Materials Science and Engineering/Doctoral review questions/Major Material Classes
Examples of Major Material Classes
[edit | edit source]Indirect semiconductor: Si
[edit | edit source]
In semiconductor physics, an indirect bandgap is a bandgap in which the minimum energy in the conduction band is shifted by a k-vector relative to the valence band. The k-vector difference represents a difference in momentum.
Semiconductors that have an indirect bandgap are inefficient at emitting light. This is because any electrons present in the conduction band quickly settle into the energy minimum of that band. Electrons in this minimum require some source of momentum allowing them to overcome the offset and fall into the valence band. Photons have very little momentum compared to this energy offset –. The momentum "kick" of a photon being emitted or absorbed is negligible and direct transitions are essentially 'vertical' in k-space.
Since the electron cannot rejoin the valence band by radiative recombination, conduction band electrons typically last quite some time before recombining through less efficient means. Silicon is an indirect bandgap semiconductor, and hence is not generally useful for light-emitting diodes or laser diodes.
However, the indirect (non-radiative) recombination takes place at point defects or at grain boundaries (surface) in Si. If the excited electrons are prevented from reaching these recombination places, they have no choice but to fall back into the valence band by radiative recombination. This can be done by creating a dislocation loop in the silicon. At the edge of the loop, the planes above and beneath the "dislocation disk" are pulled apart, creating a negative pressure, which raises the energy of the conduction band substantially, with the result that the electrons cannot pass this edge. Provided that the area directly above the dislocation loop is defect-free (no non-radiative recombination possible), the electrons will fall back into the valence shell by radiative recombination and thus emitting light.
Direct semiconductor: GaAs
[edit | edit source]
In semiconductor physics, a direct bandgap means that the minimum of the conduction band lies directly above the maximum of the valence band in momentum space. In a direct bandgap semiconductor, electrons at the conduction-band minimum can combine directly with holes at the valence band maximum, while conserving momentum. The energy of the recombination across the bandgap will be emitted in the form of a photon of light. This is radiative recombination, also called spontaneous emission.
The prime example of a direct bandgap semiconductor is gallium arsenide—a material commonly used in laser diodes.
Ferroelectric: BaTiO3
[edit | edit source]Barium titanate is an oxide of barium and titanium with the chemical formula BaTiO3. It is a ferroelectric ceramic material, with a photorefractive effect and piezoelectric properties. It has five phases as a solid, listing from high temperature to low temperature: hexagonal, cubic, tetragonal, orthorhombic, and rhombohedral crystal structure. All of the structures exhibit the ferroelectric effect except cubic.
Superconductor: YBaCuO3, Nb-Ti
[edit | edit source]Superconductivity is a phenomenon occurring in certain materials at extremely low temperatures, characterized by exactly zero electrical resistance and the exclusion of the interior magnetic field (the Meissner effect).
The electrical resistivity of a metallic conductor decreases gradually as the temperature is lowered. However, in ordinary conductors such as copper and silver, impurities and other defects impose a lower limit. Even near absolute zero a real sample of copper shows a non-zero resistance. The resistance of a superconductor, on the other hand, drops abruptly to zero when the material is cooled below its "critical temperature". An electrical current flowing in a loop of superconducting wire can persist indefinitely with no power source. Like ferromagnetism and atomic spectral lines, superconductivity is a quantum mechanical phenomenon. It cannot be understood simply as the idealization of "perfect conductivity" in classical physics.
Superconductivity occurs in a wide variety of materials, including simple elements like tin and aluminium, various metallic alloys and some heavily-doped semiconductors. Superconductivity does not occur in noble metals like gold and silver, nor in most ferromagnetic metals.
In 1986 the discovery of a family of cuprate-perovskite ceramic materials known as high-temperature superconductors, with critical temperatures in excess of 90 kelvin, spurred renewed interest and research in superconductivity for several reasons. As a topic of pure research, these materials represented a new phenomenon not explained by the current theory. And, because the superconducting state persists up to more manageable temperatures, past the economically-important boiling point of liquid nitrogen, more commercial applications are feasible, especially if materials with even higher critical temperatures could be discovered.
Antiferromagnetic: Cr, Mn
[edit | edit source]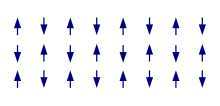
In materials that exhibit antiferromagnetism, the spins of electrons align in a regular pattern with neighboring spins pointing in opposite directions. This is a different manifestation of magnetism. Generally, antiferromagnetic materials exhibit antiferromagnetism at a low temperature, and become disordered above a certain temperature; the transition temperature is called the Néel temperature. Above the Néel temperature, the material is typically paramagnetic.
The antiferromagnetic behaviour at low temperature usually results in diamagnetic properties, but can sometimes display ferrimagnetic behaviour, which in many physically observable properties is more similar to ferromagnetic interactions.
The magnetic susceptibility of an antiferromagnetic material will appear to go through a maximum as the temperature is lowered; in contrast, that of a paramagnet will continually increase with decreasing temperature. However, more complicated behavior may result if the magnetic structure is more complicated.
Antiferromagnetic materials have low geometrical frustration, and a negative coupling between adjacent moments.
Antiferromagnetic materials are relatively uncommon. An example is the heavy-fermion superconductor URu2Si2. More everyday examples include metals such as chromium, alloys such as iron manganese (FeMn), and oxides such as nickel oxide (NiO). There are also numerous examples among high nuclearity metal clusters. Organic molecules can also exhibit antiferromagnetic coupling under rare circumstances, as seen in radicals such as 5-dehydro-m-xylylene.
Soft ferromagnetic (refrigerator doors): defect-free Fe-Ni (permalloy)
[edit | edit source]Permalloy is the term for a nickel iron magnetic alloy. Generically, it refers to an alloy with about 20% iron and 80% nickel content. Permalloy has a high magnetic permeability, low coercivity, near zero magnetostriction, and significant anisotropic magnetoresistance. The low magnetostriction is critical for industrial applications, where variable stresses in thin films would otherwise cause a ruinously large variation in magnetic properties. Permalloy's electrical resistivity generally varies within the range of 5% depending on the strength and the direction of an applied magnetic field.
After a prolonged search, Permalloy was discovered in 1914 by Gustav Elmen of Bell Laboratories, who found it had higher permeability than silicon steel. Later, in 1923, he found its permeability could be greatly enhanced by heat treatment. Other compositions of Permalloy are available, designated by a numerical prefix denoting the percentage of nickel in the alloy, for example 45 Permalloy containing 45% nickel, and 55% iron. Molybdenum Permalloy is an alloy of 81% nickel, 17% iron and 2% molybdenum. The latter was invented at Bell Labs in 1940. At the time, when used in long distance copper telephone lines, it allowed a tenfold increase in maximum line working speed.
Hard ferromagnetic (refrigerator magnets): defect-full BaFeOx, rare-earth compounds, Alnico
[edit | edit source]Alnico is an acronym referring to alloys which are composed primarily of aluminium (symbol Al), nickel (symbol Ni) and cobalt (symbol Co), hence al-ni-co, with the addition of iron, copper, and sometimes titanium, typically 8-12% Al, 15-26% Ni, 5-24% Co, up to 6% Cu, up to 1% Ti, rest is Fe. The primary use of alnico alloys is magnet applications.
Alnico alloys make strong permanent magnets, and can be magnetized to produce strong magnetic fields. Of the more commonly available magnets, only rare-earth magnets such as neodymium and samarium-cobalt are stronger. Alnico magnets produce magnetic field strength at their poles as high as 1500 gauss (0.15 tesla), or about 3000 times the strength of Earth's magnetic field. Some types of Alnico are isotropic and can be efficiently magnetized in any direction. Other types, such as Alnico 5 and Alnico 8, are anisotropic, with each having a preferred direction of magnetization, or orientation. Anisotropic alloys generally have greater magnetic capacity in a preferred orientation than isotropic types. Alnico remanence (Br) may exceed 12000 gauss (1.2T), its coercion force (Hc) can be up to 1000 oersted (80kA/m), its energy product ((B*H)max) can be up to 5.5M Gs*Oe (44 T*A/m) - this means Alnico can produce high magnetic flux in closed magnetic circuit, but has relatively small resistance against demagnetization.
Alnico is produced by casting or sintering processes. Anisotropic alnico magnets are oriented by heating above a critical temperature, and cooling in the presence of a magnetic field. Both isotropic and anisotropic Alnico require proper heat treatment to get its magnetic properties - without it its coercion is about 10 Oe - comparable to technical iron, which is a soft magnetic material. After the heat treatment alnico becomes kind of composite material, named "precipitation material" - it consist of rich-Co precipitate in rich-NiAl matrix. Alnico anisotropy is oriented if it is in external magnetic field during the precipitate particle nucleation, which occur when cooling from 900 to 800 C, near Curie point. Without external field there are local anisotropies of different orientations, due to spontaneous magnetization. The precipitate structure is a "barrier" against magnetization changes, as it prefers few magnetization states requiring high energy to get the material into any intermediate state. Also, small magnetic field change magnetization of matrix phase only, and it is reversible.
Alnico alloys have some of the highest Curie points of any magnetic material, around 800 °C. This property, as well as its brittleness and high melting point, is the result of the strong tendency toward order due to intermetallic bonding between aluminium and its other constituents. They are also one of the most stable magnets if they are handled properly.
Anisotropic ferromagnets: Fe (BCC, [100] easy direction), Ni (FCC, [111]), Co (HCP, [0001])
[edit | edit source]Diamagnetic: C2 (no net spin)
[edit | edit source]
Diamagnetism is a weak repulsion from a magnetic field. It is a form of magnetism that is only exhibited by a substance in the presence of an externally applied magnetic field. It results from changes in the orbital motion of electrons. Applying a magnetic field creates a magnetic force on a moving electron in the form of F = Qv × B. This force changes the centripetal force on the electron, causing it to either speed up or slow down in its orbital motion. This changed electron speed modifies the magnetic moment of the orbital in a direction opposing the external field.
Consider two electron orbitals; one rotating clockwise and the other counterclockwise. An external magnetic field into the page will make the centripetal force on an electron rotating clockwise increase, causing it to speed up. That same field would make the centripetal force on an electron rotating counterclockwise decrease, causing it to slow down. The orbiting electrons create magnetic fields themselves, and in both cases, the change in B due to the electron's change in velocity is in the opposite direction to the external B field. Since the material originally had no net magnetic field from its orbiting electrons (because their orbits were aligned in random directions), the result is that the induced B field opposes the applied B field, and these repel each other.
All materials show a diamagnetic response in an applied magnetic field. In fact, diamagnetism is a very general phenomenon, because all paired electrons, including the core electrons of an atom will always make a weak diamagnetic contribution to the material's response. However, for materials which show some other form of magnetism (such as ferromagnetism or paramagnetism), the diamagnetism is completely overpowered. Substances which only, or mostly, display diamagnetic behaviour are termed diamagnetic materials, or diamagnets. Materials that are said to be diamagnetic are those which are usually considered by non-physicists as "non magnetic", and include water, DNA, most organic compounds such as petroleum and some plastics, and many metals, particularly the heavy ones with many core electrons, such as mercury, gold and bismuth.
Paramagnetic: B2 (net spin)
[edit | edit source]
Paramagnetism is a form of magnetism which occurs only in the presence of an externally applied magnetic field. Paramagnetic materials are attracted to magnetic fields, hence have a relative magnetic permeability greater than one (or, equivalently, a positive magnetic susceptibility). The force of attraction generated by the applied field is linear in the field strength and rather weak. It typically requires a sensitive analytical balance to detect the effect. Unlike ferromagnets paramagnets do not retain any magnetization in the absence of an externally applied magnetic field, because thermal motion causes the spins to become randomly oriented without it. Thus the total magnetization will drop to zero when the applied field is removed. Even in the presence of the field there is only a small induced magnetization because only a small fraction of the spins will be orientated by the field. This fraction is proportional to the field strength and this explains the linear dependency. The attraction experienced by ferromagnets is non-linear and much stronger, so that it is easily observed on the door of one's fridge.
Magnetoresistance:
[edit | edit source]Magnetoresistance is the property of a material to change the value of its electrical resistance when an external magnetic field is applied to it. The effect was first discovered by William Thomson (more commonly known as Lord Kelvin) in 1856, but he was unable to lower the electrical resistance of anything by more than 5%. This effect was later called ordinary magnetoresistance (OMR). More recent researchers discovered materials showing giant magnetoresistance (GMR), colossal magnetoresistance (CMR) and magnetic tunnel effect (TMR).
High-K Dielectric: HfO2
[edit | edit source]
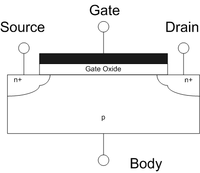
The term high-κ dielectric refers to a material with a high dielectric constant (κ) (as compared to silicon dioxide) used in semiconductor manufacturing processes which replaces the silicon dioxide gate dielectric. The implementation of high-κ gate dielectrics is one of several strategies developed to allow further miniaturization of microelectronic components, colloquially referred to as extending Moore's Law.
Silicon dioxide has been used as a gate oxide material for decades. As transistors have decreased in size, the thickness of the silicon dioxide gate dielectric has steadily decreased to increase the gate capacitance and thereby drive current and device performance. As the thickness scales below 2 nm, leakage currents due to tunneling increase drastically, leading to unwieldy power consumption and reduced device reliability. Replacing the silicon dioxide gate dielectric with a high-κ material allows increased gate capacitance without the concomitant leakage effects.
The gate oxide in a MOSFET can be modeled as a parallel plate capacitor. Ignoring quantum mechanical and depletion effects from the Si substrate and gate, the capacitance C of this parallel plate capacitor is given by
Where
- is the capacitor area
- is the relative dielectric constant of the material (3.9 for silicon dioxide)
- is the permittivity of free space
- is the thickness of the capacitor oxide insulator
Low-K dielectric:
[edit | edit source]In semiconductor manufacturing, a Low-κ dielectric is a material with a small dielectric constant relative to silicon dioxide. Although the proper symbol for the dielectric constant is the Greek letter κ (kappa), in conversation such materials are referred to as being "low-k" (low-kay) rather than "low-κ" (low-kappa). Low-κ dielectric material implementation is one of several strategies used to allow continued scaling of microelectronic devices, colloquially referred to as extending Moore's law. In digital circuits, insulating dielectrics separate the conducting parts (wire interconnects and transistors) from one another. As components have scaled and transistors have gotten closer and closer together, the insulating dielectrics have thinned to the point where charge build up and crosstalk adversely affect the performance of the device. Replacing the silicon dioxide with a low-κ dielectric of the same thickness reduces parasitic capacitance, enabling faster switching speeds and lower heat dissipation. The dielectric constant of SiO2, the insulating material used in silicon chips, is 3.9.
Polymer: Polyethylene
[edit | edit source]Miscible system:
[edit | edit source]Miscibility is a term in chemistry that refers to the property of liquids to mix in all proportions, forming a homogeneous solution. In principle, the term applies also to other phases (solids and gases), but the main focus is on the solubility of one liquid in another. For example, water and ethanol are miscible in all proportions.
Miscibility is partly a function of entropy, and so is seen more commonly in states of matter that are more entropic. Gases mix quite readily, but solids only rarely display miscibility. Two useful exceptions to this rule are solid solutions of copper with nickel (the cupronickel used in coins and specialty plumbing), and of silicon with germanium (used in electronics). Substances with extremely low configurational entropy, especially polymers, are unlikely to be miscible in one another even in the liquid state. Perdeuteropolyethylene is immiscible in polyethylene.
Immiscible system:
[edit | edit source]By contrast, substances are said to be immiscible if in any proportion, they do not form a homogeneous phase. For example, diethyl ether is fairly soluble in water, but these two solvents are not miscible since they are not soluble in all proportions.