Design for the Environment/Bus Fuel Alternatives

The Toronto Transit Commission (TTC) operates one of the most heavily used public transit networks in North America. In 2007, TTC had over 1500 public buses in use, with their total operating mileage exceeding 66 million miles[1]. Consequently, it has a high demand for a clean, efficient fuel source to power its bus fleet.
With predictions of a petroleum shortage in the near future, the TTC is looking for new, more environmentally friendly, ways to fulfill its energy needs. The following analysis will attempt to provide an overreaching comparison of petroleum based diesel, biodeisel and hydrogen fuel cells and will recommend one as a viable solution. Although the TTC’s current fleet consists of electric hybrids, diesel and biodiesel fueled buses the analysis assumes the selected alternative would be used exclusively.
Project Information
[edit | edit source]Group 6, Section 2,
Members:
Dmitry Lilko (Dimo)
Iman Datta (Imandatta)
Mariam Jawaid Syed (Mariam)
Pulkit Shah (aerodreamer)
Project Background
[edit | edit source]Diesel is a petroleum derived fuel. Its chemical structure is that of a hydrocarbon chain made up of 10-15 carbons, giving it an average energy density of 40.9 MJ/L. Diesel is combusted in an internal combustion engine, similar to gasoline, and operates on the Diesel cycle. In brief, air is compressed in the combustion chamber followed by fuel injection, at which point the rise in temperature causes the fuel to ignite. In this analysis, diesel with a concentration of 0.05%wt of sulfur is taken to be the baseline fuel source used by the TTC. The Orion Model V is assumed to be the TTC’s only type of bus, simplifying the analysis in the sense that no change in existing infrastructure is needed to accommodate this fuel type.
Biodieselis made by combining oil extracted from the canola seed and methanol. Canola oil and methanol are combined by a process called transesterification. This alternative proposes the use of biodiesel in its pure form (B100) without any addition of diesel fuel. An immediately apparent advantage of biodiesel is that it is a renewable fuel source due to the fact that canola seeds can be continuously produced. Furthermore, biodiesel can be used in the current TTC buses without any significant changes in the infrastructure. However, a cloud point depressor must be added to biodiesel during winter in order to prevent its crystallization [2].

Hydrogen Fuel Cells are a much more complicated method of energy generation, but also one that shows great promise with further development. Hydrogen is first separated from water, by a process known as electrolysis, using solar power. It is then fed into a fuel cell where it is oxidized producing a flow of electrons which is applied over a load, in our case, the bus motor. Infrastructure changes that go with this method include; equipment necessary for hydrogen synthesis, storing and transport of hydrogen and changes to existing bus motor. In this analysis, Dye-Sensitized Tandem Solar based cells will be used to synthesize hydrogen and Two Ballard 150 kW HD-6 Heavy duty Fuel Cell Modules will be used to generate the required power to operate a bus.
Highlights and Recommendations
[edit | edit source]Comparison of the SLCA scores show biodiesel to be the best option, with a score of 58, followed by diesel (46) and hydrogen (39.5). We find that the biodiesel is the cleanest fuel source in the premanufacture, manufacture, and delivery stages. The clear advantage is found in the first 2 stages, where we find the farming of the canola seeds and production methods are far more environmentally friendly than the mining and chemical processes associated with hydrogen production and crude oil drilling associated with diesel.
Comparison of EIOLCA analyses for each of the alternatives also showed the biodiesel to be the most environmentally friendly alternative. Separate analysis of relative Global Warming Potentials as well as the relative total emissions of CO, NOx, VOCs, SO2, and PM between each alternative clearly showed that the biodiesel causes the least amount of emissions and represents the lowest Global Warming Potential. This backs up our conclusion from the SLCA that this is they most environmentally viable alternative.
Based on a well-to-wheel comparison of the fuel sources, we also find biodiesel to be the best alternative. It is by far the most efficient fuel source when calculating from a premanufacture stage to use stage. It is almost 4 times more efficient than diesel and more than 50 times more efficient than hydrogen. So as an energy source, this is the most viable option.
Cost comparisons indicate that continuing with diesel would be the cheapest solution for the TTC, costing them about $97 million per year in fuel costs. Switching to biodiesel would increase this number by $10 million. The existing infrastructure of the TTC would not have to change as the biodiesel would be able to be used in existing buses and their engines. The cost of switching to a hydrogen-powered system would be exorbitant in terms of both annual fuel cost and the cost of overturning the fleet into one with fuel cell and hydrogen storage capabilities.
When comparing societal issues we find those involving the biodiesel to be the the most problematic while the hydrogen face the least amount of negative societal impacts. The dangerous impacts of the biodiesel production on food prices and supply seem to outweigh the impacts of the diesel's combustion impact on the environment and pressurized hydrogen tanks' threat to passenger safety.
The relative scores of each alternative according to each analysis (SLCA, EIOLCA, Cost, Functional, and Societal) were scored against each other using a specific rubric for each type of analysis. The direct scores of the SLCA were used in the calculation along with relative Greenhouse Gas and various particle emissions from the EIOLCA. The relative costs were scored against each other and a relative well-to-wheel comparison was used for the functional analysis comparison. For the societal analysis comparison, a subjective measure was used to rate the societal impacts associated with the alternatives against each other. The result is shown in the table below (10 being the best and 0 being the worst):
This table scores a 34 for biodiesel, followed by diesel (22) and hydrogen (16). This clearly shows the superiority of the biodiesel and is the reason we recommend the biodiesel as a new fuel source for the Toronto Transit Commission.
Functional Analysis
[edit | edit source]TTC’s fleet currently consists of Orion Model V buses which are powered by Cummins ISM M11 engines [3]. Hydrogen buses currently in operation in 9 European countries under the CUTE project are being powered by Ballard P5 Heavy Duty Fuel Cells generating 250kW. The new proposed Mercedes Citaro Buses will use 2 new Ballard HD-6 150kW Heavy duty fuel cells instead.
Efficiency
[edit | edit source]Diesel as a fuel has a well to wheel energy efficiency of 83%[4]. Biodiesel B100 has a well to wheel efficiency of 320%. The well to wheel efficiency for hydrogen is relatively very low. The Dye-Sensitized Tandem Cell producing hydrogen has an efficiency of only 10%. The fuel cell has an efficiency of about 40-60% resulting in an upper limit efficiency of 6%. Hydrogen storage which is quite energy intensive has not been factored into this calculation.
Performance
[edit | edit source]The Orion Model V diesel powered buses demonstrate a mileage of 3.4 mpg. When biodiesel is used instead of diesel in the same engine, the mileage drops by 10%, resulting in a mileage of 3 mpg. Hydrogen has a mileage of 3.52 miles/kg H2 which is much higher than diesel’s 1.25 miles/kg diesel [5]. The milage has been calculated based on the range of the hydrogen bus. However, hydrogen is much more expensive to purchase as compared to diesel. Hydrogen fuel cell powered Citaro buses have a top speed of 80 kmph [6].
Range
[edit | edit source]Based on calculations with a 150 Gallons onboard diesel fuel tank, the current TTC Orion buses run 510 miles before running out of fuel. When biodiesel is used in the same engine, the range reduces to 450miles. Comparatively hydrogen has a much smaller range of 155 miles [7] on a full tank. The refueling time for hydrogen is approximately 15 minutes.
Streamlined Lifecycle Analysis (SLCA)
[edit | edit source]Premanufacturing
[edit | edit source]Materials Choice
[edit | edit source]For this square diesel receives a low score due to an input material of crude oil, which is a virgin, nonrenewable resource [8]. Biodiesel received an average score due to its different input materials in this stage. It is produced from natural gas (nonrenewable) and canola (renewable) which can be continuously planted without additional fertilizers [9]. The production of hydrogen requires the manufacture of the Tandem Cell at this stage, which requires platinum and ruthenium extraction, both of which are rare metals and cause hydrogen to get a low score [10].
Energy Use
[edit | edit source]Immense pressure and heat levels must be used in the drilling of crude oil, especially in the tar fields of northern Alberta, which supplies the most of this material to Ontario [11]. This results in the low score for diesel. The average score for biodiesel stems from the fact that energy use at this stage is dominated by truck transportation between the canola fields in rural areas to the TTC. Energy is also consumed by transportation of methanol. Hydrogen receives a low score due to the energy consumption attributed to transportation of metals from mines, chemical synthesis for dyes that make up the Tandem Cell, and purification process performed after mining [12].
Solid Residues
[edit | edit source]Both diesel and biodiesel receive high scores in this category. Minimal solid residues in the form of non-contaminated earth an rock are produced in crude oil drilling for diesel production and no solid residues are produced from biodiesel production. The high material diversity of the Tandem cell consisting of metals with limited recycling capability yield the low score for hydrogen [13] .
Liquid Residues
[edit | edit source]Liquid residues from crude oil drilling for diesel include oil-polluted water, low-weight hydrocarbons, and inorganic salts as well as other materials [1], which leads to its average score. The production of biodiesel involves limited residues of pesticides and high amounts of water, which give it a slightly higher score. Iron and platinum extraction for the Tandem Cell cause a low score for hydrogen as mining of these metals cause acid residues [8].
Gaseous Residues
[edit | edit source]Flaring out gaseous wastes during oil extraction is a major contributor to gas residue for diesel production, although this has decreased in recent years [4]. leading to its average score. Biodiesel receives a similar score due to pollutants released during canola cultivation as well as CO and CO2 released during methanol extraction. Hydrogen receives a low score due to the fact that the metals that produce liquid residues also produce gaseous residues [8].
Manufacturing
[edit | edit source]Materials Choice
[edit | edit source]Diesel refining is not an environmentally friendly procedure, but is done alongside other useful hydrocarbons. Biodiesel gets a slightly better score because the materials do not undergo any highly energy intensive chemical processes in its formation. Hydrogen gets an average score between the high score of light and water used in the Tandem Cells [14] and low score for metals, nafion membrane, and composite materials used in fuel cells.
Energy Use
[edit | edit source]Fractional distillation of diesel from petroleum is a very energy intensive process [15], leading to diesel's low score here. Biodiesel's average score stems from canola seed crushing, heating, and transesterification. Hydrogen receives an average score for sunlight input into the Tandem Cell and the energy intensive processes of nafion production [16] and metal production.
Solid Residues
[edit | edit source]There is very little solid residue in diesel manufacture, leading to its high score. Biodiesel production leads to some soap residue, which gives it a slightly lower score [17]. Hydrogen receives an average score due an average of a high score for input light into the Tandem Cell and low score for materials used in the fuel cell, which are similar to those of Tandem Cell [7].
Liquid Residues
[edit | edit source]All alternatives get average scores in this section, with biodiesel slightly better than the rest. Here water needed to wash excess soap is the main residue. The hydrogen gets an average between the minimum residue of the Tandem Cell and the high residues of the fuel cell. The diesel has many different residues.
Gaseous Residues
[edit | edit source]Fractional distillation causes sulfur oxide, NOx, VOC, particulate, and GHG residue [15], leading to its low score. The hydrogen gets an average score for the same reasons it does for solid and liquid residues. Biodiesel gets a score of 2 for gases released during transesterification.
Product Packaging and Transport
[edit | edit source]Materials Choice
[edit | edit source]Diesel receives the highest score for this category due to the uniform steel composition of pipelines which transport the fuel source from Alberta. Biodiesel does worse because it cannot be transported in pipelines due to its tendency to freeze in them [18]. Hydrogen does the worst due to need for composite and insulating materials in both pipelines and storage tanks [7], [19], [20].
Energy Use
[edit | edit source]Biodiesel receives the highest score for this category due to the energy usage from trucks. Diesel and hydrogen get low scores due to the pressures needed to transport the fuel sources (with hydrogen requiring the higher pressures in pipelines and storage tanks) [21], [22].
Solid, Liquid, and Gas Residues
[edit | edit source]Both diesel and biodiesel score highly in solid and liquid residues because they are both a minimum. For hydrogen, solid residues are very low due to high material diversity in composites and polymers. Liquid residues gets a higher score, with only steel production causing acid releases. Finally, gas emissions from truck transportation of diesel and biodiesel are the same and are scored higher than the gas residue associated with hydrogen loss from high pressure in pipelines and fuel tanks.
Product Use
[edit | edit source]Material Choice
[edit | edit source]In this category diesel scores the worst as it is the the most unclean fuel at this stage since it causes the emissions of NOx, soot, sulfates, silicates, and other various ash particulates. Biodiesel does better because it provides extra lubrication to the engine, providing better combustion and increasing the lifespan of the engine, despite requiring some additives. Hydrogen does the best here as the only emission from the fuel cell is water [23].
Energy Use
[edit | edit source]There is no extra energy loss associated with the use phase of both the diesel and hydrogen, which leads to their high scores. Biodiesel does slightly worse due to additives needed in the combustion process [24].
Solid, Liquid, and Gaseous Residues
[edit | edit source]Hydrogen is clearly the best here as the only residue is water vapour [23]. In fact, there are no solid or liquid emissions for any of the alternatives. However, there are various gaseous emissions originating from both diesel and biodiesel combustion, which lead to their lower score.
Recycling and Disposal
[edit | edit source]This stage is not scored for any of the alternatives as they are all consumed during the use phase, leaving none of the product available for recycle.
Economic Input-Output Lifecyle Analysis (EIOLCA)
[edit | edit source]EIO-LCA analysis was performed using the GHGenius and US Department of Commerce 1997 Industry Benchmark models. The GHGenius models considers the use phase and the implications of the fuel on the environment while the latter does not. Hydrogen as a fuel would trump other alternative fuels if analyzed from a Pump to Wheel aspect. In reality, TTC would need to lay down an extensive infrastructure before a change can be made. The infrastructure which includes the production of Dye-Sensitized Solar Cells, fuel cells and storage tanks for the hydrogen fuel cell contributes towards increasing the final green house gas and other toxic gas emissions for hydrogen fuel. The cost inputs for the EIO-LCA analysis were calculated over 10 year period for the entire TTC fleet and converted to 1997 dollars using an inflation rate of 4%.
Global Warming Potential
[edit | edit source]The use phase of hydrogen does not release any green house gas emissions as the byproduct of the chemical reaction in the fuel cell is water vapor. The ‘Power Generation and Supply’ sector is the main contributor towards green house gas emissions which is no surprise as rare metals such as titanium and platinum are being used in the manufacturing of the Tandem cell and hydrogen fuel cell. The nano-crystalline thin film coating of Tungsten on the glass of the first part of the tandem cell and the Titanium dioxide film on the glass of the second half of the tandem cell consumes considerable amounts of energy [25] It was discovered that “use” phase of the biodiesel releases the most amount of emissions because during the use stage, TTC buses utilize the energy stored in biodiesel via combustion in order to operate. Combustion releases a significant amount of gases in the air. During the operation of the buses, 220,298 MTCO2 emissions released for each kilometre travelled by the bus. However, the canola crops absorb an equivalent of 214,682 MTCO2 emissions during photosynthesis, thus reducing the total amount of emissions released in the air. According to the analysis, hydrogen production actually releases more green house gases. Amongst the three fuels, diesel releases the most amount of methane. This is because when the wells are bored during extraction, natural gas is released as it is lighter than diesel and floats above it. Since diesel also releases a lot of carbon dioxide as a result of combustion, it has the highest global warming potential among the three fuels.
Conventional Air Pollutants
[edit | edit source]The premanufacturing and extraction stage contributes towards the carbon monoxide emissions. However the majority of carbon monoxide emissions comes from unburnt hydrocarbons as a result of incomplete combustion. The most significant source of air pollutant emissions for biodiesel is NOx, with total emissions of 1,309.5 metric tonnes. This increase has been hypothesized to be due to the higher flame point of biodiesel, which in turn increases the temperature of biodiesel during combustion, thus releasing more NOx. A heavy amount of carbon monoxide is released into the atmosphere due to the ‘Carbon and Graphite products manufacturing’ during the production of the fuel cell. Graphite which is the raw form of carbon is weak and brittle. Heat treatment to 2200оC is used to strengthen it. This heating stage results in high carbon monoxide emissions.
Cost Analysis
[edit | edit source]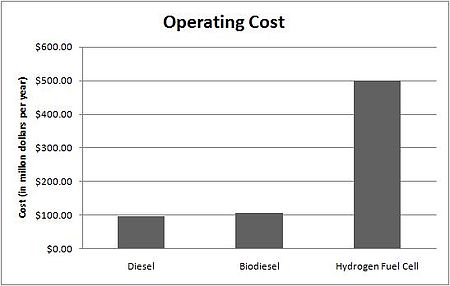
TTC buses travel an average of 108 million kilometres per year Cite error: Closing </ref>
missing for <ref>
tag. Thus, in 2008 dollars, TTC will disburse approximately $97.4 million on the utilization of diesel.
Based on the same mileage data, the utilization of biodiesel will cost the TTC approximately $106.7 million per year [1], [26]. However, due to biodiesel's low cloud point, additives must be added to it. The cost of utilizing the additives, in Toronto's weather conditions, is $ 123,241.98 per year [24]. Consequently, the total cost of employing biodiesel would increase to a total of $106.8 million per year. Since the utilization of the biodiesel requires no changes in TTC buses' existing infrastructure, there are no costs associated with replacement of diesel with biodiesel.
Similarly, the use of hydrogen fuel cells in TTC buses would cost TTC $500 million annually [7]. This cost includes the cost of hydrogen generation, transportation, and storage. The high cost related to the use of hydrogen fuel cells is due to hydrogen generation costs. Currently, the cost of hydrogen production using solar-based electrolysis is $28.19 per kilogram of hydrogen produced [27]. Moreover, the utilization of hydrogen fuel cells would require changes in the buses' infrastructure. The total cost of replacing TTC's entire fleet of buses would be $1.55 billion, excluding the salvage value of the existing buses.
This comparison illustrates that diesel is the most economic alternative.
Societal Analysis
[edit | edit source]The burning of diesel produces soot particles. Soot particles below a critical diameter may be inhaled and settle in the lungs [28]. Studies have shown that people living in heavily polluted areas raise their risk of dying from lung cancer up to 12%. In addition, almost as much as 11% of infant deaths in the U.S are caused by soot particles [29] . Another potential impact may arise from geopolitical situations. Although Canada does not depend on crude oil import as much as the U.S, conflicts in the Middle East may lead to the rising price of petroleum. Since there would be higher foreign demand for Canadian petroleum, provincial refineries would be forced to compete for it at global prices [30].
The use of feedstock in biodiesel production may have adverse effects on environmental and economic areas. In order to obtain high quality biodiesel, the most nutritious canola seeds need to be used. This places an increased burden on farmers to produce more quality seeds for human consumption, or may simply lower the overall quality of available stock. This competition may cause inflation in the overall prices of food, which could potentially lead to famine in developing nations. Moreover, continuous cultivation of canola seeds would cause a decline in the soil quality. This would mean that fewer products can be produced and additional fertilizers would be required for the cultivation of feedstock. These additional fertilizers would increase the toxic emissions in the air and increase water pollution.
The proposed hydrogen fuel cell buses use electric motors as the mode of powering the wheels, thus running quieter than combustion engines and reducing noise pollution. In addition, fuel cells would serve to reduce dependence on fossil fuels and frustrating crude oil price fluctuations. Since power generation starts and ends with water, for hydrogen synthesis and as a waste product of the fuel cell, this technology is very close to being self-sufficient. Another aspect is the safety issue with hydrogen storage tanks. It may take a very strong marketing campaign to convince the general public to accept the hazards and dangers of driving around with pressurized storage tanks on their buses.
See also
[edit | edit source]To read other projects, please go to Design for the Environment class page.
References
[edit | edit source]- ↑ 1.0 1.1 1.2 "Toronto Transit Commission," Available at: http://web.archive.org/web/20080411084936/http://www.toronto.ca/ttc/pdf/operatingstatistics2007.pdf [Accessed March 19, 2008]
- ↑ International Energy Agency, Biofuels, 1 ed. , Paris: OECD, 1994
- ↑ "Specifications Orion V High Floor," Available at: http://www.orionbus.com/orion/0-867-584068-1-10754-1-0-0-0-0-1-10595-584008-0-0-0-0-0-0-0.html [Accessed March 1, 2008]
- ↑ 4.0 4.1 U.S Department of Energy & Department of Agriculture. “Life Cycle Inventory of Biodiesel and Petroleum diesel for Use in a Public Bus (1998)” [Accessed 31 Mar, 2008] <http://www.nrel.gov/docs/legosti/fy98/24089.pdf>
- ↑ "Green Car Congress," Available at: http://www1.eere.energy.gov/vehiclesandfuels/pdfs/basics/jtb_biodiesel.pdf [Accessed February 24, 2008]
- ↑ "Green Car Congress," Available at: http://www.greencarcongress.com/2006/03/european_fuelce.html [Accessed March 1, 2008]
- ↑ 7.0 7.1 7.2 7.3 D. Gielen, G. Simbolotti., Prospects for Hydrogen and Fuel Cells, Paris: International Energy Agency, 2005.
- ↑ 8.0 8.1 8.2 T. E. Graedel, Streamlined Life-Cycle Assessment, Prentice-Hall, 1998] Cite error: Invalid
<ref>
tag; name "SLCA" defined multiple times with different content - ↑ Hill et al., “Biodiesel: The Sustainability Dimensions” [Online Document] Available at http://attra.ncat.org/attra-pub/biodiesel_sustainable.html
- ↑ M Murayama, T Mori, "Dye-Sensitized solar cell using novel tandem cell structure," Journal of Physics D: Applied Physics, vol. 40, no. 6, pp. 1664-1668, 2 March 2007.
- ↑ Natural Environment Research Council, British Geological Survey. “Onshore Oil and Gas (November 2006)” [Accessed 28 Mar, 2008] <http://www.mineralsuk.com/britmin/mpfoil_gas.pdf>
- ↑ Emsley, Nature's Building Blocks: An A-Z Guide to the Elements, Oxford: Oxford University Press, 2001.
- ↑ M Murayama, T Mori, " Dye-Sensitized solar cell using novel tandem cell structure," Journal of Physics D: Applied Physics, vol. 40, no. 6, pp. 1664-1668, 2 March 2007
- ↑ “About Hydrogen Solar Tandem Cells”, The Energy Blog. [Online Document], (8 Oct 2005). [Accessed 15 Feb 2008]. Available at HTTP: http://thefraserdomain.typepad.com/energy/2005/10/hydrogen_solar_.html
- ↑ 15.0 15.1 About.com: Inventors, “Rudolf Diesel (1815- 1913)”. Available from: http://inventors.about.com/library/inventors/bldiesel.htm [Accessed 20 Mar, 2008]
- ↑ USA. A. Burnham, M. Wang, Y. Wu. Argonne National Laboratory. Development and Applications of GREET 2.7 – The Transportation Vehicle-Cycle Model. Argonne, Ill: U.S. Department of Energy - Argonne National Laboratory, 2006
- ↑ Hobbs, “Biodiesel from Canola Oil” [Online Document] Available at: http://web.archive.org/web/20050513161838/http://www.ballarat.edu.au/projects/ensus/case_studies/biodiesel/pdfs/biodiesel_printable.pdf
- ↑ Hill et al., “Biodiesel: The Sustainability Dimensions” [Online Document] Available at http://attra.ncat.org/attra-pub/biodiesel_sustainable.html
- ↑ U.S. Department of Energy. “Hydrogen Storage.” U.S. Department of Energy: Energy Efficiency and Renewable Energy, Hydrogen, Fuel Cells & Infrastructure Technologies Program. [Online Document]. (5 Mar 2008) [Accessed 10 Mar 2008]. Available HTTP: http://www1.eere.energy.gov/hydrogenandfuelcells/storage/hydrogen_storage.html
- ↑ K. Newell, "Low Cost, High Efficiency, High Pressure Hydrogen Storage," in DOE Hydrogen, Fuel Cells & Infrastructure Technologies Program Review, Quantam Technologies Inc, Irvine, California, May 2004.
- ↑ U.S. Department of Energy. “Hydrogen Storage.” U.S. Department of Energy: Energy Efficiency and Renewable Energy, Hydrogen, Fuel Cells & Infrastructure Technologies Program. [Online Document]. (5 Mar 2008) [Accessed 10 Mar 2008]. Available HTTP: http://www1.eere.energy.gov/hydrogenandfuelcells/storage/hydrogen_storage.html
- ↑ Enbridge Inc, 2007 Corporate Social Responsibility Report. Available from: http://www.enbridge.com/csr2007/environmental-performance/energy-use/ [Accessed 19 Mar, 2008],
- ↑ 23.0 23.1 Ballard. "Bus Market: Features and Benefits," [Online Document], (2008), [Accessed 8 Mar 2008]. Available HTTP: http://www.ballard.com/Bus_Fuel_Cells/Features_and_Benefits.htm
- ↑ 24.0 24.1 World Watch Institute, Biofuels for Transport, London: Earthscan, 2007
- ↑ “About Hydrogen Solar Tandem Cells”, The Energy Blog. [Online Document], (8 Oct 2005). [Accessed 15 Feb 2008]. Available at HTTP: http://thefraserdomain.typepad.com/energy/2005/10/hydrogen_solar_.html
- ↑ "TTC Fleet Roster," Available at: http://stw.ryerson.ca/~m4yip/transit/ttc/ [Accessed March 1, 2008]
- ↑ The Hydrogen Economy : Opportunities, Costs, Barriers, and R&D Needs. Ed. National Research Council (U.S.)., National Academy of Engineering., and National Academy of Sciences (U.S.). Washington, D.C.: National Academies Press, 2004.
- ↑ Health Canada, “Diesel Assessment” Date Modified: 2005-12-09 Available from: http://www.hc-sc.gc.ca/ewh-semt/air/out-ext/effe/diesel/assess_index_e.html [Accessed 25 Mar, 2008]
- ↑ University of Minnisota, Environmental & Occupational Health, “Health Effects of Diesel Exhaust” Available from: http://enhs.umn.edu/hazards/hazardssite/dieselexhaust/dieselhealtheffects.html [Accessed 28 Mar, 2008]
- ↑ Centre for Energy, “Canada’s Energy trade”. Available from: http://www.centreforenergy.com/silos/EnergyTrade/CanadaEnergyTrade.asp?page=2 [Accessed 23 Mar, 2008]