- ↑ 48.0 48.1 Brian Dunbar (November 29, 2012). MESSENGER Finds New Evidence for Water Ice at Mercury's Poles. http://www.nasa.gov/mission_pages/messenger/media/PressConf20121129.html. Retrieved 2013-10-24.
- ↑ File:Venus xray 420.jpg. San Francisco, California: Wikimedia Foundation, Inc. October 28, 2010. http://commons.wikimedia.org/wiki/File:Venus_xray_420.jpg. Retrieved 2012-08-08.
- ↑ K. Dennerl (November 2002). "Discovery of X-rays from Mars with Chandra". Astronomy & Astrophysics 394 (11): 1119-28. doi:10.1051/0004-6361:20021116.
- ↑ Vladimir A. Krasnopolsky; Paul D. Feldman (November 2001). "Detection of Molecular Hydrogen in the Atmosphere of Mars". Science 294 (5548): 1914-7. doi:10.1126/science.1065569. http://www.sciencemag.org/content/294/5548/1914.short. Retrieved 2013-10-05.
- ↑ Kelley, M. S.. "Quantified mineralogical evidence for a common origin of 1929 Kollaa with 4 Vesta and the HED meteorites". Icarus 165 (1): 215 2003. doi:10.1016/S0019-1035(03)00149-0.
- ↑ Vesta. NASA/JPL. 12 July 2011. http://web.archive.org/web/20110629153841/http://www.nasa.gov/mission_pages/dawn/ceresvesta/index.html. Retrieved 30 July 2011.
- ↑ Steven J. Ostro (October 1993). "Planetary radar astronomy". Reviews of Modern Physics 65 (4): 1235-79. doi:10.1103/RevModPhys.65.1235. http://rmp.aps.org/abstract/RMP/v65/i4/p1235_1. Retrieved 2012-02-09.
- ↑ Sue Lavoie (October 13, 1998). PIA01637: Io's Aurorae. Pasadena, California: NASA and the Jet Propulsion Laboratory, California Institute of Technology. http://photojournal.jpl.nasa.gov/catalog/PIA01637. Retrieved 2012-07-22.
- ↑ 56.0 56.1 56.2 56.3 Jonathan I. Lunine (1993). "The Atmospheres of Uranus and Neptune". Annual Review of Astronomy and Astrophysics 31: 217–63 1993. doi:10.1146/annurev.aa.31.090193.001245.
- ↑ Conrath, B.; Gautier, D.; Hanel, R.; Lindal, G.; Marten, A. (1987). "The helium abundance of Uranus from Voyager measurements". Journal of Geophysical Research: Space Physics 92: 15003–15010. doi:10.1029/JA092iA13p15003.
- ↑ Pearl, J.C.; Conrath, B.J.; Hanel, R.A.; Pirraglia, J.A.; Coustenis, A. (1990). "The albedo, effective temperature, and energy balance of Uranus, as determined from Voyager IRIS data". Icarus 84: 12–28. doi:10.1016/0019-1035(90)90155-3.
- ↑ Lindal, G. F.; Lyons, J. R.; Sweetnam, D. N.; Eshleman, V. R.; Hinson, D. P.; Tyler, G. L. (1987). "The atmosphere of Uranus: Results of radio occultation measurements with Voyager 2". Journal of Geophysical Research: Space Physics 92: 14987–15001. doi:10.1029/JA092iA13p14987.
- ↑ Tyler, J.L.; Sweetnam, D.N.; Anderson, J.D.; Campbell, J. K.; Eshleman, V. R.; Hinson, D. P.; Levy, G. S.; Lindal, G. F. et al. (1986). "Voyger 2 Radio Science Observations of the Uranian System: Atmosphere, Rings, and Satellites". Science 233 (4759): 79–84. doi:10.1126/science.233.4759.79. PMID 17812893.
- ↑ 61.0 61.1 Bishop, J.; Atreya, S.K.; Herbert, F.; Romani, P. (1990). "Reanalysis of voyager 2 UVS occultations at Uranus: Hydrocarbon mixing ratios in the equatorial stratosphere". Icarus 88 (2): 448–464. doi:10.1016/0019-1035(90)90094-P.
- ↑ Summers, Michael E.; Strobel, Darrell F. (1989). "Photochemistry of the atmosphere of Uranus". The Astrophysical Journal 346: 495. doi:10.1086/168031.
- ↑ 63.0 63.1 Burgdorf, M.; Orton, G.; Vancleve, J.; Meadows, V.; Houck, J. (2006). "Detection of new hydrocarbons in Uranus' atmosphere by infrared spectroscopy". Icarus 184 (2): 634–637. doi:10.1016/j.icarus.2006.06.006.
- ↑ 64.0 64.1 Encrenaz, Thérèse (2003). "ISO observations of the giant planets and Titan: What have we learnt?". Planetary and Space Science 51 (2): 89–103. doi:10.1016/S0032-0633(02)00145-9.
- ↑ Encrenaz, Th.; Lellouch, E.; Drossart, P.; Feuchtgruber, H.; Orton, G. S.; Atreya, S. K. (2004). "First detection of CO in Uranus". Astronomy & Astrophysics 413 (2): L5–L9. doi:10.1051/0004-6361:20034637.
- ↑ 66.0 66.1 Kirk Munsell; Harman Smith; Samantha Harvey (13 November 2007). Neptune overview, In: Solar System Exploration. NASA. http://solarsystem.nasa.gov/planets/profile.cfm?Object=Neptune&Display=OverviewLong. Retrieved 20 February 2008.
- ↑ Hubbard, W. B.. "Neptune's Deep Chemistry". Science 1997 275 (5304): 1279–1280. doi:10.1126/science.275.5304.1279. PMID 9064785.
- ↑ D. Crisp; H. B. Hammel (14 June 1995). Hubble Space Telescope Observations of Neptune. Hubble News Center. http://hubblesite.org/newscenter/archive/releases/1995/09/image/a/. Retrieved 22 April 2007.
- ↑ 69.0 69.1 69.2 D. K. Yeomans (1998). Great Comets in History. Jet Propulsion Laboratory. http://ssd.jpl.nasa.gov/?great_comets. Retrieved 15 March 2007.
- ↑ D. A. Mendis. "A Postencounter view of comets". Annual Review of Astronomy and Astrophysics 26 (1): 11–49 1988. doi:10.1146/annurev.aa.26.090188.000303.
- ↑ Ian Ridpath (1985). Through the comet’s tail. Revised extracts from A Comet Called Halley by Ian Ridpath, published by Cambridge University Press in 1985. http://www.ianridpath.com/halley/halley12.htm. Retrieved 2011-06-19.
- ↑ Brian Nunnally (May 16, 2011). This Week in Science History: Halley’s Comet. pfizer: ThinkScience Now. http://science.pfizer.com/content/this-week-in-science-history-halleys-comet/. Retrieved 2011-06-19.
- ↑ "Yerkes Observatory Finds Cyanogen in Spectrum of Halley's Comet". The New York Times. 8 February 1910. Retrieved 15 November 2009.
- ↑ 74.0 74.1 "Ten Notable Apocalypses That (Obviously) Didn't Happen". Smithsonian magazine 2009. Retrieved 14 November 2009.
- ↑ Interesting Facts About Comets. Universe Today 2009. http://www.universetoday.com/tag/comet-nucleus/. Retrieved 15 January 2009.
- ↑ Heber D. Curtis (June 1910). "Photographs of Halley's Comet made at the Lick Observatory". Publications of the Astronomical Society of the Pacific 22 (132): 117-30.
- ↑ Alan Stern; Colwell, Joshua E.. "Collisional Erosion in the Primordial Edgeworth-Kuiper Belt and the Generation of the 30–50 AU Kuiper Gap". The Astrophysical Journal 490 (2): 879–882 1997. doi:10.1086/304912.
- ↑ Jane Luu; David Jewitt (November 1996). "Color Diversity among the Centaurs and Kuiper Belt Objects". The Astronomical Journal 112 (5): 2310-8. http://adsabs.harvard.edu/full/1996AJ....112.2310L. Retrieved 2013-11-05.
- ↑ The MPC Orbit (MPCORB) Database. http://www.cfa.harvard.edu/iau/MPCORB.html.
- ↑ Carl D. Murray; Stanley F. Dermott. Solar System Dynamics. Cambridge University Press 1999. ISBN 0-521-57295-9.
- ↑ R Pier; HC Harris; DE Vanden Berk (December 2000). "SDSS Stellar Spectroscopy". Bulletin of the American Astronomical Society 32 (12): 1424. http://adsabs.harvard.edu/abs/2000AAS...197.1310P. Retrieved 2013-07-15.
- ↑ Robert Nemiroff; Jerry Bonnell (November 2, 2003). Astronomy Picture of the Day. Washington, DC USA: NASA. http://apod.nasa.gov/apod/ap031102.html. Retrieved 2014-08-31.
- ↑ K. Strassmeier (November 2, 2003). Giant starspot. NOAO. http://www.noao.edu/image_gallery/html/im0571.html. Retrieved 2014-08-31.
- ↑ 84.0 84.1 84.2 Caty Pilachowski (December 1999). XX Marks the Spot. NOAO. http://www.noao.edu/noao/noaonews/dec99/node2.html. Retrieved 2014-08-31.
- ↑ Too Close for Comfort. NASA. 7 August 2003. http://hubblesite.org/newscenter/archive/releases/2003/21/. Retrieved 2010-01-21.
- ↑ Astronomy Picture of the Day - Blue Stragglers in NGC 6397. 22 June 2000. http://antwrp.gsfc.nasa.gov/apod/ap000622.html. Retrieved 2010-01-18.
- ↑ double star. San Francisco, California: Wikimedia Foundation, Inc. July 14, 2013. http://en.wiktionary.org/wiki/double_star. Retrieved 2013-07-14.
- ↑ optical double. San Francisco, California: Wikimedia Foundation, Inc. June 16, 2013. http://en.wiktionary.org/wiki/optical_double. Retrieved 2013-07-14.
- ↑ visual binary. San Francisco, California: Wikimedia Foundation, Inc. June 16, 2013. http://en.wiktionary.org/wiki/visual_binary. Retrieved 2013-07-14.
- ↑ star cluster. San Francisco, California: Wikimedia Foundation, Inc. June 19, 2013. http://en.wiktionary.org/wiki/star_cluster. Retrieved 2013-07-14.
- ↑ open cluster. San Francisco, California: Wikimedia Foundation, Inc. June 16, 2013. http://en.wiktionary.org/wiki/open_cluster. Retrieved 2013-07-14.
- ↑ globular cluster. San Francisco, California: Wikimedia Foundation, Inc. June 20, 2013. http://en.wiktionary.org/wiki/globular_cluster. Retrieved 2013-07-14.
- ↑ star cloud. San Francisco, California: Wikimedia Foundation, Inc. June 19, 2013. http://en.wiktionary.org/wiki/star_cloud. Retrieved 2013-07-14.
- ↑ galaxy. San Francisco, California: Wikimedia Foundation, Inc. July 2, 2013. http://en.wiktionary.org/wiki/galaxy. Retrieved 2013-07-14.
- ↑ dwarf galaxy. San Francisco, California: Wikimedia Foundation, Inc. June 19, 2013. http://en.wiktionary.org/wiki/dwarf_galaxy. Retrieved 2013-07-14.
- ↑ asterism. San Francisco, California: Wikimedia Foundation, Inc. June 16, 2013. http://en.wiktionary.org/wiki/asterism. Retrieved 2013-07-14.
- ↑ constellation. San Francisco, California: Wikimedia Foundation, Inc. July 9, 2013. http://en.wiktionary.org/wiki/constellation. Retrieved 2013-07-14.
- ↑ J. E. Hibbard; Puragra Guhathakurta; J. H. van Gorkom; François Schweizer (January 1994). "Cold, Warm, and Hot Gas in the Late-Stage Merger NGC 7252". The Astronomical Journal 107 (1): 67-89. doi:10.1086/116835.
- ↑ J Abraham; P Abreu; M Aglietta; C Aguirre; D Allard; The Pierre Auger Collaboration (November 9, 2007). "Correlation of the highest-energy cosmic rays with nearby extragalactic objects". Science 318 (5852): 938-43. doi:10.1126/science.1151124. http://www.sciencemag.org/content/318/5852/938.short. Retrieved 2013-11-04.
- ↑ 100.0 100.1 Daniele Fargion (April 2010). "UHECR besides CenA: Hints of galactic sources". Progress in Particle and Nuclear Physics 64 (2): 363-5. doi:10.1016/j.ppnp.2009.12.049. http://www.sciencedirect.com/science/article/pii/S0146641009001276. Retrieved 2014-01-09.
External links
[edit | edit source]- African Journals Online
- Bing Advanced search
- Google Books
- Google scholar Advanced Scholar Search
- International Astronomical Union
- JSTOR
- Lycos search
- NASA/IPAC Extragalactic Database - NED
- NASA's National Space Science Data Center
- Office of Scientific & Technical Information
- Questia - The Online Library of Books and Journals
- SAGE journals online
- The SAO/NASA Astrophysics Data System
- Scirus for scientific information only advanced search
- SDSS Quick Look tool: SkyServer
- SIMBAD Astronomical Database
- SIMBAD Web interface, Harvard alternate
- Spacecraft Query at NASA
- SpringerLink
- Taylor & Francis Online
- Universal coordinate converter
- Wiley Online Library Advanced Search
- Yahoo Advanced Web Search