Jupiter

Jupiter is the largest planet in the Solar System and contains nearly 3/4 of all planetary matter.
With no solid surface, Jupiter is a gas and liquid filled giant. Its turbulent belts of clouds circulate parallel to the equator and often contain oval spots which are storm systems with the largest being easily twice the diameter of Earth. The great red spot has been observed for at least 300 years and rotates counter-clockwise with wind speeds of 270 miles per hour [430 km/hr].
Although observed and studied from Earth for centuries it wasn't until the mid 1970's that humans were able to get a closer look with the spacecraft Pioneer 10 and 11. The Voyager 1 and 2 spacecraft were launched with the specific purpose of collecting information and data on the Jovian worlds. In December 1995 the Galileo spacecraft entered into orbit and began it's long-term study of Jupiter and it's moons, a probe was also sent deep into the atmosphere of the gas giant.
Astronomical units
[edit | edit source]Notation: let the symbol indicate the radius of Jupiter.
Astrophysics
[edit | edit source]- Diameter: 142,900 km (11.2 x Earth's diameter)
- Length of Day: 9h 55m 30s
- Length of year: 11.867 years (4334.3 days)
- Average distance from the Sun: 5.2028 AU (7.783 x 108 km)
- Average orbital velocity: 13.06 km/s.
- Average Density: 1.34g/cm3
- Mass: 1.899 x 1027 kg (317.83 x Earth's Mass).
Jupiter has an equatorial radius of 71,492 ±4 km, a polar radius of 66,854 ±10 km, and a mean radius of 69,911 ± 6 km.[1]
Orbital characteristics (2002)
[edit | edit source](Epoch J2000, 17 May 2002):
- orbital radius: 778,330,000 km (5.20 AU) from Sun.
- aphelion: 815,700,000 km.
- perihelion: 740,900,000 km.
- orbital period: 4333 days.
Orbital characteristics (2004)
[edit | edit source](Epoch J2000, 20 September 2004 & 16 November 2004):
- aphelion: 816,620,000 km[2][3], 5.46 AU, 507,000,000 miles.
- perihelion: 740,520,000 km, 4.95 AU, 460,280,000 miles.
- semimajor: 778,300,000 km, 5.20336301 AU, 483,680,000 miles.
- eccentricity: 0.04839266.
- inclination: 1.30530°, (6.09° to Sun's equator).
- longitude of ascending node: 100.55615°.
- argument of perihelion: 14.75385°.
- orbital circ: 4.888 Tm, 32.675 AU.
- sidereal period: 4,332.589 day, 11.862 julian year (yr).
- synodic period: 398.88 day.
- avg speed: 13.07 km/s.
- max speed: 13.72 km/s.
- min speed: 12.44 km/s.
Orbital characteristics (2012)
[edit | edit source](Epoch J2000, 1 May 2012, 13 July 2006):[4][5]
- aphelion: 816,520,800 kilometres (5.458104 AU).
- perihelion: 740,573,600 kilometres (4.950429 AU).
- semimajor: 778,547,200 kilometres (5.204267 AU).
- eccentricity: 0.048775
- inclination: 1.305° to Ecliptic, 6.09° to Sun's equator, 0.32° to Invariable plane.[6]
- longitude of ascending node: 100.492°
- argument of perihelion: 275.066°
- mean anomaly: 18.818°
- period: 4,332.59 days, 11.8618 julian year (yr), 10,475.8 Jupiter solar days[7]
- synodic period: 398.88 days[2]
- avg speed: 13.07 km/s.[2]
Orbital characteristics (2017)
[edit | edit source](Epoch J2000, 12 May 2017, February 1994):[8]
- aphelion: 5.45492 AU (816.04 Gm)
- perihelion: 4.95029 AU (740.55 Gm)
- semimajor: 5.20260 AU (778.299 Gm)
- eccentricity: 0.048498
- inclination: 1.303° to ecliptic, 6.09° to Sun's equator, 0.32° to invariable plane (3 April 2009).[6]
- longitude of ascending node: 100.464°.
- argument of perihelion: 273.867°.
- mean anomaly: 20.020°.
- period: 11.8618 Julian year (yr), 4332.59 days, 10475.8 Jovian solar days[7]
- synodic period: 398.88 days[2]
- avg speed: 13.07 km/s[2]
Orbital characteristics (2022)
[edit | edit source](Epoch J2000, 18 May 2022, before 13 October 2017):[9]
- aphelion: 816.363 Gm (5.4570 AU).
- perihelion: 740.595 Gm (4.9506 AU).
- semi-major axis: 778.479 Gm (5.2038 AU).
- eccentricity: 0.0489.
- orbital period (sidereal): 11.862 yr (Julian year), 4,332.59 d, 10,476.8 Jovian solar days (before 13 August 2009).[7]
- orbital period (synodic): 398.88 d.
- average orbital speed: 13.07 km/s (8.12 mi/s).
- mean anomaly: 20.020° (February 1994).[10]
- inclination: 1.303° to ecliptic (February 1994),[10] 6.09° to Sun's equator (February 1994),[10] 0.32° to invariable plane (July 2012).[11]
- longitude of ascending node: 100.464°.
- time of perihelion: 21 January 2023 (before 7 September 2021).[12]
- argument of perihelion: 273.867° (February 1994).[10]
Entities
[edit | edit source]The entity Thor (also called Jupiter in some cultures) is assigned to throwing lightning bolts.
Objects
[edit | edit source]
"[T]he ancients’ religions and mythology speak for their knowledge of Uranus; the dynasty of gods had Uranus followed by Saturn, and the latter by Jupiter."[13]
"This false-color view of Jupiter [on the right] was taken by the Hubble Space Telescope in 2006. The red color traces high-altitude haze blankets in the polar regions, equatorial zone, the Great Red Spot, and a second red spot below and to the left of its larger cousin. The smaller red spot is approximately as wide as Earth."[14]
"NASA's Hubble Space Telescope is giving astronomers their most detailed view yet of a second red spot emerging on Jupiter. For the first time in history, astronomers have witnessed the birth of a new red spot on the giant planet, which is located half a billion miles away. The storm is roughly one-half the diameter of its bigger and legendary cousin, the Great Red Spot. Researchers suggest that the new spot may be related to a possible major climate change in Jupiter's atmosphere. These images were taken with Hubble's Advanced Camera for Surveys on April 8 and 16, 2006."[14]
Bands
[edit | edit source]
At the right is a significant observation of Jupiter in the H2O band using the Stockholm Infrared Camera (SIRCA) on the Nordic Observatory Telescope (NOT).
The image clearly shows that water vapor is plentiful in the Jovian atmosphere.
Meteors
[edit | edit source]


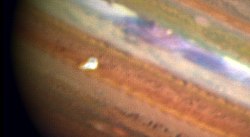

Jupiter has been called the Solar System's vacuum cleaner,[16] because of its immense gravity well and location near the inner Solar System. It receives the most frequent comet impacts of the Solar System's planets.[17]
A 1997 survey of historical astronomical drawings suggested that the astronomer Cassini may have recorded an impact scar in 1690. The survey determined eight other candidate observations had low or no possibilities of an impact.[18] A fireball was photographed by Voyager 1 during its Jupiter encounter in March 1979.[19] During the period July 16, 1994, to July 22, 1994, over 20 fragments from the comet Shoemaker–Levy 9 (SL9, formally designated D/1993 F2) collided with Jupiter's southern hemisphere, providing the first direct observation of a collision between two Solar System objects. This impact provided useful data on the composition of Jupiter's atmosphere.[20][21]
On July 19, 2009, an impact site was discovered at approximately 216 degrees longitude in System 2.[22][23] This impact left behind a black spot in Jupiter's atmosphere, similar in size to Oval BA. Infrared observation showed a bright spot where the impact took place, meaning the impact warmed up the lower atmosphere in the area near Jupiter's south pole.[24]
A fireball, smaller than the previous observed impacts, was detected on June 3, 2010, by Anthony Wesley, an amateur astronomer in Australia, and was later discovered to have been captured on video by another amateur astronomer in the Philippines.[25] Yet another fireball was seen on August 20, 2010.[26]
On September 10, 2012, another fireball was detected.[19][27]
The second image at right shows the atmospheric impact sites for the Comet Shoemaker-Levy 9 fragments. Spectroscopic studies revealed absorption lines in the Jovian spectrum due to diatomic sulfur (S2) and carbon disulfide (CS2), the first detection of either in Jupiter, and only the second detection of S2 in any astronomical object. Other molecules detected included ammonia (NH3) and hydrogen sulfide (H2S). The amount of sulfur implied by the quantities of these compounds was much greater than the amount that would be expected in a small cometary nucleus, showing that material from within Jupiter was being revealed.
"A false-color composite image [fourth down on the right] of Jupiter and its South Equatorial Belt shows an unusually bright spot, or outbreak, where winds are lofting particles to high altitudes in this image made from data obtained by the W.M. Keck telescope on Nov. 11, 2010."[28]
"The white clouds [in the fifth image down on the right], which get up to 50 miles (80 kilometers) wide or so, are high up in Jupiter's atmosphere — so high that they're very cold, and the material they shed is therefore almost certainly frozen."[29]
"It's snowing on Jupiter, and we're seeing how it works."[29]
"It's probably mostly ammonia ice, but there may be water ice mixed into it, so it's not exactly like the snow that we have [on Earth]. And I was using my imagination when I said it was snowing there — it could be hail."[29]
"This photo taken by NASA’s Juno spacecraft on May 19, 2017, at 5:50 UTC from an altitude of 5,500 miles (8,900 kilometers) shows high-flying white clouds composed of water ice and/or ammonia ice. In some areas, these clouds appear to form squall lines — narrow bands of high winds and storms associated with a cold front."[29]
Electrons
[edit | edit source]"Field-aligned equatorial electron beams [have been] observed within Jupiter’s middle magnetosphere. ... the Jupiter equatorial electron beams are spatially and/or temporally structured (down to <20 km at auroral altitudes, or less than several minutes), with regions of intense beams intermixed with regions absent of such beams."[30]
"Jovian electrons, both at Jupiter and in the interplanetary medium near Earth, have a very hard spectrum that varies as a power law with energy (see, e.g., Mewaldt et al. 1976). This spectral character is sufficiently distinct from the much softer solar and magnetospheric electron spectra that it has been used as a spectral filter to separate Jovian electrons from other sources ... A second Jovian electron characteristic is that such electrons in the interplanetary medium tend to consist of flux increases of several days duration which recur with 27 day periodicities ... A third feature of Jovian electrons at 1 AU is that the flux increases exhibit a long-term modulation of 13 months which is the synodic period of Jupiter as viewed from Earth".[31]
X-rays
[edit | edit source]

The "image of Jupiter [at right] shows concentrations of auroral X-rays near the north and south magnetic poles."[32] The Chandra X-ray Observatory accumulated X-ray counts from Jupiter for its entire 10-hour rotation on December 18, 2000. Note that X-rays from the entire globe of Jupiter are detected.
In the second at right is a diagram describing interaction with the local magnetic field. Jupiter's strong, rapidly rotating magnetic field (light blue lines in the figure) generates strong electric fields in the space around the planet. Charged particles (white dots), "trapped in Jupiter's magnetic field, are continually being accelerated (gold particles) down into the atmosphere above the polar regions, so auroras are almost always active on Jupiter. Electric voltages of about 10 million volts, and currents of 10 million amps - a hundred times greater than the most powerful lightning bolts - are required to explain the auroras at Jupiter's poles, which are a thousand times more powerful than those on Earth. On Earth, auroras are triggered by solar storms of energetic particles, which disturb Earth's magnetic field. As shown by the swept-back appearance in the illustration, gusts of particles from the Sun also distort Jupiter's magnetic field, and on occasion produce auroras."[33]
Ultraviolets
[edit | edit source]

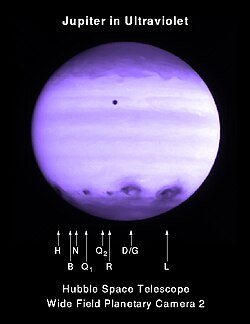
In astronomy, very hot objects preferentially emit UV radiation (see Wien's law). Because the ozone layer blocks many UV frequencies from reaching telescopes on the surface of the Earth, most UV observations are made from space.
At right is an ultraviolet image of aurora at Jupiter's north pole by the Hubble Space Telescope.
At left is a recent ulraviolet image of Jupiter showing the Great Red Spot and Red Spot Jr. on 11 January 2021.
"Experiments on the Voyager 1 and 2 spacecraft and observations made by the International Ultraviolet Explorer (IUE) have provided evidence for the existence of energetic particle precipitation into the upper atmosphere of Jupiter from the magnetosphere."[34]
The image at lower right shows Jupiter's atmosphere at a wavelength of 2550 Angstroms after many impacts by fragments of comet Shoemaker-Levy 9. The most recent impactor is fragment R which is below the center of Jupiter (third dark spot from the right). This photo was taken 3:55 EDT on July 21, about 2.5 hours after R's impact. A large dark patch from the impact of fragment H is visible rising on the morning (left) side. Proceeding to the right, other dark spots were caused by impacts of fragments Q1, R, D and G (now one large spot), and L, with L covering the largest area of any seen thus far. Small dark spots from B, N, and Q2 are visible with careful inspection of the image. The spots are very dark in the ultraviolet because a large quantity of dust is being deposited high in Jupiter's stratosphere, and the dust absorbs sunlight.
Visuals
[edit | edit source]
There is anecdotal evidence that people had seen the Galilean moons of Jupiter before telescopes were invented.[35]
Violets
[edit | edit source]
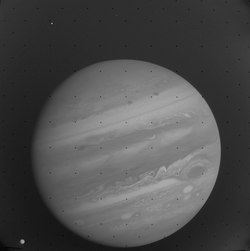

"This movie [at right] records an eruptive event in the southern hemisphere of Jupiter over a period of 8 Jupiter days. Prior to the event, an undistinguished oval cloud mass cruised through the turbulent atmosphere. The eruption occurs over a very short time at the very center of the cloud. The white eruptive material is swirled about by the internal wind patterns of the cloud. As a result of the eruption, the cloud then becomes a type of feature seen elsewhere on Jupiter known as "spaghetti bowls.""[36]
"As Voyager 2 approached Jupiter in 1979, it took images of the planet at regular intervals. This sequence is made from 8 images taken once every Jupiter rotation period (about 10 hours). These images were acquired in the Violet filter around May 6, 1979. The spacecraft was about 50 million kilometers from Jupiter at that time."[36]
At left is a "Voyager 1 image showing Jupiter with its satellite Io visible at lower left. Jupiter is 140,000 km in diameter and Io is 3600 km across. This image was taken with the narrow angle camera using the violet filter from a distance of 25 million km on 9 February 1979. North is at about 11:00 (Voyager 1, 15672.37)".[37]
"These images [at lower right] show the apparent edge (limb) of the planet Jupiter as seen through both the violet filter (top frame) and an infrared filter (756 nanometers, bottom frame) of the Solid State Imaging (CCD) system aboard NASA's Galileo spacecraft. North is to the top of the picture. A separate haze layer is clearly visible above the northern part of the limb."[38]
"This haze layer becomes less well defined to the south (bottom left). Such a detached haze layer has been seen previously on only one other body with a thick atmosphere: Saturn's satellite Titan. The haze layer cannot be lower in the atmosphere than a pressure of about 10 millibars (mbar), or about 40 kilometers (km) above the tropopause. (The tropopause, where the temperature stops decreasing with height, is at about 100 mbar, 20 km above the tops of the ammonia clouds.) There is some indication of streaks of slightly brighter and darker material running roughly north-south (parallel to the limb) on Jupiter's crescent."[38]
"The images, which show the limb between 60.5 degrees and 61.8 degrees North latitude (planetographic) and near 315 degrees West longitude, were obtained on December 20, 1996 Universal Time. The spacecraft was about 1,286,000 km (18.0 Jovian radii) from the limb of Jupiter and the resolution is about 13 kilometers per picture element."[38]
Blues
[edit | edit source]
The wide equatorial zone is visible in the center surrounded by two dark equatorial belts (SEB and NEB).
"The large grayish-blue [irregular] "hot spots" at the northern edge of the white Equatorial Zone change over the course of time as they march eastward across the planet."[39]
"The Great Red Spot shows its counterclockwise rotation, and the uneven distribution of its high haze is obvious. To the east (right) of the Red Spot, oval storms, like ball bearings, roll over and pass each other. Horizontal bands adjacent to each other move at different rates. Strings of small storms rotate around northern-hemisphere ovals."[39]
"Small, very bright features appear quickly and randomly in turbulent regions, candidates for lightning storms."[39]
"The smallest visible features at the equator are about 600 kilometers (about 370 miles) across."[39]
"The clip consists of 14 unevenly spaced timesteps, each a true color cylindrical projection of the complete circumference of Jupiter, from 60 degrees south to 60 degrees north. The maps are made by first assembling mosaics of six images taken by Cassini's narrow-angle camera in the same spectral filter over the course of one Jupiter rotation and, consequently, covering the whole planet. Three such global maps -- in red, green and blue filters -- are combined to make one color map showing Jupiter during one Jovian rotation. Fourteen such maps, spanning 24 Jovian rotations at uneven time intervals comprise the movie."[39]
The passage of time is accelerated by a factor of 600,000.
Yellows
[edit | edit source]The #8 yellow filter is used to show Mars's maria and Jupiter's belts.[40]
Oranges
[edit | edit source]
In the image at the top of the page, orange cloud bands are clearly visible on Jupiter.
"[O]range [is] the color of Jupiter"[41].
The orange and brown coloration in the clouds of Jupiter are caused by upwelling compounds that change color when they are exposed to ultraviolet light from the Sun. The exact makeup remains uncertain, but the substances are believed to be phosphorus, sulfur or possibly hydrocarbons.[42][43] These colorful compounds, known as chromophores, mix with the warmer, lower deck of clouds. The zones are formed when rising convection cells form crystallizing ammonia that masks out these lower clouds from view.[44]
"This image [on the right] of Jupiter is a composite of three color images taken on Nov. 16, 2010, by NASA's Infrared Telescope Facility. The particles lofted by the initial outbreak are easily identified in green as high altitude particles at the upper right, with a second outbreak to the lower left."[28]
"Earlier this year, one of Jupiter’s stripes went missing. The Southern Equatorial Band started to get lighter and paler, and eventually disappeared. Now, follow-up images from both professional and amateur astronomers are showing some activity in the area of the SEB, and scientists now believe the vanished dark stripe is making a comeback."[28]
“The reason Jupiter seemed to ‘lose’ this band – camouflaging itself among the surrounding white bands – is that the usual downwelling winds that are dry and keep the region clear of clouds died down. One of the things we were looking for in the infrared was evidence that the darker material emerging to the west of the bright spot was actually the start of clearing in the cloud deck, and that is precisely what we saw.”[45]
"This white cloud deck is made up of white ammonia ice. When the white clouds float at a higher altitude, they obscure the missing brown material, which floats at a lower altitude. Every few decades or so, the South Equatorial Belt turns completely white for perhaps one to three years, an event that has puzzled scientists for decades. This extreme change in appearance has only been seen with the South Equatorial Belt, making it unique to Jupiter and the entire solar system."[28]
"The white band wasn’t the only change on the big, gaseous planet. At the same time, Jupiter’s Great Red Spot became a darker red color."[28]
"The color of the spot – a giant storm on Jupiter that is three times the size of Earth and a century or more old – will likely brighten a bit again as the South Equatorial Belt makes its comeback."[45]
"The South Equatorial Belt underwent a slight brightening, known as a “fade,” just as NASA’s New Horizons spacecraft was flying by on its way to Pluto in 2007. Then there was a rapid “revival” of its usual dark color three to four months later. The last full fade and revival was a double-header event, starting with a fade in 1989, revival in 1990, then another fade and revival in 1993. Similar fades and revivals have been captured visually and photographically back to the early 20th century, and they are likely to be a long-term phenomenon in Jupiter’s atmosphere."[28]
Reds
[edit | edit source]
The Great Red Spot (GRS) is a persistent anticyclonic storm, 22° south of Jupiter's equator, which has lasted for at least 194 years and possibly longer than 359 years.[46][47] The storm is large enough to be visible through Earth-based telescopes. Its dimensions are 24–40,000 km west–to–east and 12–14,000 km south–to–north. The spot is large enough to contain two or three planets the size of Earth. At the start of 2004, the Great Red Spot had approximately half the longitudinal extent it had a century ago, when it was 40,000 km in diameter. The Great Red Spot's latitude has been stable for the duration of good observational records, typically varying by about a degree.
It is not known exactly what causes the Great Red Spot's reddish color. Theories supported by laboratory experiments suppose that the color may be caused by complex organic molecules, red phosphorus, or yet another sulfur compound. The Great Red Spot (GRS) varies greatly in hue, from almost brick-red to pale salmon, or even white. The reddest central region is slightly warmer than the surroundings, which is the first evidence that the Spot's color is affected by environmental factors.[48] The spot occasionally disappears from the visible spectrum, becoming evident only through the Red Spot Hollow, which is its niche in the South Equatorial Belt. The visibility of GRS is apparently coupled to the appearance of the SEB; when the belt is bright white, the spot tends to be dark, and when it is dark, the spot is usually light. The periods when the spot is dark or light occur at irregular intervals; as of 1997, during the preceding 50 years, the spot was darkest in the periods 1961–66, 1968–75, 1989–90, and 1992–93.[49]
"Jupiter’s most celebrated atmospheric beauty mark, the Great Red Spot (GRS), has been shrinking for years. When I was a kid in the ’60s peering through my Edmund 6-inch reflector, not only was the Spot decidedly red, but it was extremely easy to see. Back then it really did span three Earths."[50]
"In the 1880s the GRS resembled a huge blimp gliding high above white crystalline clouds of ammonia and spanned 40,000 km (25, 000 miles) across. You couldn’t miss it even in those small brass refractors that were the standard amateur observing gear back in the day. Nearly one hundred years later in 1979, the Spot’s north-south extent has remained virtually unchanged, but it’s girth had shrunk to 25,000 km (15,535 miles) or just shy of two Earth diameters. Recent work done by expert astrophotographer Damian Peach using the WINJUPOS program to precisely measure the GRS in high resolution photos over the past 10 years indicates a continued steady shrinkage:"[50] 2003 Feb – 18,420km (11,445 miles) 2005 Apr – 18,000km (11,184) 2010 Sep – 17,624km (10,951) 2013 Jan – 16,954km (10,534) 2013 Sep – 15,894km (9,876) 2013 Dec – 15,302km (9,508) = 1.2 Earth diameters.
"Not only has the Spot been shrinking, its rotation period has been speeding up. Older references give the period of one rotation at 6 days. John Rogers (British Astronomical Assn.) published a 2012 paper on the evolution of the GRS and discovered that between 2006 to 2012 – the same time as the Spot has been steadily shrinking – its rotation period has spun up to 4 days."[50]
"Rogers also estimated a max wind speed of 300 mph, up from about 250 mph in 2006. Despite its smaller girth, this Jovian hurricane’s winds pack more punch than ever. Even more fascinating, the Great Red Spot may have even disappeared altogether from 1713 to 1830 before reappearing in 1831 as a long, pale “hollow”. According to Rogers, no observations or sketches of that era mention it. Surely something so prominent wouldn’t be missed. This begs the question of what happened in 1831. Was the “hollow” the genesis of a brand new Red Spot unrelated to the one first seen by astronomer Giovanni Cassini in 1665? Or was it the resurgence of Cassini’s Spot?"[50]
Infrareds
[edit | edit source]







"Spectra from the Voyager I IRIS experiment confirm the existence of enhanced infrared emission near Jupiter's north magnetic pole in March 1979."[51] "Some species previously detected on Jupiter, including CH3D, C2H2, and C2H6, have been observed again near the pole. Newly discovered species, not previously observed on Jupiter, include C2H4, C3H4, and C6H6. All of these species except CH3D appear to have enhanced abundances at the north polar region with respect to midlatitudes."[51]
The image at third lower right is "of Jupiter taken in infrared light on the night of [August 17, 2008,] with the Multi-Conjugate Adaptive Optics Demonstrator (MAD) prototype instrument mounted on ESO's Very Large Telescope. This false color photo is the combination of a series of images taken over a time span of about 20 minutes, through three different filters (2, 2.14, and 2.16 microns). The image sharpening obtained is about 90 milli-arcseconds across the whole planetary disc, a real record on similar images taken from the ground. This corresponds to seeing details about 186 miles wide on the surface of the giant planet. The great red spot is not visible in this image as it was on the other side of the planet during the observations. The observations were done at infrared wavelengths where absorption due to hydrogen and methane is strong. This explains why the colors are different from how we usually see Jupiter in visible-light. This absorption means that light can be reflected back only from high-altitude hazes, and not from deeper clouds. These hazes lie in the very stable upper part of Jupiter's troposphere, where pressures are between 0.15 and 0.3 bar. Mixing is weak within this stable region, so tiny haze particles can survive for days to years, depending on their size and fall speed. Additionally, near the planet's poles, a higher stratospheric haze (light blue regions) is generated by interactions with particles trapped in Jupiter's intense magnetic field."[52]
The image at the top shows Jupiter in the near infrared. "Five spots -- one colored white, one blue, and three black are scattered across the upper half of the planet. Closer inspection by NASA's Hubble Space Telescope reveals that these spots are actually a rare alignment of three of Jupiter's largest moons -- Io, Ganymede, and Callisto -- across the planet's face. In this image, the telltale signatures of this alignment are the shadows [the three black circles] cast by the moons. Io's shadow is located just above center and to the left; Ganymede's on the planet's left edge; and Callisto's near the right edge. Only two of the moons, however, are visible in this image. Io is the white circle in the center of the image, and Ganymede is the blue circle at upper right. Callisto is out of the image and to the right. ... Jupiter appears in pastel colors in this photo because the observation was taken in near-infrared light. Astronomers combined images taken in three near-infrared wavelengths to make this color image. The photo shows sunlight reflected from Jupiter's clouds. In the near infrared, methane gas in Jupiter's atmosphere limits the penetration of sunlight, which causes clouds to appear in different colors depending on their altitude. Studying clouds in near-infrared light is very useful for scientists studying the layers of clouds that make up Jupiter's atmosphere. Yellow colors indicate high clouds; red colors lower clouds; and blue colors even lower clouds in Jupiter's atmosphere. The green color near the poles comes from a thin haze very high in the atmosphere. Ganymede's blue color comes from the absorption of water ice on its surface at longer wavelengths. Io's white color is from light reflected off bright sulfur compounds on the satellite's surface. ... In viewing this rare alignment, astronomers also tested a new imaging technique. To increase the sharpness of the near-infrared camera images, astronomers speeded up Hubble's tracking system so that Jupiter traveled through the telescope's field of view much faster than normal. This technique allowed scientists to take rapid-fire snapshots of the planet and its moons. They then combined the images into one single picture to show more details of the planet and its moons."[53]
On July 19, 2009, a new black spot about the size of Earth was discovered in Jupiter's southern hemisphere by an amateur astronomer. Thermal infrared analysis showed it was warm and spectroscopic methods detected ammonia. JPL scientists confirmed that another impact event on Jupiter had occurred, probably a small undiscovered comet or other icy body.[54][55][56]
"These images [at right] show the distribution of the organic molecule acetylene at the north and south poles of Jupiter, based on data obtained by NASA's Cassini spacecraft in early January 2001. It is the highest-resolution map of acetylene to date on Jupiter. The enhanced emission results both from the warmer temperatures in the auroral hot spots, and probably also from an enhanced abundance in these regions. The detection helps scientists understand the chemical interactions between sunlight and molecules in Jupiter's stratosphere."[57]
The sixth image down on the right shows Jupiter in an infrared band where the Great Red Spot (on the lower left) is almost unseen.
The image down on the left shows global temperatures for Jupiter ranging from dark 500 K to white 1,000 K as determined by infrared astronomy measurements of the median Jovian H+
3 temperatures.
"Long black-and-white dashed lines show Jupiter’s main auroral oval, short black-and-white dashed lines correspond to the magnetic footprint of Io, and the single thick black line corresponds to the magnetic footprint of Amalthea [...]. A visible computer-generated globe of Jupiter based on Hubble Space Telescope imagery is shown underneath the H+
3 temperature projection."[58]
Compare the Juno image at left with the view of Jupiter on the lowest right created from data captured on 11 January 2017 with the Near-InfraRed Imager (NIRI) instrument at Gemini North in Hawaiʻi, the northern member of the international Gemini Observatory, a Program of NSF’s NOIRLab. It is actually a mosaic of individual frames that were combined to produce a global portrait of the planet. In the image warmer areas appear bright, including four large hot spots that appear in a row just north of the equator. South of the equator, the oval-shaped and cloud-covered Great Red Spot appears dark.
Submillimeters
[edit | edit source]"[F]or wavelengths between 0.35 and 0.45 mm ... the radiances can be matched by models which include NH3 ice particles which are between 30 and 100 µm in size, regardless of the scale height characterizing the cloud."[59]
Microwaves
[edit | edit source]
"We in essence created a three-dimensional picture of ammonia gas in Jupiter’s atmosphere, which reveals upward and downward motions within the turbulent atmosphere."[60]
"The radio map shows ammonia-rich gases rising into and forming the upper cloud layers: an ammonium hydrosulfide cloud at a temperature near 200 Kelvin (minus 100 degrees Fahrenheit) and an ammonia-ice cloud in the approximately 160 Kelvin cold air (minus 170 degrees Fahrenheit). These clouds are easily seen from Earth by optical telescopes."[61]
"Conversely, the radio maps show ammonia-poor air sinking into the planet, similar to how dry air descends from above the cloud layers on Earth."[61]
"The map also shows that hotspots – so-called because they appear bright in radio and thermal infrared images – are ammonia-poor regions that encircle the planet like a belt just north of the equator. Between these hotspots are ammonia-rich upwellings that bring ammonia from deeper in the planet."[61]
"With radio, we can peer through the clouds and see that those hotspots are interleaved with plumes of ammonia rising from deep in the planet, tracing the vertical undulations of an equatorial wave system."[62]
"We now see high ammonia levels like those detected by Galileo from over 100 kilometers deep, where the pressure is about eight times Earth’s atmospheric pressure, all the way up to the cloud condensation levels."[60]
"We now see fine structure in the 12 to 18 gigahertz band, much like we see in the visible, especially near the Great Red Spot, where we see a lot of little curly features. Those trace really complex upwelling and downwelling motions there."[62]
"Jupiter’s rotation once every 10 hours usually blurs radio maps, because these maps take many hours to observe. But we have developed a technique to prevent this and so avoid confusing together the upwelling and downwelling ammonia flows, which had led to the earlier underestimate."[63]
"The pink glow surrounding the planet [in the image on the right] is synchrotron radiation produced by spiraling electrons trapped in Jupiter’s magnetic field. Banded details on the planet’s disk probe depths of 30-90 km below the clouds. This image is averaged from 10 hours of VLA data, so the fine details seen in the other maps are smeared here by the planet’s rotation."[60]
Radios
[edit | edit source]
In 1955, Bernard Burke and Kenneth Franklin detected bursts of radio signals coming from Jupiter at 22.2 MHz.[64] The period of these bursts matched the rotation of the planet, and they were also able to use this information to refine the rotation rate. Radio bursts from Jupiter were found to come in two forms: long bursts (or L-bursts) lasting up to several seconds, and short bursts (or S-bursts) that had a duration of less than a hundredth of a second.[65]
Forms of decametric radio signals from Jupiter:
- bursts (with a wavelength of tens of meters) vary with the rotation of Jupiter, and are influenced by interaction of Io with Jupiter's magnetic field.[66]
- emission (with wavelengths measured in centimeters) was first observed by Frank Drake and Hein Hvatum in 1959.[64] The origin of this signal was from a torus-shaped belt around Jupiter's equator. This signal is caused by cyclotron radiation from electrons that are accelerated in Jupiter's magnetic field.[67]
Between September and November 23, 1963, Jupiter is detected by radar astronomy.[68]
"The dense atmosphere makes a penetration to a hard surface (if indeed one exists at all) very unlikely. In fact, the JPL results imply a correlation of the echo with Jupiter ... which corresponds to the upper (visible) atmosphere. ... Further observations will be needed to clarify the current uncertainties surrounding radar observations of Jupiter."[68]
"Although in 1963 some claimed to have detected echoes from Jupiter, these were quite weak and have not been verified by later experiments."[69]
"A search for radar echoes from Jupiter at 430 MHz during the oppositions of 1964 and 1965 failed to yield positive results, despite a sensitivity several orders of magnitude better than employed by other groups in earlier (1963) attempts at higher frequencies. ... [I]t might be suspected that meteorological disturbances of a random nature were involved, and that the echoes might be returned only in exceptional circumstances. Further support for this point of view may be gleaned from the fact that JPL found positive results for only 1 (centered at 32° System I longitude) of the 8 longitude regions investigated in 1963 (Goldstein 1964) and, in fact, had no success during their observations in 1964 (see comment by Goldstein following Dyce 1965)."[70]
"This VLA image of Jupiter [at right] doesn't look like a planetary disk at all. Most of the radio emission is synchrotron radiation from electrons in Jupiter's magnetic field."[71]
Poles
[edit | edit source]



"The familiar banded appearance of Jupiter gradually gives way to a more mottled appearance closer to the north pole in this true color image [on the right] taken in 2000 by NASA's Cassini spacecraft."[72]
"The intricate structures seen in the polar region are clouds of different chemical composition, height and thickness. Clouds are organized by winds, and the mottled appearance in the polar regions suggests more vortex-type motion and winds of less vigor at higher latitudes."[72]
"One possible contributor is that the horizontal component of the Coriolis force, which arises from the planet's rotation and is responsible for curving the trajectories of ocean currents and winds on Earth, has its greatest effect at high latitudes and vanishes at the equator. This tends to create small, intense vortices at high latitudes on Jupiter. Another possibility may lie in that fact that Jupiter overall emits nearly as much of its own heat as it absorbs from the Sun, and this internal heat flux is very likely greater at the poles. This condition could lead to enhanced convection at the poles and more vortex-type structures."[72]
"The resolution here is 114 kilometers (71 miles) per pixel. This contrast-enhanced, edge-sharpened frame was composited from images take at different wavelengths with Cassini's narrow-angle camera, from a distance of 19 million kilometers (11.8 million miles). The spacecraft was in almost a direct line between the Sun and Jupiter, so the solar illumination on Jupiter is almost full phase."[72]
"These color maps [second down on the right] of Jupiter were constructed from images taken by the narrow-angle camera onboard NASA's Cassini spacecraft on Dec. 11 and 12, 2000, as the spacecraft neared Jupiter during its flyby of the giant planet. Cassini was on its way to Saturn. They are the most detailed global color maps of Jupiter ever produced. The smallest visible features are about 120 kilometers (75 miles) across."[73]
"The maps are composed of 36 images: a pair of images covering Jupiter's northern and southern hemispheres was acquired in two colors every hour for nine hours as Jupiter rotated beneath the spacecraft. Although the raw images are in just two colors, 750 nanometers (near-infrared) and 451 nanometers (blue), the map's colors are close to those the human eye would see when gazing at Jupiter."[73]
"The maps show a variety of colorful cloud features, including parallel reddish-brown and white bands, the Great Red Spot, multi-lobed chaotic regions, white ovals and many small vortices. Many clouds appear in streaks and waves due to continual stretching and folding by Jupiter's winds and turbulence. The bluish-gray features along the north edge of the central bright band are equatorial "hot spots," meteorological systems such as the one entered by NASA's Galileo probe. Small bright spots within the orange band north of the equator are lightning-bearing thunderstorms. The polar regions are less clearly visible because Cassini viewed them at an angle and through thicker atmospheric haze (such as the whitish material in the south polar map) [third down on the right]."[73]
"This image [on the left] shows Jupiter's south pole, as seen by NASA's Juno spacecraft from an altitude of 32,000 miles (52,000 kilometers). The oval features are cyclones, up to 600 miles (1,000 kilometers) in diameter. Multiple images taken with the JunoCam instrument on three separate orbits were combined to show all areas in daylight, enhanced color, and stereographic projection."[74]
Planetary astronomy
[edit | edit source]

"Jupiter takes 12 years to make one trip around the Sun. These 12 images [on the right] were taken between 2003 and 2015. At far left we see Jupiter in 2003, and the years proceed counterclockwise. The 2015 view is immediately above 2003."[75]
"Jupiter’s axial tilt is just 3° or nearly straight up and down, so seasons don’t exist. One part of the Jovian year is much the same as another. Still, as you can plainly see, the solar system’s biggest planet has plenty of weather."[76]
"Just look at the Great Red Spot or GRS. Through about 2008, it’s relatively large and pale but suddenly darkens in 2010 at the same time the South Equatorial Cloud Belt (the wide stripe of clouds above the Spot) disappears. If you look closely at the Spot from year to year, you’ll see another big change — it’s shrinking! The GRS has been dwindling for several decades, but it’s amazing how obvious the difference is in only a dozen years."[76]
"The planet gives off 1.6 times as much energy as it get from the Sun."[76]
"Fun to think that the light we see from Jupiter is reflected sunlight, but if we could view it with heat-sensing, infrared eyes, it would glow like an ember."[76]
"Images [second down on the right] in the visible-light and infrared parts of the spectrum highlight the massive changes roiling the atmosphere of Jupiter. In the visible-light images on the left that were obtained by amateur astronomers, Jupiter can be seen "losing" a brown-colored belt south of the equator called the South Equatorial Belt (SEB) from 2009 to 2010. This belt returned in 2011 and was still present in 2012. From 2011 to January 2012, a belt north of the equator known as the North Equatorial Belt (NEB) can be seen to be thinning out. In 2011, it whitened to an extent not seen in over a century. In March of 2012, after the last picture in this series was taken, the northern belt began to darken again."[77]
"Scientists compared the visible-light data to data obtained in infrared wavelengths (middle and right columns), which show progressively deeper levels in the Jovian atmosphere. The infrared images were obtained from NASA's Infrared Telescope Facility on Mauna Kea, Hawaii, except for the 2011 image in the 8.7-micron wavelength (right column, third from the top), which was taken by the Subaru Telescope, also in Mauna Kea, Hawaii. Those data showed a thickening of the deeper cloud decks in the northern belt during that time, and a partial thickening of the upper cloud deck. The South Equatorial Belt saw both levels of clouds thicken and then clear up. The infrared data also resolved brown elongated features in the whitened area of the North Equatorial Belt known as "brown barges" as distinct features and revealed them to be regions clearer of clouds and probably characterized by downwelling, dry air."[77]
"Also visible in the infrared observations are a series of blue-gray features that are the clearest and driest regions on the planet and show up as apparent hotspots in the infrared view because they reveal the radiation emerging from a very deep layer of Jupiter's atmosphere. Those hotspots disappeared from 2010 to 2011, but had reestablished themselves by June of this year, coincident with the whitening and re-darkening of the North Equatorial Belt."[77]
Sun-Jupiter binary
[edit | edit source]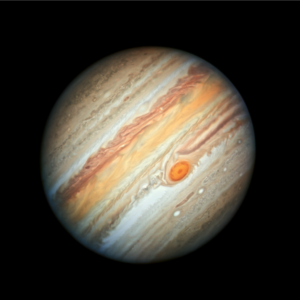


The Sun-Jupiter binary may serve to establish an upper limit for interstellar cometary capture when three bodies are extremely unequal in mass, such as the Sun, Jupiter, and a third body (potential comet) at a large distance from the binary.[78] The basic problem with a capture scenario even from passage through “a cloud of some 10 million years, or from a medium enveloping the solar system, is the low relative velocity [~0.5 km s-1] required between the solar system and the cometary medium.”[79] The capture of interstellar comets by Saturn, Uranus, and Neptune together cause about as many captures as Jupiter alone.[79]
In a mechanism of chaos assisted capture (CAC), particles such as comets or those of sizes in the range of the irregular moons of Jupiter become entangled in chaotic layers which temporarily “extend the lifetimes of [these] particles within the Hill sphere, thereby providing the breathing space necessary for relatively weak dissipative forces (eg gas-drag) to effect permanent capture.”[80] These objects of the Sun-Jupiter binary system may localize near Jupiter and become satellites, specifically the irregular moons.[80]
"This new Hubble Space Telescope view of Jupiter, taken on June 27, 2019, [image on the right] reveals the giant planet's trademark Great Red Spot, and a more intense color palette in the clouds swirling in Jupiter's turbulent atmosphere than seen in previous years."[10]
"Hubble's Wide Field Camera 3 observed Jupiter when the planet was 400 million miles from Earth, when Jupiter was near "opposition" or almost directly opposite the Sun in the sky."[10]
"At the time of observation, Jupiter was 4.30AU (400 million miles or 645 million kilometers) from Earth. The semi-major axis of Jupiter's orbit about the Sun is 5.2 astronomical units (483 million miles or 778 million km)."[10]
"The new image was taken in visible light as part of the Outer Planets Atmospheres Legacy program, or OPAL. The program provides yearly Hubble global views of the outer planets to look for changes in their storms, winds, and clouds."[10]
"These images are a composite of separate exposures acquired by the WFC3 instrument on the Hubble Space Telescope. Several filters were used to sample narrow wavelength ranges. The color results from assigning different hues (colors) to each monochromatic (grayscale) image associated with an individual filter. In this case, the assigned colors are: Blue: F395N (395 nm); Green: F502N (502 nm); and Red: F631N (631 nm)."[10]
"A worm-shaped feature located below the Great Red Spot is a cyclone, a vortex around a low-pressure area with winds spinning in the opposite direction from the Red Spot. Researchers have observed cyclones with a wide variety of different appearances across the planet. The two white oval-shaped features are anticyclones, like small versions of the Great Red Spot."[10]
The "color of the wide band at the equator [the] bright orange [...] may be a sign that deeper clouds are starting to clear out, emphasizing red particles in the overlying haze."[10]
"The Great Red Spot is a towering structure shaped like a wedding cake, whose upper haze layer extends more than 3 miles (5 kilometers) higher than clouds in other areas. The gigantic structure, with a diameter slightly larger than Earth's, is a high-pressure wind system called an anticyclone that has been slowly downsizing since the 1800s. The reason for this change in size is still unknown."[10]
"On the opposite side of the planet, the band of deep red color northeast of the Great Red Spot and the bright white band to the southeast of it become much fainter. The swirling filaments seen around the outer edge of the red super storm are high-altitude clouds that are being pulled in and around it."[10]
"All of Jupiter's colorful cloud bands in this image are confined to the north and south by jet streams that remain constant, even when the bands change color. The bands are all separated by winds that can reach speeds of up to 400 miles (644 kilometers) per hour."[10]
"Among the most striking features in the image are the rich colors of the clouds moving toward the Great Red Spot, a storm rolling counterclockwise between two bands of clouds. These two cloud bands, above and below the Great Red Spot, are moving in opposite directions. The red band above and to the right (northeast) of the Great Red Spot contains clouds moving westward and around the north of the giant tempest. The white clouds to the left (southwest) of the storm are moving eastward to the south of the spot."[10]
"The bands are created by differences in the thickness and height of the ammonia ice clouds. The colorful bands, which flow in opposite directions at various latitudes, result from different atmospheric pressures. Lighter bands rise higher and have thicker clouds than the darker bands."[10]
Each of the three images of Jupiter on the right were taken at different oppositions.
Coronal clouds
[edit | edit source]Because of an eccentricity of 0.048, the distance from Jupiter and the Sun varies by 75 million km between perihelion and aphelion, or the nearest and most distant points of the planet along the orbital path respectively.
"Favorable oppositions occur when Jupiter is passing through perihelion, an event that occurs once per orbit. As Jupiter approached perihelion in March 2011, there was a favorable opposition in September 2010.[81]
It's orbital period is 4,332.59 d (11.8618 y).
"It is shown that starting with the alignment of Venus with Jupiter at perihelion position, these two planets will perfectly align at Jupiter's perihelion after every 23.7236 years".[82]
"The tidal forces hypothesis for solar cycles has been proposed by Wood (1972) and others. Table 2 below shows the relative tidal forces of the planets on the sun. Jupiter, Venus, Earth and Mercury are called the "tidal planets" because they are the most significant. According to Wood, the especially good alignments of J-V-E with the sun which occur about every 11 years are the cause of the sunspot cycle. He has shown that the sunspot cycle is synchronous with the alignments, and J. Schove's data for 1500 year of sunspot maxima supports the 11.07 year J-V-E period average."[83]
"Both the 11.86 year Jupiter tropical period (time between perihelion's or closest approaches to the sun and the 9.93 year J-S alignment periods are found in sunspot spectral analysis. Unfortunately direct calculations of the tidal forces of all planets does not support the occurrence of the dominant 11.07 year cycle. Instead, the 11.86 year period of Jupiter's perihelion dominates the results. This has caused problems for several researchers in this field."[83]
The coronal cloud around Jupiter is exactly opposite to that around the Sun. At the Sun there are polar coronal holes, whereas at Jupiter the coronal cloud is most prevalent over the magnetic poles.
Jupiter perihelions
[edit | edit source]Jupiter's orbital period is 4,332.59 d (11.8618 y). Jupiter's perihelion and aphelion from 1900-2200 with approximate numbers that are somewhat different from the correct values.[84]
Solar cycle 24 began on 4 January 2008,[85] with minimal activity until early 2010.[86][87] The cycle featured a "double-peaked" solar maximum. The first peak reached 99 in 2011 and the second in early 2014 at 101.[88] Cycle 24 ended in December 2019 after 11.0 years.[89]
"To identify temporal variations of the characteristics of Jupiter’s cloud layer, we take into account the geometric modulation caused by the rotation of the planet and planetary orbital motion. Inclination of the rotation axis to the orbital plane of Jupiter is 3.13°, and the angle between the magnetic axis and the rotation axis is β ≈ 10°. Therefore, over a Jovian year, the jovicentric magnetic declination of the Earth φm varies from –13.13° to +13.13°, and the subsolar point on Jupiter’s magnetosphere is shifted by 26.26° per orbital period. In this connection, variations of the Earth’s jovimagnetic latitude on Jupiter will have a prevailing influence in the solar-driven changes of ref lective properties of the cloud cover and overcloud haze on Jupiter. Because of the orbit eccentricity (e = 0.048450), the northern hemisphere receives 21% greater solar energy inf low to the atmosphere, because Jupiter is at perihelion near the time of the summer solstice. The results of our studies have shown that the brightness ratio Aj of northern to southern tropical and temperate regions is an evident factor of photometric activity of Jupiter’s atmospheric processes. The analysis of observational data for the period from 1962 to 2015 reveals the existence of cyclic variations of the activity factor Aj of the planetary hemispheres with a period of 11.86 years, which allows us to talk about the seasonal rearrangement of Jupiter’s atmosphere."[90]
Aphelion: 447.
Perihelion: 453.
Aphelion: 459.
Perihelion: 465.
Aphelion: 471.
Perihelion: 477.
Aphelion: 483.
Perihelion: 489.
Aphelion: 495.
Perihelion: 501.
Aphelion: 507.
Perihelion: 513.
Aphelion: 519.
Perihelion: 525.
Aphelion: 531.
Perihelion: 537.
Aphelion: 543.
Perihelion: 549.
Aphelion: 555.
Perihelion: 561.
Aphelion: 567.
Perihelion: 573.
Aphelion: 579.
Perihelion: 585.
Aphelion: 591.
Perihelion: 597.
Aphelion: 603.
Perihelion: 609.
Aphelion: 615.
Perihelion: 621.
Aphelion: 627.
Perihelion: 633.
Aphelion: 639.
Perihelion: 645.
Aphelion: 651.
Perihelion: 657.
Aphelion: 663.
Perihelion: 669.
Aphelion: 675.
Perihelion: 681.
Aphelion: 687.
Perihelion: 693.
Aphelion: 699.
Perihelion: 705.
Aphelion: 711.
Perihelion: 717.
Aphelion: 723.
Perihelion: 729.
Aphelion: 735.
Perihelion: 741.
Aphelion: 747.
Perihelion: 753.
Aphelion: 759.
Perihelion: 765.
Aphelion: 771.
Perihelion: 777.
Aphelion: 783.
Perihelion: 789.
Aphelion: 795.
Perihelion: 801.
Aphelion: 807.
Perihelion: 813.
Aphelion: 819.
Perihelion: 825.
Aphelion: 831.
Perihelion: 837.
Aphelion: 843.
Perihelion: 849.
Aphelion: 855.
Perihelion: 861.
Aphelion: 867.
Perihelion: 873.
Aphelion: 879.
Perihelion: 885.
Aphelion: 891.
Perihelion: 897.
Aphelion: 903.
Perihelion: 909.
Aphelion: 915.
Perihelion: 921.
Aphelion: 927.
Perihelion: 933.
Aphelion: 939.
Perihelion: 945.
Aphelion: 951.
Perihelion: 957.
Aphelion: 963.
Perihelion: 969.
Aphelion: 975.
Perihelion: 981.
Aphelion: 987.
Perihelion: 993.
Aphelion: 999.
Perihelion: 1005.
Aphelion: 1011.
Perihelion: 1017.
Aphelion: 1023.
Perihelion: 1029.
Aphelion: 1035.
Perihelion: 1041.
Aphelion: 1047.
Perihelion: 1053.
Aphelion: 1059.
Perihelion: 1065.
Aphelion: 1071.
Perihelion: 1077.
Aphelion: 1083.
Perihelion: 1089.
Aphelion: 1095.
Perihelion: 1101.
Aphelion: 1107.
Perihelion: 1113.
Aphelion: 1119.
Perihelion: 1125.
Aphelion: 1131.
Perihelion: 1137.
Aphelion: 1143.
Perihelion: 1149.
Aphelion: 1155.
Perihelion: 1161.
Aphelion: 1167.
Perihelion: 1173.
Aphelion: 1179.
Perihelion: 1185.
Aphelion: 1191.
Perihelion: 1197.
Aphelion: 1203.
Perihelion: 1219.
Aphelion: 1225.
Perihelion: 1231.
Aphelion: 1237.
Perihelion: 1243.
Aphelion: 1249.
Perihelion: 1255.
Aphelion: 1261.
Perihelion: 1267.
Aphelion: 1273.
Perihelion: 1279.
Aphelion: 1285.
Perihelion: 1291.
Aphelion: 1297.
Perihelion: 1303.
Aphelion: 1309.
Perihelion: 1315.
Aphelion: 1321.
Perihelion: 1327.
Aphelion: 1333.
Perihelion: 1339.
Aphelion: 1345.
Perihelion: 1351.
Aphelion: 1357.
Perihelion: 1363.
Aphelion: 1369.
Perihelion: 1375.
Aphelion: 1381.
Perihelion: 1387.
Aphelion: 1393.
Perihelion: 1399.
Aphelion: 1405.
Perihelion: 1411.
Aphelion: 1417.
Perihelion: 1423.
Aphelion: 1429.
Perihelion: 1435.
Aphelion: 1441.
Perihelion: 1447.
Aphelion: 1453.
Perihelion: 1459.
Aphelion: 1465.
Perihelion: 1471.
Aphelion: 1477.
Perihelion: 1483.
Aphelion: 1489.
Perihelion: 1495.
Aphelion: 1501.
Perihelion: 1507.
Aphelion: 1513.
Perihelion: 1519.
Aphelion: 1525.
Perihelion: 1531.
Aphelion: 1537.
Perihelion: 1543.
Aphelion: 1549.
Perihelion: 1555.
Aphelion: 1561.
Perihelion: 1567.
Aphelion: 1573.
Perihelion: 1579.
Aphelion: 1585.
Perihelion: 1591.
Aphelion: 1597.
Perihelion: 1603.
Aphelion: 1609.
Perihelion: 1615.
Aphelion: 1621.
Perihelion: 1627.
Aphelion: 1633.
Perihelion: 1639.
Aphelion: 1645.
Perihelion: 1651.
Aphelion: 1657.
Perihelion: 1663.
Aphelion: 1669.
Perihelion: 1675.
Aphelion: 1681.
Perihelion: 1687.
Aphelion: 1693.
Perihelion: 1699.
Aphelion: 1705.
Perihelion: 1711.
Aphelion: 1717.
Perihelion: 1723.
Aphelion: 1729.
Perihelion: 1735.
Aphelion: 1741.
Perihelion: 1747.
Aphelion: 1753.
Perihelion: 1759.
Aphelion: 1765.
Perihelion: 1771.
Aphelion: 1777.
Perihelion: 1783.
Aphelion: 1789.
Perihelion: 1795.
Aphelion: 1801.
Perihelion: 1807.
Aphelion: 1813.
Perihelion: 1819.
Aphelion: 1825.
Perihelion: 1831.
Aphelion: 1837.
Perihelion: 1843.
Aphelion: 1849.
Perihelion: 1855.
Aphelion: 1861.
Perihelion: 1867.
Aphelion: 1873.
Perihelion: 1879.
Aphelion: 1885.
Perihelion: 1891.
Aphelion: 1897.
Perihelion | Aphelion | ||||
---|---|---|---|---|---|
Distance from Sun | Distance from Sun | ||||
Date | AU | mill. km | Date | AU | mill. km |
1904 June 1 | 4.95166 | 740.758 | 1910 May 10 | 5.45355 | 815.840 |
1916 April 18 | 4.95262 | 740.902 | 1922 April 5 | 5.45416 | 815.930 |
1928 March 15 | 4.95127 | 740.700 | 1934 February 19 | 5.45523 | 816.090 |
1940 January 23 | 4.94945 | 740.427 | 1945 December 19 | 5.45671 | 816.312 |
1951 November 21 | 4.94840 | 740.271 | 1957 October 23 | 5.45640 | 816.266 |
1963 September 26 | 4.95133 | 740.708 | 1969 September 3 | 5.45374 | 815.868 |
1975 August 12 | 4.95265 | 740.906 | 1981 July 28 | 5.45352 | 815.835 |
1987 July 10 | 4.95241 | 740.870 | 1993 June 15 | 5.45429 | 815.950 |
1999 May 20 | 4.95046 | 740.579 | 2005 April 15 | 5.45652 | 816.283 |
2011 March 17 | 4.94838 | 740.266 | 2017 February 17 | 5.45651 | 816.282 |
2023 January 20 | 4.95100 | 740.659 | 2028 December 28 | 5.45387 | 815.887 |
2034 December 5 | 4.95268 | 740.911 | 2040 November 18 | 5.45294 | 815.748 |
2046 November 1 | 4.95341 | 741.020 | 2052 October 7 | 5.45335 | 815.809 |
2058 September 13 | 4.95139 | 740.718 | 2064 August 9 | 5.45639 | 816.264 |
2070 July 11 | 4.94824 | 740.247 | 2076 June 13 | 5.45670 | 816.311 |
2082 May 15 | 4.95052 | 740.587 | 2088 April 21 | 5.45407 | 815.917 |
2094 March 29 | 4.95250 | 740.884 | 2100 March 11 | 5.45255 | 815.690 |
2106 February 23 | 4.95413 | 741.128 | 2112 January 31 | 5.45260 | 815.697 |
2118 January 6 | 4.95214 | 740.829 | 2123 December 4 | 5.45641 | 816.267 |
2129 November 5 | 4.94790 | 740.195 | 2135 October 8 | 5.45701 | 816.357 |
2141 September 7 | 4.94978 | 740.476 | 2147 August 15 | 5.45446 | 815.976 |
2153 July 22 | 4.95204 | 740.815 | 2159 July 2 | 5.45256 | 815.692 |
2165 June 15 | 4.95441 | 741.169 | 2171 May 23 | 5.45219 | 815.636 |
2177 April 29 | 4.95249 | 740.882 | 2183 March 28 | 5.45663 | 816.301 |
2189 February 27 | 4.94732 | 740.109 | 2195 January 31 | 5.45752 | 816.433 |
2200 December 31 | 4.94882 | 740.332 | 2206 December 8 | 5.45509 | 816.070.[84] |
Perihelion: March 2011. "between 2001 and 2005, towards aphelion in March 2005, and then increasing between 2007 and 2010, towards perihelion in March 2011."[91] "The orbital excitation is driven by the fast change of the normal oscillation modes of the system corresponding to the perihelion precession of Jupiter (for the eccentricity) and the nodal regression of Uranus (for the inclination)."[92]
Solar Cycle 25 began in December 2019.[89] Sunspot minimum.
Perihelion: 20 January 2023 at 22:53 MST (05:53 UTC)).
Sunspots and aurorae have been documented back to the Fourth Century A. D.[93][94]
Astrognosy
[edit | edit source]

The model for the interior of Jupiter suggests the occurrence of such materials as metallic hydrogen.
"Recent measurements of Jupiter’s gravitational field indicate that, rather than a dense pit of rock and ice, Jupiter’s core is a haze of heavy elements possibly spanning half the planet’s radius [...]. That observation, made by NASA’s Juno spacecraft that started orbiting Jupiter in 2016, flies in the face of current planet formation models [...]. Those models suggest that Jupiter would have formed from a dense kernel that accumulated a thick envelope of gas."[95]
"New computer simulations now show that a collision between Jupiter and another large planetary body could have shattered Jupiter’s original compact core into the scattered collection of heavy elements seen today."[95]
“This impact may have happened when the solar system was very, very young, and in a chaotic phase when there were lots of objects roaming around. As the biggest planetary body in its neighborhood, Jupiter was liable to gravitationally attract other objects wandering the solar system."[96]
"Billions of years ago, Jupiter may have collided with a rogue planetary body equal to about 10 Earth masses (impact and its aftermath seen from left to right in this computer simulation). That impact could have fractured the gas giant’s original compact core and mixed the heavy elements there into its gaseous envelope to create the bloated, fuzzy core seen today."[95]
A "planetary body of about 10 Earth masses could have broken apart and merged with Jupiter’s dense core, causing that jumble of material to mix into the planet’s inner gaseous envelope. Within hours, the merger would have transformed Jupiter’s original core, around only 15 percent the planet’s radius, into a dilute core that extended to nearly half of Jupiter’s radius. Further simulations confirmed that this diffuse core could have persisted for over 4 billion years to the present day."[95]
"The idea that a giant impact reshaped Jupiter’s internal structure is plausible. But other scenarios — such as heavy elements mixing with gas during Jupiter’s formation, or an internal churning process dredging up core material — may also explain Jupiter’s diffuse core. Computer simulations of those competing scenarios may help scientists tease out which is most likely noting that figuring out how Jupiter formed and evolved is very much “a work in progress.”"[97]
Jupiter systems
[edit | edit source]On the right is a Voyager 2 image of Jupiter's rings.
"A definite color gradient is observed [in the small inner satellites of Jupiter], with the satellites closer to Jupiter being redder: the mean violet/green ratio (0.42/0.56 μm) decreases from Thebe to Metis. This ratio also is lower for the trailing sides of Thebe and Amalthea than for their leading sides."[98]
Io and Europa were seen for the first time as separate bodies during Galileo's observations of the Jupiter system the following day, January 8, 1610 (used as the discovery date for Io by the IAU).[99] The discovery of Io and the other Galilean satellites of Jupiter was published in Galileo's Sidereus Nuncius in March 1610.[100]
Trojan asteroids
[edit | edit source]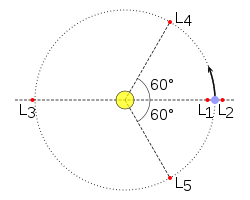
Def. "the L4 and L5 Lagrange points of the Sun-Jupiter orbital configuration"[101] are called the Trojan points.
Def. "an asteroid occupying the Trojan points of the Sun-Jupiter system"[102] is called a Trojan asteroid.
Planetary sciences
[edit | edit source]
The image at right represents "[t]he Jovian magnetosphere [magnetic field lines in blue], including the Io flux tube [in green], Jovian aurorae, the sodium cloud [in yellow], and sulfur torus [in red]."[103]
"Io may be considered to be a unipolar generator which develops an emf [electromotive force] of 7 x 105 volts across its radial diameter (as seen from a coordinate frame fixed to Jupiter)."[104]
"This voltage difference is transmitted along the magnetic flux tube which passes through Io. ... The current [in the flux tube] must be carried by keV electrons which are electrostatically accelerated at Io and at the top of Jupiter's ionosphere."[104]
"Io's high density (4.1 g cm-3) suggests a silicate composition. A reasonable guess for its electrical conductivity might be the conductivity of the Earth's upper mantle, 5 x 10-5 ohm-1 cm-1 (Bullard 1967)."[104]
As "a conducting body [transverses] a magnetic field [it] produces an induced electric field. ... The Jupiter-Io system ... operates as a unipolar inductor" ... Such unipolar inductors may be driven by electrical power, develop hotspots, and the "source of heating [may be] sufficient to account for the observed X-ray luminosity".[105]
"The electrical surroundings of Io provide another energy source which has been estimated to be comparable with that of the [gravitational] tides (7). A current of 5 x 106 A is ... shunted across flux tubes of the Jovian field by the presence of Io (7-9)."[106]
"[W]hen the currents [through Io] are large enough to cause ohmic heating ... currents ... contract down to narrow paths which can be kept hot, and along which the conductivity is high. Tidal heating [ensures] that the interior of Io has a very low eletrical resistance, causing a negligible extra amount of heat to be deposited by this current. ... [T]he outermost layers, kept cool by radiation into space [present] a large resistance and [result in] a concentration of the current into hotspots ... rock resistivity [and] contact resistance ... contribute to generate high temperatures on the surface. [These are the] conditions of electric arcs [that can produce] temperatures up to ionization levels ... several thousand kelvins".[106]
"[T]he outbursts ... seen [on the surface may also be] the result of the large current ... flowing in and out of the domain of Io ... Most current spots are likely to be volcanic calderas, either provided by tectonic events within Io or generated by the current heating itself. ... [A]s in any electric arc, very high temperatures are generated, and the locally evaporated materials ... are ... turned into gas hot enough to expand at a speed of 1 km/s."[106]
Radiative dynamos
[edit | edit source]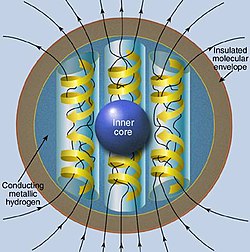
"The interior of Jupiter is the seat of a strong dynamo that produces a surface magnetic field in the equatorial region with an intensity of ~ 4 Gauss. This strong magnetic field and Jupiter’s fast rotation (rotation period ~ 9 h 55 min) create a unique magnetosphere in the solar system which is known for its immense size (average subsolar magnetopause distance 45-100 RJ where 1 RJ = 71492 km is the radius of Jupiter) and fast rotation [...]. Jupiter’s magnetosphere differs from most other magnetospheres in the fact that it derives much of its plasma internally from Jupiter’s moon Io. The heavy plasma, consisting principally of various charge states of S and O, inflates the magnetosphere from the combined actions of centrifugal force and thermal pressure."[107]
In "the absence of an internal heavy plasma, the dipole field would balance the average dynamic pressure of the solar wind (0.08 nPa) at a distance of ~ 42 RJ in the subsolar region [...] the observed average magnetopause location of ~ 75 RJ [...] The heavy plasma is also responsible for generating an azimuthal current exceeding 160 MA in the equatorial region of Jupiter’s magnetosphere where it is confined to a thin current sheet (half thickness ~ 2 RJ in the dawn sector)."[107]
"The energization of plasma by various electrical fields as it diffuses inwards is responsible for the creation of radiation belts in the inner magnetosphere of Jupiter. It is believed that the radial diffusion is driven by the ionospheric dynamo fields produced by winds in Jupiter’s atmosphere"[107]
"In situ and remote observations of Io and its surroundings from Voyager showed that Io is the main source of plasma in Jupiter’s magnetosphere [...] "[107]
"It is estimated that upward of 6 × 1029 amu/s (~ 1 ton/s) of plasma mass is added to the magnetosphere by Io. The picked-up plasma consists mostly of various charged states of S and O and populates a torus region extending from a radial distance of ~ 5.2 RJ to ~ 10 RJ."[107]
"The next most important source of plasma in Jupiter’s magnetosphere is the solar wind whose source strength can be estimated by a consideration of the solar wind mass flux incident on Jupiter’s magnetopause and the fractional amount that makes it into the magnetosphere (< 1%). Such a calculation suggests that the solar wind source strength is < 100 kg/s (Hill et al. 1983) considerably lower than the Io source. Nevertheless, the number density of protons (as opposed to the mass density) may be comparable to the iogenic plasma number density in the middle and outer magnetospheres where the solar wind may be able to gain access to the magnetosphere."[107]
"The escape of ions (mainly H+ and H2+ ) from the ionosphere of Jupiter provides the next significant source of plasma in Jupiter’s magnetosphere. The ionospheric plasma escapes along field lines when the gravity of Jupiter is not able to contain the hot plasma (~ 10 eV and above). The escape however is not uniform and depends on the local photoelectron density, the temperature variations of the ionosphere with the solar zenith angle, other factors such as the auroral precipitation of ions and electrons and the ionospheric heating from Pedersen currents. In situ measurements show that in Io’s torus, protons contribute to less than 20% of total ion number density and constitute < 1% of mass suggesting that the ionosphere is not a major source of plasma in Jupiter’s magnetosphere. [The] ionospheric source strength [is] in the range of ~ 20 kg/s."[107]
The "surface sputtering of the three icy satellites by jovian plasma provides the last significant source of plasma in Jupiter’s magnetosphere. Because the icy moons lack extended atmospheres and the fluxes of the incident plasma are low at the locations of these moons, the total pickup of plasma from these satellites is estimated to be less than 20 kg/s based on the plasma sputtering rates provided".[107]
"Other minor constituents found in the torus [...] were Na+ (with an abundance of < 5%) and molecular ions SO+ and SO2+ (both with abundances of < 1% of the total). The average mass of a torus ion is ~ 20 and the average fractional charge on an ion is ~ 1.2 [...]. The bulk velocity of the plasma was found to be ~ 75 km/s, close to the corotational value."[107]
Classical planets
[edit | edit source]"[O]range [is] the color of Jupiter"[41]
5102 b2k, -3102 or 3102 BC, is the historical year assigned to a Hindu table of planets that does include the classical planet Jupiter.[108] "Babylonian astronomy, too, had a four-planet system. In ancient prayers the planets Saturn, Jupiter, Mars, and Mercury are invoked; ... and one speaks of "the four-planet system of the ancient astronomers of Babylonia."[109]"[110]
~2800 b2k: The observation of Jupiter dates back to the Babylonian astronomers of the 7th or 8th century BC.[111] ... To the Babylonians, this object represented their god Marduk. They used the roughly 12-year orbit of this planet along the ecliptic to define the constellations of their zodiac.[112][113]
2362 b2k: The Chinese historian of astronomy, Xi Zezong, has claimed that Gan De, a Chinese astronomer, made the discovery of one of Jupiter's moons in 362 BC with the unaided eye. The Chinese, Korean and Japanese referred to the planet as the wood star.
The Greeks called it Phaethon, "blazing."
Erentüz
[edit | edit source]In the Central Asian-Turkic myths, Jupiter is called an "Erendiz/Erentüz", which means "eren(?)+yultuz(star)". There are many theories about meaning of "eren". Also, these peoples calculated the orbit of Jupiter as 11 years and 300 days. They believed that some social and natural events connected to Erentüz's movements on the sky.[114]
Guru
[edit | edit source]Hindu astrologers named the planet after Brihaspati, the religious teacher of the gods, and often called it "Guru", which literally means the "Heavy One."[115]
Poeninus
[edit | edit source]Mercury was the most honoured of all the gods and many images of him were to be found. Mercury was regarded as the inventor of all the arts, the patron of travellers and of merchants, and the most powerful god in matters of commerce and gain. After him, the Gauls honoured Apollo, who drove away diseases, Mars, who controlled war, Jupiter, who ruled the heavens, and Minerva, who promoted handicrafts. He adds that the Gauls regarded Dis Pater as their ancestor.[116] The names of Roman gods are coupled with native Celtic theonyms and epithets, such as Mercury Visucius, Lenus Mars, Jupiter Poeninus, or Sulis Minerva.
Thor
[edit | edit source]Thor [is] associated with the planet Jupiter in Germanic mythology.[117]
Tinia
[edit | edit source]Tinia (also Tin, Tinh, Tins or Tina) was the god of the sky and the highest god in Etruscan mythology, equivalent to the Roman Jupiter and the Greek Zeus.[118]
Zeus
[edit | edit source]In the ancient Greek religion, Zeus (Ancient Greek is the "Father of Gods and men". He is the god of sky and thunder in Greek mythology. His Roman counterpart is Jupiter and Etruscan counterpart is Tinia. Zeus is the child of Cronus and Rhea, and the youngest of his siblings. In most traditions he is married to Hera, although, at the oracle of Dodona, his consort is Dione: according to the Iliad, he is the father of Aphrodite by Dione.
Observatory geology
[edit | edit source]
Observatory geology has two forms:
- the geological study necessary to put an observatory on a solid foundation to maximize telescope function through minimizing ground-based vibration and
- imaging terrain by the observatory so that geological study may be performed.
"Images of Europa from the Galileo spacecraft [launch release at right from the shuttle Atlantis] show a surface with a complex history involving tectonic deformation, impact cratering, and possible emplacement of ice-rich materials and perhaps liquids on the surface. Differences in impact crater distributions suggest that some areas have been resurfaced more recently than others; Europa could experience current cryovolcanic and tectonic activity. Global-scale patterns of tectonic features suggest deformation resulting from non-synchronous rotation of Europa around Jupiter. Some regions of the lithosphere have been fractured, with icy plates separated and rotated into new positions. The dimensions of these plates suggest that the depth to liquid or mobile ice was only a few kilometers at the time of disruption. Some surfaces have also been upwarped, possibly by diapirs, cryomagmatic intrusions, or convective upwelling. In some places, this deformation has led to the development of chaotic terrain in which surface material has collapsed and/or been eroded"[119]
Planetary observatories
[edit | edit source]
"The 0.6m Tortugas Mountain Observatory is used to monitor the temporal changes in the Jovian cloud deck and equatorial activity on Saturn. The data are collected with a CCD camera, archived at the NMSU astronomy department and made available to the Astronomical community through the NASA Planetary Data System Subnode. Images collected over the last 27 years are being used as a climatic data base to interpret the Hubble Space Telescope ~HST!, Galileo and Cassini data. Although funding has been reduced, simultaneous observations are scheduled when the 3.5 meter telescope is used for infrared imaging of Jupiter."[120]
Airborne astronomy
[edit | edit source]
"SOFIA, the Stratospheric Observatory for Infrared Astronomy, captured its "first light" images on May 26, from an altitude of 35,000 feet. While flying above most of planet Earth's infrared-absorbing water vapor, SOFIA's premier infrared views of the cosmos included this remarkable false-color image (right panel) of Jupiter. For comparison, on the left is a recent, ground-based visible light image. Both show our solar system's ruling gas giant without its dark southern equatorial belt (normally seen in the upper hemisphere in this orientation). That familiar feature faded from view early in May. But the bright white stripe in SOFIA's image is a region of Jupiter's clouds transparent to infrared light, offering a glimpse below the cloud tops."[121]
Hypotheses
[edit | edit source]- Certain conjunctions of Jupiter with Venus occur in the same cycle as the main sunspot cycle.
- The conjunctions of Venus and Jupiter may be causing the sunspot cycle as a result of enhanced electron flux to the Sun and positive ions from the Sun to Jupiter.
- The peak of the sunspot cycle occurs at Jupiter and Venus perihelion.
- The peaks of the sunspot cycles occur due to the maximizing of the electric currents from the planets to the Sun.
- The sunspot minimums are due to the minimizing of electric currents from the planets to the Sun.
See also
[edit | edit source]References
[edit | edit source]- ↑ P. Kenneth Seidelmann; B. A. Archinal; M. F. A'hearn; A. Conrad; G. J. Consolmagno; D. Hestroffer; J. L. Hilton; G. A. Krasinsky et al. (2007). "Report of the IAU/IAG Working Group on cartographic coordinates and rotational elements: 2006". Celestial Mechanics and Dynamical Astronomy 98 (3): 155-80. doi:10.1007/s10569-007-9072-y.
- ↑ 2.0 2.1 2.2 2.3 2.4 Williams, David R. (November 16, 2004). "Jupiter Fact Sheet". NASA. Retrieved 2007-02-21.
- ↑ "Jupiter". European Space Agency. September 20, 2004. Retrieved 2007-02-21.
- ↑ Yeomans, Donald K. (2006-07-13). "HORIZONS System". NASA JPL. Retrieved 2007-08-08. — At the site, go to the "web interface" then select "Ephemeris Type: Elements", "Target Body: Jupiter Barycenter" and "Center: Sun".
- ↑ Orbital elements refer to the barycenter of the Jupiter system, and are the instantaneous osculating values at the precise J2000 epoch. Barycenter quantities are given because, in contrast to the planetary centre, they do not experience appreciable changes on a day-to-day basis due to the motion of the moons.
- ↑ 6.0 6.1 "The MeanPlane (Invariable plane) of the Solar System passing through the barycenter". 2009-04-03. Retrieved 2009-04-10. (produced with Solex 10 written by Aldo Vitagliano; see also Invariable plane)
- ↑ 7.0 7.1 7.2 Seligman, Courtney. "Rotation Period and Day Length". Retrieved 2009-08-13.
- ↑ Simon, J.L.; Bretagnon, P.; Chapront, J.; Chapront-Touzé, M.; Francou, G.; Laskar, J. (February 1994). "Numerical expressions for precession formulae and mean elements for the Moon and planets". Astronomy and Astrophysics 282 (2): 663–683.
- ↑ Williams, David R. (December 23, 2021). "Jupiter Fact Sheet". NASA. Retrieved October 13, 2017.
- ↑ 10.00 10.01 10.02 10.03 10.04 10.05 10.06 10.07 10.08 10.09 10.10 10.11 10.12 10.13 10.14 10.15 Simon, J. L.; Bretagnon, P.; Chapront, J.; Chapront-Touzé, M.; Francou, G.; Laskar, J. (February 1994). "Numerical expressions for precession formulae and mean elements for the Moon and planets". Astronomy and Astrophysics 282 (2): 663–683. Cite error: Invalid
<ref>
tag; name "Simon" defined multiple times with different content - ↑ Souami, D.; Souchay, J. (July 2012). "The solar system's invariable plane". Astronomy & Astrophysics 543: 11. doi:10.1051/0004-6361/201219011. A133.
- ↑ "HORIZONS Planet-center Batch call for January 2023 Perihelion". ssd.jpl.nasa.gov (Perihelion for Jupiter's planet-centre (599) occurs on 2023-Jan-21 at 4.9510113au during a rdot flip from negative to positive). NASA/JPL. Retrieved 2021-09-07.
- ↑ Immanuel Velikovsky. Uranus. The Immanuel Velikovsky Archive. http://www.varchive.org/itb/uranus.htm#f_1. Retrieved 2013-01-14.
- ↑ 14.0 14.1 I. de Pater; M. Wong (4 May 2006). Hubble Snaps Baby Pictures of Jupiter's "Red Spot Jr.". Baltimore, Maryland USA: HubbleSite. http://hubblesite.org/news_release/news/2006-19/20-jupiter. Retrieved 2017-02-12.
- ↑ Dennis Overbye (2009-07-24). Hubble Takes Snapshot of Jupiter’s ‘Black Eye’. New York Times. http://www.nytimes.com/2009/07/25/science/space/25hubble.html?ref=science. Retrieved 2009-07-25.
- ↑ Lovett, Richard A. (December 15, 2006). Stardust's Comet Clues Reveal Early Solar System. National Geographic News. http://news.nationalgeographic.com/news/2006/12/061215-comet-stardust.html. Retrieved 2007-01-08.
- ↑ Nakamura, T.; Kurahashi, H. (1998). "Collisional Probability of Periodic Comets with the Terrestrial Planets: An Invalid Case of Analytic Formulation". Astronomical Journal 115 (2): 848–54. doi:10.1086/300206. http://www.iop.org/EJ/article/1538-3881/115/2/848/970144.html. Retrieved 2007-08-28.
- ↑ Tabe, Isshi; Watanabe; Jimbo (February 1997). "Discovery of a Possible Impact SPOT on Jupiter Recorded in 1690". Publications of the Astronomical Society of Japan 49: L1–L5.
- ↑ 19.0 19.1 Franck Marchis (2012-09-10). Another fireball on Jupiter?. Cosmic Diary blog. http://cosmicdiary.org/fmarchis/2012/09/10/another-fireball-on-jupiter/. Retrieved 2012-09-11.
- ↑ Baalke, Ron. Comet Shoemaker-Levy Collision with Jupiter. NASA. http://www2.jpl.nasa.gov/sl9/. Retrieved 2007-01-02.
- ↑ Britt, Robert R. (August 23, 2004). Remnants of 1994 Comet Impact Leave Puzzle at Jupiter. space.com. http://www.space.com/scienceastronomy/mystery_monday_040823.html. Retrieved 2007-02-20.
- ↑ Staff (2009-07-21). "Amateur astronomer discovers Jupiter collision". ABC News online. http://www.abc.net.au/news/stories/2009/07/21/2632368.htm. Retrieved 2009-07-21.
- ↑ Salway, Mike (July 19, 2009). "Breaking News: Possible Impact on Jupiter, Captured by Anthony Wesley". Ice In Space News (IceInSpace). http://www.iceinspace.com.au/index.php?id=70,550,0,0,1,0. Retrieved 2009-07-19.
- ↑ Grossman, Lisa (July 20, 2009). "Jupiter sports new 'bruise' from impact". New Scientist. http://www.newscientist.com/article/dn17491-jupiter-sports-new-bruise-from-impact.html.
- ↑ Bakich, Michael (2010-06-04). Another impact on Jupiter. Astronomy Magazine online. http://www.astronomy.com/asy/default.aspx?c=a&id=9918. Retrieved 2010-06-04.
- ↑ Beatty, Kelly (22 August 2010). Another Flash on Jupiter!. Sky Publishing. http://web.archive.org/web/20100827180208/http://www.skyandtelescope.com/community/skyblog/observingblog/101264994.html. Retrieved 23 August 2010. "Masayuki Tachikawa was observing ... 18:22 Universal Time on the 20th ... Kazuo Aoki posted an image ... Ishimaru of Toyama prefecture observed the event"
- ↑ Hall, George (September 2012). George's Astrophotography. http://georgeastro.weebly.com/jupiter.html. Retrieved 17 September 2012. "10 Sept. 2012 11:35 UT .. observed by Dan Petersen"
- ↑ 28.0 28.1 28.2 28.3 28.4 28.5 Nancy Atkinson (24 December 2015). How Jupiter is Getting Its Belt Back. Universe Today. http://www.universetoday.com/79931/how-jupiter-is-getting-its-belt-back/. Retrieved 2017-02-12.
- ↑ 29.0 29.1 29.2 29.3 Scott Bolton (30 May 2017). 'It's Snowing on Jupiter': Stunning Photos Show Clouds High in Gas Giant's Skies. Space.com. http://www.space.com/37009-jupiter-snow-high-clouds-juno-photos.html. Retrieved 2017-06-04.
- ↑ Barry H. Mauk; Joachim Saur (October 26, 2007). "Equatorial electron beams and auroral structuring at Jupiter". Journal of Geophysical Research 112 (A10221): 20. doi:10.1029/2007JA012370. http://www.agu.org/journals/ja/ja0710/2007JA012370/figures.shtml. Retrieved 2012-06-02.
- ↑ C. T. Russell; D. N. Baker; J. A. Slavin (January 1, 1988). Faith Vilas. ed. The Magnetosphere of Mercury, In: Mercury. Tucson, Arizona, United States of America: University of Arizona Press. pp. 514-61. ISBN 0816510857. Bibcode: 1988merc.book..514R. http://www-ssc.igpp.ucla.edu/personnel/russell/papers/magMercury.pdf. Retrieved 2012-08-23.
- ↑ NASA/CXC/SWRI/G.R.Gladstone (February 27, 2002). Jupiter Hot Spot Makes Trouble For Theory. Cambridge, Massachusetts: Harvard-Smithsonian Center for Astrophysics. http://chandra.harvard.edu/photo/2002/0001/. Retrieved 2012-07-11.
- ↑ R.Elsner; M.Weiss (March 2, 2005). Jupiter: Chandra Probes High-Voltage Auroras on Jupiter. Cambridge, Massachusetts: Harvard-Smithsonian Center for Astrophysics. http://chandra.harvard.edu/photo/2005/jupiter/. Retrieved 2012-07-11.
- ↑ T. E. Cravens (1987). "Vibrationally excited molecular hydrogen in the upper atmosphere of Jupiter". Journal of Geophysical Research 92 (A10): 11,083-100. doi:10.1029/JA092iA10p11083. http://www.agu.org/pubs/crossref/1987/JA092iA10p11083.shtml. Retrieved 2012-07-09.
- ↑ Zezong, Xi, "The Discovery of Jupiter's Satellite Made by Gan De 2000 years Before Galileo", Chinese Physics 2 (3) (1982): 664–67.
- ↑ 36.0 36.1 Image Processing Laboratory (April 6, 2000). PIA02257: Voyager 2 Jupiter Eruption Movie. Pasadena, California USA: NASA/JPL. http://photojournal.jpl.nasa.gov/catalog/PIA02257. Retrieved 2013-03-22.
- ↑ Voyager 1 team (February 9, 1979). Jupiter, Io - Voyager 1. Greenbelt, Maryland USA: NASA Goddard Space Flight Center. http://nssdc.gsfc.nasa.gov/imgcat/html/object_page/vg1_1567237.html. Retrieved 2013-03-22.
- ↑ 38.0 38.1 38.2 Sue Lavoie (March 6, 1998). PIA01195: Hazes near Jupiter's Limb (60 degrees North, 315 degrees West). Pasadena, California USA: NASA/JPL. http://photojournal.jpl.nasa.gov/catalog/pia01195. Retrieved 2013-04-01.
- ↑ 39.0 39.1 39.2 39.3 39.4 Sue Lavoie (December 28, 2000). PIA02863: Planetwide Color Movie. Tucson, Arizona USA: NASA/JPL/University of Arizona. http://photojournal.jpl.nasa.gov/catalog/PIA02863. Retrieved 2013-05-30.
- ↑ filters - popular and hot telescope filters. Lumicon international. http://web.archive.org/web/20101125034023/http://lumicon.com/astronomy-accessories.php?cid=1&cn=Filters. Retrieved 2010-11-22.
- ↑ 41.0 41.1 Faber Birren (Summer 1983). "Color and human response". Color Research and Application 8 (2): 75-81. doi:10.1002/col.5080080204. http://onlinelibrary.wiley.com/doi/10.1002/col.5080080204/abstract. Retrieved 2012-04-23.
- ↑ Elkins-Tanton, Linda T. (2006). Jupiter and Saturn. New York: Chelsea House. ISBN 0-8160-5196-8.
- ↑ Strycker, P. D.; Chanover, N.; Sussman, M.; Simon-Miller, A. (2006). A Spectroscopic Search for Jupiter's Chromophores. American Astronomical Society. Bibcode: 2006DPS....38.1115S.
- ↑ Gierasch, Peter J.; Nicholson, Philip D. (2004). Jupiter. World Book @ NASA. http://www.nasa.gov/worldbook/jupiter_worldbook.html. Retrieved 2006-08-10.
- ↑ 45.0 45.1 Glenn Orton (24 December 2015). How Jupiter is Getting Its Belt Back. Universe Today. http://www.universetoday.com/79931/how-jupiter-is-getting-its-belt-back/. Retrieved 2017-02-12.
- ↑ Staff (2007). Jupiter Data Sheet – SPACE.com. Imaginova. http://www.space.com/scienceastronomy/solarsystem/jupiter-ez.html. Retrieved 2008-06-03.
- ↑ Anonymous (August 10, 2000). The Solar System – The Planet Jupiter – The Great Red Spot. Dept. Physics & Astronomy – University of Tennessee. http://csep10.phys.utk.edu/astr161/lect/jupiter/redspot.html. Retrieved 2008-06-03.
- ↑ Fletcher, Leigh N.; Orton,, G.S.; Mousis et. al, O.; Yanamandra-Fisher, P.; Parrish, P.D.; Irwin, P.G.J.; Fisher, B.M.; Vanzi, L. et al. (2010). "Thermal structure and composition of Jupiter's Great Red Spot from high-resolution thermal imaging". Icarus 208 (1): 306–328. doi:10.1016/j.icarus.2010.01.005. http://www.eso.org/public/archives/releases/sciencepapers/eso1010/eso1010.pdf.
- ↑ Beebe, R. (1997). Jupiter the Giant Planet (2nd ed.). Washington: Smithsonian Books. ISBN 1-56098-685-9. OCLC 224014042.
- ↑ 50.0 50.1 50.2 50.3 Bob King (23 December 2015). Will Jupiter’s Great Red Spot Turn into a Wee Red Dot?. Universe Today. http://www.universetoday.com/108257/will-jupiters-great-red-spot-turn-into-a-wee-red-dot/. Retrieved 2017-02-12.
- ↑ 51.0 51.1 Sang J. Kim; John Caldwell; A.R. Rivolo; R. Wagener; Glenn S. Orton (November 1985). "Infrared polar brightening on Jupiter. III - Spectrometry from the Voyager 1 IRIS experiment". Icarus 64 (2): 233-48. doi:10.1016/0019-1035(85)90088-0. http://www.sciencedirect.com/science/article/pii/0019103585900880. Retrieved 2012-07-09.
- ↑ ESO/F. Marchis; M. Wong; E. Marchetti; P. Amico; S. Tordo (October 2, 2008). Sharpening up Jupiter. ESO Santiago, Chile: ESO. http://www.eso.org/public/images/eso0833a/. Retrieved 2012-07-11.
- ↑ Phil Davis (May 3, 2011). Triple Eclipse. National Aeronautics and Space Administration. http://solarsystem.nasa.gov/multimedia/display.cfm?Category=Planets&IM_ID=3083. Retrieved 2012-07-20.
- ↑ Mystery impact leaves Earth-sized mark on Jupiter. CNN. July 21, 2009. http://www.cnn.com/2009/TECH/space/07/21/jupiter.nasa.meteor.scar/index.html.
- ↑ Overbye, Dennis (July 22, 2009). All Eyepieces on Jupiter After a Big Impact. New York Times. http://www.nytimes.com/2009/07/22/science/space/22jupiter.html?hpw.
- ↑ Amateur astronomer spots Earth-size scar on Jupiter, Guardian, July 21, 2009
- ↑ Sue Lavoie01 (December 31, 2010). Acetylene at Jupiter's North and South Poles. Ministry of Space Exploration. http://minsex.blogspot.com/2010_12_01_archive.html. Retrieved 2013-02-06.
- ↑ J. O’Donoghue, L. Moore, T. Bhakyapaibul, H. Melin, T. Stallard, J. E. P. Connerney & C. Tao (4 August 2021). "Global upper-atmospheric heating on Jupiter by the polar aurorae". Nature 596: 54-57. doi:10.1038/s41586-021-03706-w. https://www.nature.com/articles/s41586-021-03706-w. Retrieved 9 August 2021.
- ↑ M.J. Griffin; P.A.R. Ade; G.S. Orton; E.I. Robson; W.K. Gear; I.G. Nolt; J.V. Radostitz (February-March 1986). "Submillimeter and millimeter observations of Jupiter". Icarus 65 (2-3): 244-56. doi:10.1016/0019-1035(86)90137-5. http://www.sciencedirect.com/science/article/pii/0019103586901375. Retrieved 2012-08-04.
- ↑ 60.0 60.1 60.2 Imke de Pater (2 June 2016). New radio map of Jupiter reveals what’s beneath colorful clouds. Berkeley, Calfornia USA: University of California, Berkeley. http://news.berkeley.edu/2016/06/02/new-radio-map-of-jupiter-reveals-whats-beneath-colorful-clouds/. Retrieved 2016-08-18.
- ↑ 61.0 61.1 61.2 Robert Sanders (2 June 2016). New radio map of Jupiter reveals what’s beneath colorful clouds. Berkeley, Calfornia USA: University of California, Berkeley. http://news.berkeley.edu/2016/06/02/new-radio-map-of-jupiter-reveals-whats-beneath-colorful-clouds/. Retrieved 2016-08-18.
- ↑ 62.0 62.1 Michael Wong (2 June 2016). New radio map of Jupiter reveals what’s beneath colorful clouds. Berkeley, Calfornia USA: University of California, Berkeley. http://news.berkeley.edu/2016/06/02/new-radio-map-of-jupiter-reveals-whats-beneath-colorful-clouds/. Retrieved 2016-08-18.
- ↑ Robert Sault (2 June 2016). New radio map of Jupiter reveals what’s beneath colorful clouds. Berkeley, Calfornia USA: University of California, Berkeley. http://news.berkeley.edu/2016/06/02/new-radio-map-of-jupiter-reveals-whats-beneath-colorful-clouds/. Retrieved 2016-08-18.
- ↑ 64.0 64.1 Linda T. Elkins-Tanton (2006). Jupiter and Saturn. New York: Chelsea House. ISBN 0-8160-5196-8.
- ↑ Weintraub, Rachel A. (September 26, 2005). How One Night in a Field Changed Astronomy. NASA. http://www.nasa.gov/vision/universe/solarsystem/radio_jupiter.html. Retrieved 2007-02-18.
- ↑ Garcia, Leonard N. The Jovian Decametric Radio Emission. NASA. http://radiojove.gsfc.nasa.gov/library/sci_briefs/decametric.htm. Retrieved 2007-02-18.
- ↑ Klein, M. J.; Gulkis, S.; Bolton, S. J. (1996). Jupiter's Synchrotron Radiation: Observed Variations Before, During and After the Impacts of Comet SL9. NASA. http://deepspace.jpl.nasa.gov/technology/TMOT_News/AUG97/jupsrado.html. Retrieved 2007-02-18.
- ↑ 68.0 68.1 Gordon H. Pettengill; Irwin I. Shapiro (1965). "Radar Astronomy". Annual Review of Astronomy and Astrophysics 3: 377-410.
- ↑ Irwin I. Shapiro (March 1968). "Planetary radar astronomy". Spectrum, IEEE 5 (3): 70-9. doi:10.1109/MSPEC.1968.5214821. http://ieeexplore.ieee.org/xpls/abs_all.jsp?arnumber=5214821. Retrieved 2012-12-25.
- ↑ R. B. Dyce; G. H. Pettengill; A. D. Sanchez (August 1967). "Radar Observations of Mars and Jupiter at 70 cm". The Astronomical Journal 72 (4): 771-7. doi:10.1086/110307. http://articles.adsabs.harvard.edu/cgi-bin/nph-iarticle_query?1967AJ.....72..771D&data_type=PDF_HIGH&whole_paper=YES&type=PRINTER&filetype=.pdf. Retrieved 2012-12-25.
- ↑ S.G. Djorgovski (16 March 2016). A Tour of the Radio Universe. National Radio Astronomy Observatory. http://www.cv.nrao.edu/course/astr534/Tour.html. Retrieved 2014-03-16.
- ↑ 72.0 72.1 72.2 72.3 Sue Lavoie (13 December 2000). PIA02856: High Latitude Mottling on Jupiter. Pasadena, California USA: NASA/JPL. http://photojournal.jpl.nasa.gov/catalog/PIA02856. Retrieved 2017-02-12.
- ↑ 73.0 73.1 73.2 Sue Lavoie (27 March 2006). PIA07783: Cassini's Best Maps of Jupiter (North Polar Map). NASA/JPL. http://photojournal.jpl.nasa.gov/catalog/PIA07783. Retrieved 2017-02-12.
- ↑ Betsy Asher Hall; Gervasio Robles (25 May 2017). PIA21641: Southern Storms. Pasadena, California USA: NASA/JPL. https://photojournal.jpl.nasa.gov/catalog/PIA21641. Retrieved 2017-07-10.
- ↑ Damian Peach (23 December 2015). Once Around The Sun With Jupiter. Universe Today. http://www.universetoday.com/121259/once-around-the-sun-with-jupiter/. Retrieved 2017-02-12.
- ↑ 76.0 76.1 76.2 76.3 Bob King (23 December 2015). Once Around The Sun With Jupiter. Universe Today. http://www.universetoday.com/121259/once-around-the-sun-with-jupiter/. Retrieved 2017-02-12.
- ↑ 77.0 77.1 77.2 A. Wesley; A. Kazemoto; C. Go (March 2012). Global Upheaval at Jupiter. SWRI. https://www.missionjuno.swri.edu/media-gallery/jupiter. Retrieved 2017-02-12.
- ↑ MJ Valtonen (February 1983). "On the capture of comets into the Solar System". The Observatory 103 (2): 1-4.
- ↑ 79.0 79.1 M. J. Valtonen; K. A. Innanen (April 1982). "The capture of interstellar comets". The Astrophysical Journal 255 (4): 307-15. doi:10.1086/159830.
- ↑ 80.0 80.1 Sergey A. Astakhov; David Farrelly (November 2004). "Capture and escape in the elliptic restricted three?body problem". Monthly Notices of the Royal Astronomical Society 354 (4): 971-9. doi:10.1111/j.1365-2966.2004.08280.x. http://arxiv.org/pdf/astro-ph/0408271. Retrieved 2012-03-12.
- ↑ Horizons output. Favorable Appearances by Jupiter. http://home.surewest.net/kheider/astro/jup2010.txt. Retrieved 2008-01-02. (Horizons)
- ↑ S.D. Verma (1986). K. B. Bhatnagar. ed. Influence of Planetary Motion and Radial Alignment of Planets on Sun, In: Space Dynamics and Celestial Mechanics. 127. Springer Netherlands. pp. 143-54. doi:10.1007/978-94-009-4732-0_13. ISBN 978-94-010-8603-5. http://link.springer.com/chapter/10.1007/978-94-009-4732-0_13. Retrieved 2013-07-07.
- ↑ 83.0 83.1 Ray Tomes (February 1990). Towards a Unified Theory of Cycles. Cycles Research Institute. pp. 21. http://cyclesresearchinstitute.org/cycles-general/tomes_unified_cycles.pdf. Retrieved 2013-07-07.
- ↑ 84.0 84.1 Jean Meeus (1998). "Planet Apsides". Astronomical Algorithms. https://stjerneskinn.com/planet-apsides.htm. Retrieved 25 September 2022.
- ↑ esa. "SOHO: the new solar cycle starts with a 'bang'". European Space Agency. Retrieved 2017-05-11.
- ↑ Tony Phillips (2008-01-10). "Solar Cycle 24 begins". NASA. Retrieved 2010-05-29.
- ↑ Tony Phillips (2010-06-04). "As the Sun Awakens, NASA Keeps a Wary Eye on Space Weather". NASA. Retrieved 2013-05-18.
- ↑ "Solar Cycle Progression". www.swpc.noaa.gov. NOAA / NWS Space Weather Prediction Center. Retrieved 2015-07-06.
- ↑ 89.0 89.1 National Weather Service. "Hello Solar Cycle 25". Retrieved 15 September 2020.
- ↑ A. P. Vidmachenko (2016). "Seasonal Changes on Jupiter: 1. Factor of Activity of the Hemispheres". Kinematics and Physics of Celestial Bodies 32 (4): 189-195. doi:10.3103/S0884591316040073. https://www.researchgate.net/profile/A-Vidmachenko/publication/307115730_Seasonal_changes_on_Jupiter_1_Factor_of_activity_of_the_hemispheres/links/5ca0bedf92851cf0aea42491/Seasonal-changes-on-Jupiter-1-Factor-of-activity-of-the-hemispheres.pdf. Retrieved 18 May 2022.
- ↑ Constantine C.C. Tsang, John R. Spencer, Emmanuel Lellouch, Miguel A. López-Valverde, Matthew J. Richter, Thomas K. Greathouse and Henry Roe (September–October 2013). "Io's contracting atmosphere post 2011 perihelion: Further evidence for partial sublimation support on the anti-Jupiter hemisphere". Icarus 226 (1): 1177-1181. doi:10.1016/j.icarus.2013.06.032. https://www.sciencedirect.com/science/article/abs/pii/S0019103513002972. Retrieved 24 September 2022.
- ↑ Fernando Roig, David Nesvorný, and Sandro Ricardo DeSouza (1 April 2016). "Jumping Jupiter Can Explain Mercury's Orbit". The Astrophysical Journal Letters 820 (2): L30-L34. doi:10.3847/2041-8205/820/2/L30. https://iopscience.iop.org/article/10.3847/2041-8205/820/2/L30/pdf. Retrieved 24 September 2022.
- ↑ Schove, D Justin (April–June 1951). "Sunspots, Aurorae and Blood Rain: The Spectrum of Time". Isis (The University of Chicago Press on behalf of The History of Science Society) 42 (2): 133–138. doi:10.1086/349284. PMID 14840957.
- ↑ Schove, D Justin; Ho, Peng-Yoke (June 1967). "Chinese Records of Sunspots and Aurorae in the Fourth Century A. D.". Isis (The University of Chicago Press on behalf of The History of Science Society) 87 (2): 105–112. doi:10.2307/597392.
- ↑ 95.0 95.1 95.2 95.3 Maria Temming (14 August 2019). "A planetary body may have smashed into Jupiter, creating its weird core". Science News. Retrieved 15 August 2019.
- ↑ Andrea Isella (14 August 2019). "A planetary body may have smashed into Jupiter, creating its weird core". Science News. Retrieved 15 August 2019.
- ↑ Scott Bolton (14 August 2019). "A planetary body may have smashed into Jupiter, creating its weird core". Science News. Retrieved 15 August 2019.
- ↑ P.C. Thomas; J.A. Burns; L. Rossier; D. Simonelli; J. Veverka; C.R. Chapman; K. Klaasen; T.V. Johnson et al. (September 1998). "The Small Inner Satellites of Jupiter". Icarus 135 (1): 360-71. doi:10.1006/icar.1998.5976. http://www.sciencedirect.com/science/article/pii/S0019103598959760. Retrieved 2012-06-01.
- ↑ Jennifer Blue (November 9, 2009). Planet and Satellite Names and Discoverers. USGS. http://planetarynames.wr.usgs.gov/append7.html. Retrieved 2010-01-13.
- ↑ D. P. Cruikshank; R. M. Nelson (2007). "A history of the exploration of Io". In Lopes, R. M. C.. Io after Galileo. Springer-Praxis. pp. 5–33. ISBN 3-540-34681-3.
- ↑ 65.94.44.124 (11 December 2010). Trojan point. San Francisco, California: Wikimedia Foundation, Inc. https://en.wiktionary.org/wiki/Trojan_point. Retrieved 2015-08-31.
- ↑ 65.94.44.124 (11 December 2010). Trojan asteroid. San Francisco, California: Wikimedia Foundation, Inc. https://en.wiktionary.org/wiki/Trojan_asteroid. Retrieved 2015-08-31.
- ↑ John Spencer (November 2000). John Spencer's Astronomical Visualizations. Boulder, Colorado USA: University of Colorado. http://www.boulder.swri.edu/~spencer/digipics.html. Retrieved 2013-04-05.
- ↑ 104.0 104.1 104.2 Peter Goldreich; Donald Lynden-Bell (April 1969). "Io, a jovian unipolar inductor". The Astrophysical Journal 156 (04): 59-78. doi:10.1086/149947.
- ↑ Kinwah Wu; Mark Cropper; Gavin Ramsay; Kazuhiro Sekiguchi (March 2002). "An electrically powered binary star?". Monthly Notices of the Royal Astronomical Society 321 (1): 221-7. doi:10.1046/j.1365-8711.2002.05190.x.
- ↑ 106.0 106.1 106.2 Thomas Gold (November 1979). "Electrical Origin of the Outbursts on Io". Science 206 (4422): 1071-3. doi:10.1126/science.206.4422.1071.
- ↑ 107.0 107.1 107.2 107.3 107.4 107.5 107.6 107.7 107.8 Krishan K. Khurana; Margaret G. Kivelson; Vytenis M. Vasyliunas; Norbert Krupp; Joachim Woch; Andreas Lagg; Barry H. Mauk; William S. Kurth (2004). Bagenal, F.. ed. The Configuration of Jupiter’s Magnetosphere, In: Jupiter: The Planet, Satellites and Magnetosphere. Cambridge University Press. pp. 24. ISBN 0-521-81808-7. http://www.igpp.ucla.edu/people/mkivelson/Publications/279-Ch24.pdf. Retrieved 2014-03-29.
- ↑ Jean Baptiste Joseph Delambre (1817). Histoire de l'astronomie ancienne. Paris: Courcier. pp. 639. http://books.google.com/books?id=2lVUjJSxjhQC&pg=PR3&source=gbs_selected_pages&cad=3#v=onepage&f=false. Retrieved 2012-01-13.
- ↑ Ernst Friedrich Weidner (1915). Handbuch der babylonischen Astronomie, Volume 1. J. C. Hinrichs. pp. 146. http://books.google.com/books?id=K6NDAAAAYAAJ&hl=en. Retrieved 2012-03-30.
- ↑ Immanuel Velikovsky (January 1965). Worlds in Collision. New York: Dell Publishing Co., Inc.. pp. 401. http://books.google.com/books?id=FJst27kSVBgC&pg=PA13&dq=%22Worlds+in+Collision%22+1965&hl=en. Retrieved 2012-01-13.
- ↑ A. Sachs (May 2, 1974). "Babylonian Observational Astronomy". Philosophical Transactions of the Royal Society of London (Royal Society of London) 276 (1257): 43–50 (see p. 44). doi:10.1098/rsta.1974.0008.
- ↑ Eric Burgess (1982). By Jupiter: Odysseys to a Giant. New York: Columbia University Press. ISBN 0-231-05176-X.
- ↑ Rogers, J. H. (1998). "Origins of the ancient constellations: I. The Mesopotamian traditions". Journal of the British Astronomical Association, 108: 9–28.
- ↑ Türk Astrolojisi. ntvmsnbc.com. http://www.ntvmsnbc.com/id/25085903/. Retrieved 2010-04-23.
- ↑ Guru. Indian Divinity.com. http://www.webonautics.com/mythology/guru_jupiter.html. Retrieved 2007-02-14.
- ↑ Julius Caesar, Commentarii de Bello Gallico 6:17-18
- ↑ Falk, Michael (1999). "Astronomical Names for the Days of the Week". Journal of the Royal Astronomical Society of Canada 93: 122–33. doi:10.1016/j.newast.2003.07.002.
- ↑ de Grummond, Etruscan Myth, Sacred History and Legend, page 53
- ↑ Ronald Greeley; Robert Sullivan; James Klemaszewski; Kim Homan; James W. Head III; Robert T. Pappalardo; Joseph Veverka; Beth E. Clark et al. (September 1998). "Europa: Initial Galileo Geological Observations". Icarus 135 (1): 4-24. http://www.sciencedirect.com/science/article/pii/S0019103598959693. Retrieved 2013-12-09.
- ↑ A. Klypin (January 1996). "New Mexico State University, Department of Astronomy, Las Cruces, New Mexico 88003. Report for the year 1995.". Bulletin of the Astronomical Society 28 (1): 495-501. http://aas.org/archives/BAAS/v28n1/061601basr.pdf. Retrieved 2013-10-27.
- ↑ Robert Nemiroff; Jerry Bonnell (3 June 2010). Jupiter from the Stratosphere. Washington, DC USA: NASA. https://apod.nasa.gov/apod/ap100603.html. Retrieved 2017-02-12.
External links
[edit | edit source]{{Dominant group}}{{History of science resources}}{{Humanities resources}}
- Articles with hatnote templates targeting a nonexistent page
- Radiation astronomy/Lectures
- Astrophysics/Lectures
- Atmospheric sciences/Lectures
- Cultures/Lectures
- Dominant group/Lectures
- Gases/Lectures
- History of Sciences/Lectures
- Humanities/Lectures
- Planets/Lectures
- Stars/Lectures
- Astronomical objects/Lectures
- Jupiter