Chemicals/Lithiums

Lithiums is a lecture from the school of chemistry about the various lithiums that occur or are manufactured.
Cosmic rays
[edit | edit source]The "presence in ... cosmic radiation [is] of a much greater proportion of "secondary" nuclei, such as lithium, beryllium and boron, than is found generally in the universe."[1]
Neutrons
[edit | edit source]"Seven percent of a normal solar mass contains nearly 2 X 1051 iron atoms. The total number of admixed protons is of the order of 4 X 1050. Almost all of these are converted to neutrons by the 12C(p,γ)13N(e+ν)13C and 13C(α,n)16O reactions. Since significant synthesis of heavy elements requires the production of 10-102 neutrons per iron nucleus (Clayton et al. 1961; Seeger, Fowler, and Clayton 1965; Seeger and Fowler 1966), it may be seen that significant s-process production of heavy elements [such as lithium] would occur only if the metal content of the star is less than solar by two orders of magnitude."[2]
Absorptive reactions with prompt reactions - Low energy neutrons are typically detected indirectly through absorption reactions. Typical absorber materials used have high cross sections for absorption of neutrons and include Helium-3, Lithium-6, Boron-10, and Uranium-235. Each of these reacts by emission of high energy ionized particles, the ionization track of which can be detected by a number of means. Commonly used reactions include 3He(n,p) 3H, 6Li(n,α) 3H, 10B(n,α) 7Li and the fission of uranium.[3]
Protons
[edit | edit source]"Although the mean metal abundance M/H varies from a factor 12 to 250 relative to the Sun, the abundance of lithium is very constant in all these [halo and old disk] stars, but the coolest ones where the convection zone is deep enough to induce 7Li destruction by proton fusion."[4]
"[T]he lithium has a fragile nucleus which is destroyed by proton fusion when the temperature reaches 2 106 K."[4]
"[F]or the limit between "halo stars" and "old disk stars", [there is] a discontinuity in the relation δ(U - B) versus [Fe/H] at about [Fe/H] = -1.0 dex ... "halo stars" ... are metal deficient by a factor ranging from 12 to 250 relative to the Sun. ... "old disk stars" ... are metal deficient by a factor ranging from 2 to 10."[4]
These well-known metal deficient stars have "high dispersion spectra in the blue wavelength range".[4]
"In all the stars analysed ... it is very unlikely that the lithium observed in the atmosphere was produced, even in part, inside the stars themselves, and then transported up into the atmospheres."[4]
"[T]he lithium rich stars are not statistically younger than the lithium poor stars."[4]
Neutrinos
[edit | edit source]There is a practical "possibility for utilizing lithium as a solar-neutrino detector".[5]
Opticals
[edit | edit source]
The "spectral region around the lithium is blended with molecular lines of CN and TiO."[6]
The "lithium lines are much stronger in sunspots due to the lower degree of ionization and therefore the influence of blends is smaller."[6]
"In addition to the doublets of the lithium resonance lines from the isotopes Li6 and Li7 at 670.8 nm we selected the magnetically sensitive line Fe I 617.3 nm (Landé factor g = 2.5) to determine the magnetic field strength B, six nonmagnetic (g = 0) lines [Fe I 406.5, 444.3, 512.4, 543.5, 557.6, and Ni I 491.2 nm] with different atmospherical heights of formation and the K I 769.9 nm line which originates from the same atomic transition as lithium and is similar in mots line parameters except abundance."[6]
"The field strength was found to B = 2450 Gauß in an optical height of log τ500 = -2.5."[6]
The "total lithium abundance is εLi = 1.02 and the ratio Li6/Li7 = 0.02."[6]
"Some evidence for the existence of a small but notable amount of Li6 is found."[6]
Visuals
[edit | edit source]
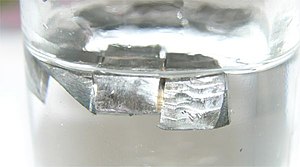
Because of its relative nuclear instability, lithium is less common in the solar system than 25 of the first 32 chemical elements even though its nuclei are very light: it is an exception to the trend that heavier nuclei are less common.[7]
The transmutation of lithium atoms to helium in 1932 was the first fully man-made nuclear reaction, and lithium deuteride serves as a fusion fuel in Teller-Ulam design staged thermonuclear weapons.[8]
Lithium is superconductive below 400 μK at standard pressure[9] and at higher temperatures (more than 9 K) at very high pressures (>20 GPa).[10]
Violets
[edit | edit source]"Violet satellite bands are caused by those lithium atoms which undergo an optical transition while a helium atom is nearby."[11]
Oranges
[edit | edit source]Lithium I has an orange line at 610.3 nm.
The Spite plateau (or Spite lithium plateau) is a baseline in the abundance of lithium found in old stars orbiting the galactic halo.
The curve on a graph of the abundance of lithium versus effective surface temperature formed a plateau among old halo stars for effective temperatures below about:
- log Teff ~ 3.75
or roughly 5,600 K. This suggested that the plateau represented the primordial abundance level of lithium in the Milky Way with an estimate that the abundance of lithium at the beginning of the galaxy was:
- NLi = (11.2 ± 3.8) × 10−11 NH
where NH is the abundance of hydrogen.[4]
"Lithium depletion through atomic diffusion has been suggested as a solution to the discrepancy between the Spite plateau abundance and the predicted value of the primordial lithium abundance"[12].
Reds
[edit | edit source]In some 824 red giant stars, the Li I 670.78 nm line was detected in several stars, "but only the five objects ... presented a strong line. Indeed, the Li subordinate line at 6103.6 Å was detected in these stars only."[13]
Infrareds
[edit | edit source]Lithium has an infrared line at 812.6 nm.[14]
Metallicities
[edit | edit source]The metallicity (also called Z[15]) of an object is the proportion of its matter made up of chemical elements other than hydrogen and helium. The metallicity of an astronomical object may provide an indication of its age. When the universe first formed, according to the Big Bang theory, it consisted almost entirely of hydrogen which, through primordial nucleosynthesis, created a sizeable proportion of helium and only trace amounts of lithium and beryllium and no heavier elements. Therefore, older stars have lower metallicities than younger stars such as our Sun.
Plasmas
[edit | edit source]Gases
[edit | edit source]"Lithium vapor is preferred when generating negative ion beams for Tandem accelerators. In all these cases, a positive ion beam interacts with the cesium or lithium vapor, contained in a charge exchange canal, communicating with a cesium or lithium reservoir."[16]
"Resonances have been observed in neutral lithium vapor by absorption spectroscopy for photons in the 50- to 70-eV energy range. These resonances are due to the excitation of a K-shell electron, or the simultaneous excitation of a K-shell electron and an outer electron to final states of the type (1s2pnl) or (1snln′l′)."[17]
Liquids
[edit | edit source]Solids
[edit | edit source]Native lithiums
[edit | edit source]As indicated in the magnesium-lithium phase diagram on the left, lithium occurs in the same crystal structure at lower temperatures as it does up to melting temperature. This is the bcc phase (α-Li).
"Native lithium is rare in nature. Most of the lithium is extracted from the mining [of] spodumene."[18]
Compounds
[edit | edit source]Lithium nitrate
[edit | edit source]


3. Credit: Ben Mills.{{fairuse}}
Compounds (right image) formed from chemical reactions (above) such as lithium nitrate are usually crystalline with a unit cell (center image), made up of one or more molecules (left, Z = number of molecules per unit cell).
Lithium chloride
[edit | edit source]
Lithium aluminium hydride
[edit | edit source]
The chemical formula for lithium aluminum hydride is LiAlH
4.
Lithium aluminium hydride, commonly abbreviated as LAH, is an inorganic compound.[19]
Organometallics
[edit | edit source]
On the right is methyl lithium.
Nucleosynthesis
[edit | edit source]- + ? MeV
"Our calculations show that production of [lithium] in low-energy flares [by nucleosynthesis], taking place in the surfaces of T Tauri-like stars, is energetically possible and can give the observed excesses over the interstellar abundance."[20]
Nucleosynthesis |
Related topics |
"[T]here is evidence of lithium production in some stars due to some undefined mechanism. The observations show that the Li abundance on some red giants ... and young stars exceeds the average abundance in the universe by 2 orders of magnitude".[21] It is "suggested that Li produced in the helium envelopes of red giants comes to the surface of the stars as the result of a strong convection."[21] For young stars, "the production of the light elements in nonthermal nuclear reactions seems the most appropriate mechanism that can be responsible for enrichment of stellar photospheres by Li."[21] "At least 0.3 metric tons of excited Li and Be nuclei were produced during the solar flare of 1991 November 15. One can estimate the equilibrium concentration of 7Li nuclei in the solar atmosphere by assuming that they are produced only in solar flares and that a leakage of Li nuclei occurs with the solar wind."[21]
Although 7Be is usually assumed to have been produced by the Big Bang nuclear fusion, excesses (100x) of the isotope on the leading edge[22] of the Long Duration Exposure Facility (LDEF) relative to the trailing edge suggest that "most of the sun's fusion must occur near the surface rather than the core."[23] The particular reaction
3He(α,γ)7Be
3He + 4He → 7Be + γ (429 keV)
and the associated reaction chains
7Be(e-,νe)7Li(p,α)α
and
7Be(p,γ)8B → 2α + e+ + νe
generate 14% and 0.1% of the α-particles, respectively, and 10.7% of the present-epoch luminosity of the Sun.[24] Usually, the 7Be produced is assumed to be deep within the core of the Sun; however, such 7Be would not escape to reach the leading edge of the LDEF.
"The light elements deuterium, lithium, beryllium, and boron pose a special problem for any theory of the origin of the elements which proposes that all the elements are built up from hydrogen in the stars. ... The difficulty arises because the lifetimes of these elements against proton capture, at the temperatures and pressures at which most stellar matter exists, are short compared to the stable lifetimes of stars. These elements then cannot be produced in stellar interiors unless they are transported rapidly to the surface, and if they are produced at the surface, non-equilibrium processes must be involved. Further, they can exist in significant quantities at the surface only in the absence of rapid mixing to the interior."[25]
Cosmic ray spallation
[edit | edit source]Def. a nuclear reaction in which a nucleus fragments into many nucleons is called spallation.
Carbon and oxygen nuclei collide with interstellar matter to form lithium, beryllium and boron in a process termed cosmic ray spallation.
Glasses
[edit | edit source]"In the other series of glasses, Li, Na, and K, in particular, that can be formed out to x=0.75, the IR spectra clearly show that this structural group becomes the dominant group in the glass at this composition [4], [5] and [43]."[26]
Glaciers
[edit | edit source]"Taylor Valley glaciers have the lowest lithium concentrations and lightest δ7Li values in the McMurdo Dry Valleys aquatic system."[27]
"The δ7Li ratios of the glacial snow are among the lightest values observed in surface and groundwater samples (+0.8 to +2.9‰) and are unlike seawater (+30.8‰) (Tomascak, 2004; Rosner et al., 2007). This suggests that marine aerosol or marine-derived salts are not the primary sources of lithium to the glacier surfaces [...] Generally, geologic materials are isotopically lighter than natural water samples because of the preferential retention of 6Li in the solid phase. Despite this general relationship, Taylor Valley glacier snow samples have δ7Li signatures more like granite and rhyolite, which range from −1.2 to +8.0‰ (Tomascak, 2004). Although this wide range of Li isotopic values for granite does not conclusively mean the source to the glaciers is felsic rock, the snow samples are extremely light and resemble aluminosilicates. We therefore conclude that the major source of Li in the glacier ice is likely the dissolution of silicate materials."[27]
Spectroscopic terms
[edit | edit source]The electronic configuration is not enough to describe the wavefunction, because for each configuration there are different ways to combine the orbital and spin angular momenta of the electrons (different combinations have different energies). The labels of a wavefunction which indicate the total angular momentum (S, P, D,...) and the spin multiplicity (singlet, doublet, triplet) are called spectroscopic terms.
You can neglect the electrons in filled sub-shells, so that the spectroscopic term of the ground state of lithium 1s22s is 2S.
Sun
[edit | edit source]"[T]he standard solar models have enjoyed tremendous success recently in terms of agreement between the predicted outer structure and the results from helioseismology[, but] some observed properties of the Sun still defy explanation, such as the degree of Li depletion" [the "solar Li abundance is roughly a factor of 200 below the meteoritic abundance"].[28]
Heliogony
[edit | edit source]"Using ESO’s very successful HARPS spectrograph, a team of astronomers has found that Sun-like stars which host planets have destroyed their lithium much more efficiently than planet-free stars. This finding does not only shed light on the low levels of this chemical element in the Sun, solving a long-standing mystery, but also provides astronomers with a very efficient way to pick out the stars most likely to host planets. It is not clear what causes the lithium to be destroyed. The general idea is that the planets or the presence of the protoplanetary disc disturb the interior of the star, bringing the lithium deeper down into the star than usual, into regions where the temperature is so hot that it is destroyed."[29]
Photospheres
[edit | edit source]The "evidence for the overwhelming majority of the Li-atoms in photospheres has its origin not only in nuclear synthesis near the stellar centers, but also by active processes in stellar atmospheres. [...] the lithium [resonance] line [is] near 478 keV."[30]
"Approximately 90% of lithium atoms originate from α - α reactions for the typical spectra of an accelerated particles on the Sun [...] During impulsive flares, interaction between the accelerated particles and the ambient medium occurs mainly at low altitudes, i.e., close to the footprints of loops."[30]
Solar winds
[edit | edit source]"The solar wind lithium isotopic ratio, (6Li/7Li)sw = 0.032±0.004, has recently been determined from measurements in lunar soil (Chaussidon & Robert 1999)."[31]
"Light element production by accelerated particle interactions [in] non-solar settings, and for accelerated particles of predominantly low energy, the dominant reactions are 4He(α,p)7Li, 4He(α,n)7Be (with 7Be decaying to 7Li) and 4He(α,x)6Li (where x stands for either a proton and a neutron, or a deuteron)."[31]
"In solar flares, [...] the reaction 4He(3He,p)6Li [has a] very low threshold energy and [...] for solar energetic particles 3He/4He can be as large as 1 or even larger (e.g. Reames 1998). Such 3He/4He enhancements are one of the main characteristics of the acceleration mechanism responsible for impulsive solar energetic particle events, as distinguished from gradual events, based on the duration of the accompanying soft X-ray emission."[31]
Flare "accelerated particle interactions produce enough 6Li which, combined with photospheric 7Li, can account for the solar wind 6Li/7Li measured in lunar soil."[31]
Although the 3.56 MeV line is usually missing from the gamma-ray spectrum during solar flares, there is evidence for significant production of 6Li in large solar flares by optical observations of sunspots,[17] and measurements of solar wind Li isotopic ratio in lunar soil.[18]
The "fact that as much as 1030 Li atoms are produced in large solar flares, suggests that flare produced lithium may be detected in a small area of the solar surface near the foot points of the flaring loops shortly after the time of the flare (see Livshits 1997). In this connection, it is interesting to point out that Ritzenhoff et al. (1997) don’t rule out the presence of 6Li near a sunspot at a value close to their reported upper limit 6Li/7Li ≤ 0.03, which in fact coincides with the measured solar wind value."[31]
Stars
[edit | edit source]TX Piscium (19 Piscium) is "a Li-rich carbon star".[32]
"Super Lithium Rich giants (SLIR) are an elusive class of objects, serendipitously discovered."[33]
"J37 has a Li abundance well above the meteoritic value ... Al, S, Si, Ca, Fe, and Ni are supersolar and that C is subsolar. ... Na, Sc, Ti are supersolar, while O is subsolar."[34]
"J37 has a Li abundance near A(Li) = 4.3
1 dex above the solar system meteoritic value."[34]
"Stars with an initial mass less than the solar mass have a large deficiency of light elements — lithium, beryllium, and boron — which burn up almost completely both in the interior and in the convective envelope."[35]
Solar twins
[edit | edit source]Solar twins have the following qualities:[36]
- Temperature within 50 K Solar (roughly 5720 to 5830 K)
- Metallicity of 89—112% (± 0.05 dex) Solar, meaning the star's proplyd would have had almost exactly the same amount of dust for planetary formation
- No stellar companion, because the Sun itself is solitary
- An age within 1 billion years Solar (roughly 3.5 to 5.6 Ga)
Identifier | Coordinates[37] | Distance[37] (ly) |
Stellar Class[37] |
Temperature (K) |
Metallicity (dex) |
Age (Gyr) |
Notes | |
---|---|---|---|---|---|---|---|---|
Right ascension | Declination | |||||||
Sun | — | — | 0.00 | G2V | 5,778 | +0.00 | 4.6 | [38] |
18 Scorpii | 16h 15m 37.3s | –08° 22′ 06″ | 45.1 | G2Va | 5,835 | +0.04 | 4.2 | Low Lithium Abundance[39] |
HD 44594 | 06h 20m 06.1s | -48° 44′ 29″ | 84 | G3V | 5,840 | +0.15 | 4.1 | Higher depletion of lithium[40] |
HD 195034 | 20h 28m 11.8s | +22° 07′ 44″ | 92 | G5 | 5,760 | -0.04 | 5.1 | [41] |
HD 138573 | 15h 32m 43.7s | +10° 58′ 06″ | 101 | G5IV-V | 5,710 | –0.03 | 7.8 | [42] |
HD 142093 | 15h 52m 00.6s | +15° 14′ 09″ | 103 | G2V | 5,841 | –0.15 | 5.0 | [42] |
HD 98618 | 11h 21m 29.1s | +58° 29′ 04″ | 126 | G5V | 5,851 | +0.03 | 4.7 | Low Lithium Abundance[39] |
HD 143436 | 16h 00m 18.8s | +00° 08′ 13″ | 141 | G0 | 5,768 | +0.00 | 3.8 | [42] |
HD 129357 | 14h 41m 22.4s | +29° 03′ 32″ | 154 | G2V | 5,749 | –0.02 | 8.2 | [42] |
HD 133600 | 15h 05m 13.2s | +06° 17′ 24″ | 171 | G0 | 5,808 | +0.02 | 6.3 | Low Lithium Abundance[39] |
HD 101364 | 11h 40m 28.5s | +69° 00′ 31″ | 208 | G5V | 5,795 | +0.02 | 3.5 | Low Lithium Abundance[39][43] |
Binary twins
[edit | edit source]16 Cyg A and B are binary solar twins.[44]
"16 Cygni or 16 Cyg is a triple star system approximately 69 light-years away from Earth in the constellation of Cygnus. It consists of two Sun-like yellow dwarf stars, 16 Cygni A and 16 Cygni B, together with a red dwarf, 16 Cygni C. In 1996 an extrasolar planet was discovered in an eccentric orbit around 16 Cygni B.
16 Cygni is a hierarchal triple system. Stars A and C form a close binary with a projected separation of 73 AU.[45] The orbital elements of the A–C binary are currently unknown. At a distance of 860 AU from A is a third component designated 16 Cygni B.
"B orbits between 100 and 160 degrees inclination, that is against the A–C pole such that 90 degrees would be ecliptical.[46]
"Both 16 Cygni A and 16 Cygni B are yellow dwarf stars like our Sun. According to data from the Geneva–Copenhagen survey, both stars have masses similar to the Sun.[47][48] Age estimates for the two stars vary slightly, but 16 Cygni is likely to be much older than the Solar System, at around 10,000 million years old. 16 Cygni C is much fainter than either of these stars, and may be a red dwarf.[45]
"Despite differing in Teff by only 35-40 K, the Li abundances of 16 Cyg A and B differ by a factor of ≥ 4.5. The solar photospheric abundance is intermediate to the two values. This intermediacy indicates that the Sun, whose highly depleted photospheric Li abundance is in gross conflict with standard stellar models, is not an isolated anomaly in its Li abundance evolution."[44]
"In 1996 an extrasolar planet in an eccentric orbit was announced around the star 16 Cygni B.[49] The planet's orbit takes 798.5 days to complete, with a semimajor axis of 1.68 AU.[50]
""For the 16 Cyg B system, only particles inside of about 0.3 AU remained stable [within a million years of formation], leaving open the possibility of short-period planets". For them, observation rules out any such planet of over a Neptune mass.[51]
Giant stars
[edit | edit source]
Notation: let the symbol AGB indicate an asymptotic giant branch star with a hydrogen-exhausted core.
Notation: let the symbol E-AGB indicate an AGB star with a hydrogen-exhausted core.
For a 7 M⊙ AGB model sequence, "[o]n the E-AGB, the convective envelope appears clearly separated from the stellar core by a radiative layer ... Density and temperature drop significantly within this layer".[52] "As evolution proceeds luminosity and radiation pressure increase ... The base of the convective envelope moves inwards into deeper and hotter parts of the interior until nuclear reactions become important ... just before the first thermal pulse ..., the radiative "buffer" layer disappears, and the convection cuts into the hydrogen-burning shell. ... high lithium abundances ... in ... oxygen rich, luminous (Mbol = -6.2... -6.8) AGB stars [are produced at the base of the convective envelope which] has a base temperature of 75 ˑ 106K, sufficient to reduce the duration of the Li-rich phase well below 104yrs".[52]
Red giants
[edit | edit source]In some 824 red giant stars, the Li I 670.78 nm line was detected in several stars, "but only the five objects ... presented a strong line."[13]
"The lithium content of red-giant stars is highly variable (Wallerstein and Conti 1969). The largest amounts of lithium are found in three carbon stars, WZ Cas, WX Cyg, and T Ara, being of the order of 10-2 of calcium. ... Boesgaard (1970) has found a similar high lithium abundance in the S star T Sgr. This is a higher ratio of lithium to calcium than is found in T Tauri stars or in meteorites."[2]
"[S]ince the majority of carbon and S giants do not possess nearly so much lithium [as has been detected in some red-giant stars], it is necessary to postulate that the production process involves some unusual events."[2]
"[H]elium-burning shell flashes in advanced stages of stellar evolution [may] occasionally induce complete convection of the outer envelope down to the helium-burning shell. If the hydrogen mixing is relatively small for the first 107 seconds, the result may be the production of large amounts of heavy elements by the s-process. When complete mixing commences, the 3He in the envelope will be converted to 7Be, and the subsequent delayed electron capture to form 7Li may allow enough lithium to remain near the surface to account for the very large lithium abundances in some S and carbon red-giant stars. ... the 7Li/6Li ratio in these stars should be quite large (> 100)."[2]
Brown dwarfs
[edit | edit source]Some of the incontrovertible brown dwarf substellar objects are "identified by the presence of the 670.8 nm lithium [I] line. The most notable of these objects was Gliese 229B, which was found to have a temperature and luminosity well below the stellar range. Remarkably, its near-infrared spectrum clearly exhibited a methane absorption band at 2 micrometres, a feature that had previously only been observed in gas giant atmospheres and the atmosphere of Saturn's moon, Titan. Methane absorption is not expected at the temperatures of main-sequence stars. This discovery helped to establish yet another spectral class even cooler than L dwarfs known as "T dwarfs" for which Gl 229B is the prototype. ... Lithium is generally present in brown dwarfs and not in low-mass stars. [T]he presence of the lithium line in a candidate brown dwarf's spectrum is a strong indicator that it is indeed substellar. The use of lithium to distinguish candidate brown dwarfs from low-mass stars is commonly referred to as the lithium test ... Some brown dwarfs emit X-rays; and all "warm" dwarfs continue to glow tellingly in the red and infrared spectra until they cool to planetlike temperatures (under 1000 K)."[53]
L stars
[edit | edit source]A "new spectral class L [has the major spectroscopic signatures of] metallic oxides [...] replaced by metallic hydrides and neutral alkali metals [...]."[54]
"At least five of the 20 2MASS L dwarfs show the 6708 Å lithium doublet at low resolution, the strongest having an equivalent width of 18.5 Å."[54]
Galactic halos
[edit | edit source]The Spite plateau (or Spite lithium plateau) is a baseline in the abundance of lithium found in old stars orbiting the galactic halo.
Cosmogony
[edit | edit source]"From the cosmogony point of view, there can be many different pathways to form a planet, and this casts some doubt that planet formation could be either an adequate physical quantity or a useful observational criterion to define what a planet is. In practice, it is convenient to adopt a planet definition that heavily relies on the mass of the object because the mass can be either measured directly or it has an impact on observable quantities such as surface gravity. For solar composition the boundary [brown dwarfs] BDs and planets is determined by deuterium fusion, which ceases to be stable at around 13 Jupiter masses [18, 19]. Just as the lithium test has effectively been applied as a tool to distinguish between very low-mass stars and BDs [6, 43, 46, 62, 72], the deuterium test has been proposed to distinguish between BDs and planets [9] but it has not been carried out yet because it is observationally very challenging. This important observational test may have to wait for the advent of the 30-meter class generation of ground-based telescopes such as the European Extremely Large Telescope or the American Thirty Meter Telescope. Particularly promising targets are nearby late-T dwarfs with effective temperatures around 500K that appear to have peculiar properties indicative of young age and planetary mass, such as for example ULASJ1335+11 [37]."[55]
"A possible link between lithium depletion, rotational history and the presence of exoplanets has been explored for solar-type main-sequence stars [15]."[55]
Gamma-ray detectors
[edit | edit source]
Germanium detectors are mostly used for spectroscopy in nuclear physics. Germanium can have a depleted, sensitive thickness of centimeters, and therefore can be used as a total absorption detector for gamma rays up to few MeV. These detectors are also called high-purity germanium detectors (HPGe) or hyperpure germanium detectors. Germanium crystals were doped with lithium ions (Ge(Li)), in order to produce an intrinsic region in which the electrons and holes would be able to reach the contacts and produce a signal. HPGe detectors commonly use lithium diffusion to make an n+ ohmic contact, and boron implantation to make a p+ contact. Coaxial detectors with a central n+ contact are referred to as n-type detectors, while p-type detectors have a p+ central contact. The thickness of these contacts represents a dead layer around the surface of the crystal within which energy depositions do not result in detector signals.
Lithium-drifted silicon detectors
[edit | edit source]Since the 1970s, new semiconductor detectors have been developed (silicon or germanium doped with lithium: Si(Li) or Ge(Li)). X-ray photons are converted to electron-hole pairs in the semiconductor and are collected to detect the X-rays. When the temperature is low enough (the detector is cooled by Peltier effect or even cooler liquid nitrogen), it is possible to directly determine the X-ray energy spectrum; this method is called energy dispersive X-ray spectroscopy (EDX or EDS); it is often used in small X-ray fluorescence spectrometers. These detectors are sometimes called "solid state detectors". Detectors based on cadmium telluride (CdTe) and its alloy with zinc, cadmium zinc telluride, have an increased sensitivity, which allows lower doses of X-rays to be used.
Ultraviolet telescopes
[edit | edit source]Ultraviolet telescopes 10 nm - 400 nm resemble optical telescopes, but conventional aluminium-coated mirrors cannot be used and alternative coatings such as magnesium fluoride or lithium fluoride are used instead.
Far Ultraviolet Spectroscopic Explorer
[edit | edit source]
The Far Ultraviolet Spectroscopic Explorer (FUSE) detected light in the far ultraviolet portion of the electromagnetic spectrum, between 90.5-119.5 nanometres, which is mostly unobservable by other telescopes. Its primary mission was to characterize universal deuterium in an effort to learn about the stellar processing times of deuterium left over from the Big Bang. The telescope comprises four individual mirrors. Each of the four mirrors is a 39-by-35 cm (15.4-by-13.8 in) off-axis parabola. Two mirror segments are coated with silicon carbide for reflectivity at the shortest ultraviolet wavelengths, and two mirror segments are coated with lithium fluoride over aluminum that reflects better at longer wavelengths. Each mirror has a corresponding astigmatism-corrected, holographically-ruled diffraction grating, each one on a curved substrate so as to produce four 1.65 m (5.4 ft) Rowland circle spectrographs. The dispersed ultraviolet light is detected by two microchannel plate intensified double delay-line detectors, whose surfaces are curved to match the curvature of the focal plane.[56]
Recent history
[edit | edit source]The recent history period dates from around 1,000 b2k to present.
"Lithium was discovered in 1817 by Johann Arfvedson in Stockholm, Sweden. The name is derived from the Greek, [λίθος] (lithos), meaning stone."[57]
Hypotheses
[edit | edit source]- Lithium can be produced by muon induced fusion.
See also
[edit | edit source]References
[edit | edit source]- ↑ Thomas K. Gaisser (1990). Cosmic Rays and Particle Physics. Cambridge University Press. pp. 279. ISBN 0521339316. http://books.google.com/books?hl=en&lr=&id=qJ7Z6oIMqeUC&oi=fnd&pg=PR15&ots=IxjwLxBwXu&sig=voHKIYstBlBYla4jcbur_b-Zwxs. Retrieved 2014-01-11.
- ↑ 2.0 2.1 2.2 2.3 A. G. W. Cameron; W. A. Fowler (February 1971). "Lithium and the s-PROCESS in Red-Giant Stars". The Astrophysical Journal 164 (02): 111-4. doi:10.1086/150821. http://adsabs.harvard.edu/abs/1971ApJ...164..111C. Retrieved 2013-08-01.
- ↑ Tsoulfanidis, Nicholas (1995). Measurement and Detection of Radiation. Washington, D.C.: Taylor & Francis. pp. 467–501. ISBN 1-56032-317-5.
- ↑ 4.0 4.1 4.2 4.3 4.4 4.5 4.6 F. Spite; M. Spite (November 1982). "Abundance of lithium in unevolved halo stars and old disk stars - Interpretation and consequences". Astronomy and Astrophysics 115 (2): 357-66. http://adsabs.harvard.edu/abs/1982A%26A...115..357S. Retrieved 2013-05-31.
- ↑ E. P. Veretenkin; V. N. Gavrin; E. A. Yanovich (January 1, 1985). "Use of metallic lithium for detecting solar neutrinos". Soviet Atomic Energy 58 (1): 82-3. doi:10.1007/BF01123252. http://link.springer.com/article/10.1007%2FBF01123252?LI=true. Retrieved 2014-02-08.
- ↑ 6.0 6.1 6.2 6.3 6.4 6.5 S. Ritzenhoff; E.H. Schrter; W. Schmidt (December 1997). "The lithium abundance in sunspots". Astronomy and Astrophysics 328 (12): 695-701. http://adsabs.harvard.edu/abs/1997A%26A...328..695R. Retrieved 2014-04-08.
- ↑ Numerical data from: Lodders, Katharina (10 July 2003). "Solar System Abundances and Condensation Temperatures of the Elements". The Astrophysical Journal (The American Astronomical Society) 591 (2): 1220–1247. doi:10.1086/375492. https://web.archive.org/web/20151107043527/http://weft.astro.washington.edu/courses/astro557/LODDERS.pdf. Graphed at File:SolarSystemAbundances.jpg
- ↑ Nuclear Weapon Design. Federation of American Scientists (1998-10-21). fas.org
- ↑ Tuoriniemi, Juha; Juntunen-Nurmilaukas, Kirsi; Uusvuori, Johanna; Pentti, Elias; Salmela, Anssi; Sebedash, Alexander (2007). "Superconductivity in lithium below 0.4 millikelvin at ambient pressure". Nature 447 (7141): 187–9. doi:10.1038/nature05820. PMID 17495921.
- ↑ Struzhkin, V. V.; Eremets, M. I.; Gan, W; Mao, H. K.; Hemley, R. J. (2002). "Superconductivity in dense lithium". Science 298 (5596): 1213–5. doi:10.1126/science.1078535. PMID 12386338.
- ↑ G. D. Mahan (April 24, 1972). "Violet satellite bands in the spectra of Li perturbed by He". Physics Letters A 39 (2): 145-6. doi:10.1016/0375-9601(72)91056-0. http://www.sciencedirect.com/science/article/pii/0375960172910560. Retrieved 2013-03-23.
- ↑ K. Lind; M. Asplund; P. S. Barklem (August 2009). "Departures from LTE for neutral Li in late-type stars". Astronomy and Astrophysics 503 (2): 541-4. doi:10.1051/0004-6361/200912221.
- ↑ 13.0 13.1 L. Monaco; S. Villanova; C. Moni Bidin; G. Carraro; D. Geisler; P. Bonifacio; O. A. Gonzalez; M. Zoccali et al. (May 2011). "Lithium-rich giants in the Galactic thick disk". Astronomy & Astrophysics 529 (5): 10. doi:10.1051/0004-6361/201016285.
- ↑ L. A. Yakovina; Ya. V. Pavlenko (October 2001). "On the lithium abundance determination in the atmospheres of super-Li-RICH CARBON stars using the resonance and subordinate Li I lines. I". Kinematika i Fizika Nebesnykh Tel 17 (5): 446-58. http://adsabs.harvard.edu/abs/2001KFNT...17..446Y. Retrieved 2012-08-03.
- ↑ http://ned.ipac.caltech.edu/level5/Kunth/Kunth1.html
- ↑ Martha Bacal and Walter Reichelt (1974). "Metal vapor confinement in vacuum". Review of Scientific Instruments 45 (6): 769. doi:10.1063/1.1686733. https://aip.scitation.org/doi/abs/10.1063/1.1686733. Retrieved 22 May 2019.
- ↑ D. L. Ederer, T. Lucatorto, and R. P. Madden (30 November 1970). "Autoionization Spectra of Lithium". Physical Review Letters 25 (22): 1537-9. doi:10.1103/PhysRevLett.25.1537. https://journals.aps.org/prl/abstract/10.1103/PhysRevLett.25.1537. Retrieved 22 May 2019.
- ↑ Shanghai Xuanshi Machinery Co., Ltd. (2011). "Xuanshi". Shanghai, PRC: Shanghai Xuanshi Machinery Co., Ltd. Retrieved 2015-08-22.
- ↑ Finholt, A. E.; Bond, A. C.; Schlesinger, H. I. (1947). "Lithium Aluminum Hydride, Aluminum Hydride and Lithium Gallium Hydride, and Some of their Applications in Organic and Inorganic Chemistry". Journal of the American Chemical Society 69 (5): 1199–1203. doi:10.1021/ja01197a061.
- ↑ Ramon Canal (May 1974). "Nucleosynthesis of Lithium in Low-Energy Flares". The Astrophysical Journal 189 (5): 531-4. doi:10.1086/152831.
- ↑ 21.0 21.1 21.2 21.3 Yu. D. Kotov; S. V. Bogovalov; O. V. Endalova; M. Yoshimori (December 1996). "7Li Production in Solar Flares". The Astrophysical Journal 473 (12): 514-8. doi:10.1086/178162.
- ↑ G. J. Fishman; B. A. Harmon; J. C. Gregory; T. A. Parnell; P. Peters; G. W. Phillips; S. E. King; R. A. Augusts et al. (February 1991). "Observation of 7Be on the surface of LDEF spacecraft". Nature 349 (6311): 678-80. doi:10.1038/349678a0.
- ↑ Maurice Dubin; Robert K. Soberman (April 1996). Resolution of the Solar Neutrino Anomaly. pp. 8. http://arxiv.org/pdf/astro-ph/9604074v1. Retrieved 2011-11-08.
- ↑ M. Krčmar; Z. Krečak; A. Ljubičić; M. Stipčević; D. A. Bradley (November 2001). "Search for solar axions using 7Li". Physical Review D 64 (11): 4. doi:10.1103/PhysRevD.64.115016. http://prd.aps.org/abstract/PRD/v64/i11/e115016. Retrieved 2011-11-08.
- ↑ Walter K. Bonsack (November 1959). "The Abundance of Lithium and Convective Mixing in Stars of Type K". The Astrophysical Journal 130 (11): 843-71. doi:10.1086/146777.
- ↑ Jaephil Cho; Steve W Martin (March 2002). "Infrared spectroscopy of glasses and polycrystals in the series xCs2S+(1−x)B2S3". Journal of non-crystalline solids 298 (2-3): 176-92. http://www.sciencedirect.com/science/article/pii/S0022309302009171. Retrieved 2013-08-28.
- ↑ 27.0 27.1 RA Witherow; WB Lyons; GM Henderson (2010). "Lithium isotopic composition of the McMurdo Dry Valleys aquatic systems". Chemical Geology 275: 139-47. doi:10.1016/j.chemgeo.2010.04.017. ftp://ftp.seti.org/jbishop/AntarcticDryValleys/papers/Witherow(2010)ChemGeol_Li_isotopes_DryValleys.pdf. Retrieved 2014-09-20.
- ↑ Jeremy R. King; Constantine P. Deliyannis; Merchant Boesgaard (April 1, 1997). "The 9Be Abundances of α Centauri A and B and the Sun: Implications for Stellar Evolution and Mixing". The Astrophysical Journal 478 (2): 778. http://iopscience.iop.org/0004-637X/478/2/778/pdf/0004-637X_478_2_778.pdf. Retrieved 2012-07-11.
- ↑ L. Calçada (11 November 2009). "Burning lithium inside a star (artist's impression)". European Southern Observatory. Retrieved 2014-07-31.
- ↑ 30.0 30.1 M. A. Livshits (July 1997). "The Amount of Lithium Produced during Impulsive Flares". Solar Physics 173 (2): 377-81. doi:10.1023/A:1004958522216. http://link.springer.com/article/10.1023/A:1004958522216#page-1. Retrieved 2014-10-01.
- ↑ 31.0 31.1 31.2 31.3 31.4 Reuven Ramaty; Vincent Tatischeff; J. P. Thibaud; Benzion Kozlovsky; Natalie Mandzhavidze (May 2000). "6Li from Solar Flares". The Astrophysical Journal 534 (2): L207-10. doi:10.1086/312671. http://adsabs.harvard.edu/abs/2000ApJ...534L.207R. Retrieved 2014-04-08.
- ↑ Aruna Goswami; N. Kameswara Rao; David L. Lambert; Guillermo Gonzalez (July 1997). "On the Hydrogen Deficient Nature of Z Ursae Minoris". Publications of the Astronomical Society of the Pacific 109: 796-8. doi:10.1086/133946. http://adsabs.harvard.edu/abs/1997PASP..109..796G. Retrieved 2013-07-14.
- ↑ V Hill; L Pasquini (August 1999). "A Super Lithium Rich giant in the metal-poor open cluster Berkeley 21". Astronomy and Astrophysics 348 (08): L21-4. http://adsabs.harvard.edu/abs/1999A%26A...348L..21H. Retrieved 2013-07-14.
- ↑ 34.0 34.1 Chris Laws; Guillermo Gonzalez (October 1, 2003). "A Reevaluation of the Super-LITHIUM-rich Star in NGC 6633". The Astrophysical Journal 595 (2): 1148-53. doi:10.1086/377449. http://iopscience.iop.org/0004-637X/595/2/1148. Retrieved 2013-07-14.
- ↑ AV Tutukov (July-August 1991). "Formation of Planetary Systems during the Evolution of Close Binary Stars". Soviet Astronomy 35 (4): 415-7. http://adsabs.harvard.edu/full/1991SvA....35..415T. Retrieved 2013-12-23.
- ↑ D. R. Soderblom; J. R. King (1998). "Solar-Type Stars: Basic Information on Their Classification and Characterization". Solar Analogs : Characteristics and Optimum Candidates. http://www.lowell.edu/users/jch/workshop/drs/drs-p1.html. Retrieved 2008-02-26.
- ↑ 37.0 37.1 37.2 SIMBAD Astronomical Database. Centre de Données astronomiques de Strasbourg. http://simbad.u-strasbg.fr/simbad/. Retrieved 2009-01-14.
- ↑ Williams, D.R. (2004). Sun Fact Sheet. NASA. http://nssdc.gsfc.nasa.gov/planetary/factsheet/sunfact.html. Retrieved 2009-06-23.
- ↑ 39.0 39.1 39.2 39.3 Meléndez, Jorge; Ramírez, Iván (November 2007). "HIP 56948: A Solar Twin with a Low Lithium Abundance". The Astrophysical Journal 669 (2): L89–L92. doi:10.1086/523942.
- ↑ Sousa, S. G.; Fernandes, J.; Israelian, G.; Santos, N. C. (March 2010). "Higher depletion of lithium in planet host stars: no age and mass effect". Astronomy and Astrophysics 512: L5. doi:10.1051/0004-6361/201014125.
- ↑ Takeda, Y.; Tajitsu (2009). "High-Dispersion Spectroscopic Study of Solar Twins: HIP 56948, HIP 79672, and HIP 100963". Publications of the Astronomical Society of Japan 61: 471.
- ↑ 42.0 42.1 42.2 42.3 King, Jeremy R.; Boesgaard, Ann M.; Schuler, Simon C. (November 2005). "Keck HIRES Spectroscopy of Four Candidate Solar Twins". The Astronomical Journal 130 (5): 2318–25. doi:10.1086/452640.
- ↑ Vázquez, M.; Pallé, E.; Rodríguez, P. Montañés (2010). Is Our Environment Special?, In: The Earth as a Distant Planet: A Rosetta Stone for the Search of Earth-Like Worlds. Springer New York. pp. 391–418. doi:10.1007/978-1-4419-1684-6. ISBN 978-1-4419-1683-9.
- ↑ 44.0 44.1 Jeremy R. King; Constantine P. Deliyannis; Daniel D. Hiltgen; Alex Stephens; Katia Cunha; Ann Merchant Boesgaard (05 1997). "Lithium Abundances in the Solar Twins 16 Cyg A and B and the Solar Analog α Cen A, Calibration of the 6707 Å Li Region Linelist, and Implications". The Astronomical Journal 113 (5): 1871-83. doi:10.1086/118399. http://adsabs.harvard.edu/abs/1997AJ....113.1871K. Retrieved 2013-08-06.
- ↑ 45.0 45.1 Deepak Raghavan, Todd J. Henry, Brian D. Mason, John P. Subasavage, Wei‐Chun Jao, Thom D. Beaulieu, Nigel C. Hambly (2006). "Two Suns in The Sky: Stellar Multiplicity in Exoplanet Systems". The Astrophysical Journal 646 (1): 523–542. doi:10.1086/504823. http://www.iop.org/EJ/article/0004-637X/646/1/523/64035.html.
- ↑ H. Hauser and G. Marcy (1999). "The Orbit of 16 Cygni AB". Publications of the Astronomical Society of the Pacific 111 (757): 321–34. doi:10.1086/316328. http://www.journals.uchicago.edu/doi/full/10.1086/316328.
- ↑ Holmberg (2007). Record 13627, In: Geneva-Copenhagen Survey of Solar neighbourhood. http://vizier.u-strasbg.fr/viz-bin/VizieR-5?-out.add=.&-source=V/117A/newcat&recno=13627. Retrieved 19 November 2008.
- ↑ Holmberg (2007). Record 13631, In: Geneva-Copenhagen Survey of Solar neighbourhood. http://vizier.u-strasbg.fr/viz-bin/VizieR-5?-out.add=.&-source=V/117A/newcat&recno=13631. Retrieved 19 November 2008.
- ↑ Cochran, Artie P. Hatzes, R. Paul Butler, Geoffrey W.Marcy (1997). "The Discovery of a Planetary Companion to 16 Cygni B". The Astrophysical Journal 483 (1): 457–63. doi:10.1086/304245. http://www.iop.org/EJ/article/0004-637X/483/1/457/35587.html.
- ↑ Butler; Wright, J. T.; Marcy, G. W.; Fischer, D. A.; Vogt, S. S.; Tinney, C. G.; Jones, H. R. A.; Carter, B. D. et al. (2006). "Catalog of Nearby Exoplanets". The Astrophysical Journal 646 (1): 505–22. doi:10.1086/504701. http://www.iop.org/EJ/article/0004-637X/646/1/505/64046.html.
- ↑ Wittenmyer; Endl, Michael; Cochran, William D.; Levison, Harold F. (2007). "Dynamical and Observational Constraints on Additional Planets in Highly Eccentric Planetary Systems". The Astronomical Journal 134 (3): 1276–84. doi:10.1086/520880. http://www.iop.org/EJ/article/1538-3881/134/3/1276/205882.html.
- ↑ 52.0 52.1 T. Blöcker; D. Schönberner (April 1991). "A 7 M⊙ AGB model sequence not complying with the core mass-luminosity relation". Astronomy and Astrophysics 244 (2): L43-6.
- ↑ "Brown dwarf, In: Wikipedia". San Francisco, California: Wikimedia Foundation, Inc. June 16, 2012. Retrieved 2012-07-11.
- ↑ 54.0 54.1 J. Davy Kirkpatrick; I. Neill Reid; James Liebert; Roc M. Cutri; Brant Nelson; Charles A. Beichman; Conard C. Dahn; David G. Monet et al. (10 July 1999). "Dwarfs Cooler than "M": The Definition of Spectral Type "L" Using Discoveries from the 2-Micron All-Sky Survey (2MASS)". The Astrophysical Journal 519 (2): 802-33. http://iopscience.iop.org/article/10.1086/307414/pdf. Retrieved 2017-04-29.
- ↑ 55.0 55.1 E. L. Martín (November 2012). "Exoplanet plenitude". Advances in Astronomy and Space Physics 2 (11): 109-13. http://aasp.kiev.ua/volume2/109-113-Martin.pdf. Retrieved 2013-12-23.
- ↑ D.J. Sahnow (1995-07-03). The Far Ultraviolet Spectroscopic Explorer Mission. http://fuse.pha.jhu.edu/papers/technical/aas95/aas95.html. Retrieved 2007-09-07.
- ↑ Mysterioso (26 July 2014). "The periodic table/Lithium". San Francisco, California: Wikimedia Foundation, Inc. Retrieved 2016-08-22.
{{cite web}}
:|author=
has generic name (help)